Disorders of Smell and Taste: Introduction
The sensations of smell (olfaction) and taste (gustation) are suitably considered together. Physiologically, these modalities share the singular attribute of responding primarily to chemical stimuli; i.e., the end organs that mediate olfaction and gustation are chemoreceptors. Also, taste and smell are interdependent clinically, as the appreciation of the flavor of food and drink depends to a large extent on their aroma, and an abnormality of one of these senses is frequently misinterpreted as an abnormality of the other. In comparison to sight and hearing, taste and smell play a less critical role in the life of the individual. However, chemical stimuli in communication between humans are probably very important for some functions that have not been fully explored. Pheromones (pherein, “to carry”; hormon, “exciting”), that is, odorants exuded from the body as well as perfumes, play a part in sexual attraction; noxious body odors repel. In certain vertebrates the olfactory system is remarkably well developed, rivaling the sensitivity of the visual system, but even humans, in whom the sense of smell is relatively weak, have the capacity to discriminate between as many as 10,000 different odorants (Reed).
Disorders of taste and smell can be persistently unpleasant, but only rarely is the loss of either of these modalities a serious handicap. Nevertheless, as all foods and inhalants pass through the mouth and nose, these two senses serve to detect noxious odors (e.g., smoke) and to avoid tainted food and potential poisons. The loss of these senses could then have serious consequences. Also, because a loss of taste and smell may signify a number of intracranial, neurodegenerative, and systemic disorders, they assume clinical importance.
Olfactory Sense
Nerve fibers subserving the sense of smell have their cells of origin in the mucous membrane of the upper and posterior parts of the nasal cavity (superior turbinates and nasal septum). The entire olfactory mucosa covers an area of about 2.5 cm2 and contains three cell types: the olfactory or receptor cells, which number between 6 and 10 million in each nasal cavity; sustentacular or supporting cells, which maintain the electrolyte (particularly K) levels in the extracellular milieu; and basal cells, which are stem cells and the source of both the olfactory and sustentacular cells during regeneration. The olfactory cells are actually bipolar neurons. Each of these cells has a peripheral process (the olfactory rod) from which project 10 to 30 fine hairs, or cilia. These hair-like processes, which lack motility, are the sites of olfactory receptors.
The central processes of these cells, or olfactory fila, are very fine (0.2 mm in diameter) unmyelinated fibers that converge to form small fascicles enwrapped by Schwann cells that pass through openings in the cribriform plate of the ethmoid bone into the olfactory bulb (Fig. 12-1). Collectively, the central processes of the olfactory receptor cells constitute the first cranial (olfactory) nerve. Notably, this is the only site in the body where neurons are in direct contact with the external environment. The epithelial surface is covered by a layer of mucus, which is secreted by tubuloalveolar cells (Bowman glands) and within which there are immunoglobulins A and M, lactoferrin, and lysoenzyme as well as odorant-binding proteins. These molecules are thought to prevent the intracranial entry of pathogens via the olfactory pathway (Kimmelman).
Figure 12-1.
Diagram illustrating the relationships between the olfactory receptors in the nasal mucosa and neurons in the olfactory bulb and tract. Cells of the anterior olfactory nucleus are found in scattered groups caudal to the olfactory bulb. Cells of the anterior olfactory nucleus make immediate connections with the olfactory tract. They project centrally via the medial olfactory stria and to contralateral olfactory structures via the anterior commissure. Inset: diagram of the olfactory structures on the inferior surface of the brain (see text for details).
In the olfactory bulb, the receptor-cell axons synapse with granule cells and mitral cells (so-called because they are triangular, like a bishop’s miter), the dendrites of which form brush-like terminals or olfactory glomeruli (Fig. 12-1). Smaller “tufted” cells in the olfactory bulb also contribute dendrites to the glomerulus. Approximately 15,000 olfactory-cell axons converge on a single glomerulus. This high degree of convergence is thought to account for an integration of afferent information. The mitral and tufted cells are excitatory; the granule cells—along with centrifugal fibers from the olfactory nuclei, locus ceruleus, and piriform cortex—inhibit mitral cell activity. Presumably, interaction between these excitatory and inhibitory neurons provides the basis for the special physiologic aspects of olfaction.
The axons of the mitral and tufted cells form the olfactory tract, which courses along the olfactory groove of the cribriform plate to the cerebrum. Lying caudal to the olfactory bulbs are groups of cells that constitute the anterior olfactory nucleus (Fig. 12-1). Dendrites of these cells synapse with fibers of the olfactory tract, while their axons project to the olfactory nucleus and bulb of the opposite side; these neurons are thought to function as a reinforcing mechanism for olfactory impulses.
Posteriorly, the olfactory tract divides into medial and lateral olfactory striae. The medial stria contains fibers from the anterior olfactory nucleus; these pass to the opposite side via the anterior commissure. Fibers in the lateral stria originate in the olfactory bulb, give off collaterals to the anterior perforated substance, and terminate in the medial and cortical nuclei of the amygdaloid complex and the prepiriform area (also referred to as the lateral olfactory gyrus). The latter represents the primary olfactory cortex, which in humans occupies a restricted area on the anterior end of the parahippocampal gyrus and uncus (area 34 of Brodmann; see Figs. 22-1 and 22-2). Thus olfactory impulses reach the cerebral cortex without a relay through the thalamus; in this respect also, olfaction is unique among sensory systems. From the prepiriform cortex, fibers project to the neighboring entorhinal cortex (area 28 of Brodmann) and the medial dorsal nucleus of the thalamus; the amygdaloid nuclei connect with the hypothalamus and septal nuclei. The role of these latter structures in olfaction is not well understood, but presumably they subserve reflexes related to eating and sexual function. As with all sensory systems, feedback regulation occurs at every point in the afferent olfactory pathway.
In quiet breathing, little of the air entering the nostril reaches the olfactory mucosa; sniffing carries the air into the olfactory crypt, which contains the olfactory receptors. To be perceived as an odor, an inhaled substance must be volatile—i.e., spread in the air as very small particles—and soluble in water. Molecules provoking the same odor seem to be related by their shape than by their chemical quality. When a jet of scented vapor is directed to the sensory epithelium, as by sniffing, a slow negative potential shift called the electroolfactogram (EOG) can be recorded from an electrode placed on the mucosa. The conductance changes that underlie this receptor potential are induced by molecules of odorous material dissolved in the mucus overlying the receptor.
The transduction of odorant stimuli to electrical signals is mediated in part by a guanosine triphosphate (GTP)-dependent adenylyl cyclase (“G protein”). Like other cyclic adenosine monophosphate (AMP) pathways, this one utilizes the same intracellular second messenger, which opens a voltage gated calcium channel in the receptor. There follow conformational changes in transmembrane receptor proteins and a series of intracellular biochemical events that generate axon potentials.
Intensity of olfactory sensation is determined by the frequency of firing of afferent neurons. The quality of the odor is thought to be provided by “cross-fiber” activation and integration, as described earlier, because the individual receptor cells are responsive to a wide variety of odorants and exhibit different types of responses to stimulants—excitatory, inhibitory, and on–off responses have been obtained. The olfactory potential can be eliminated by destroying the olfactory receptor surface or the olfactory filaments. The loss of EOG occurs 8 to 16 days after severance of the nerve; the receptor cells disappear, but the sustentacular (Sertoli) cells are not altered. As a result of division of the basal cells of the olfactory epithelium, the olfactory receptor cells are constantly dying and being replaced by new ones. In this respect, the chemoreceptors, both for smell and for taste, constitute one of the few examples of neuronal regeneration in humans.
The trigeminal system also participates in chemesthesis through undifferentiated receptors in the nasal mucosa. These receptors have little discriminatory ability but a great sensitivity to irritant stimuli. The trigeminal afferents also release neuropeptides that result in hypersecretion of mucus, local edema, and sneezing. Finally, stimulation of the olfactory pathway at cortical sites of the temporal lobe may also induce olfactory experiences.
The olfactory system adapts quickly to a sensory stimulus, and for sensation to be sustained, there must be repeated stimulation. The olfactory sense differs from other senses in yet another way. It is common experience that an aroma can restore long-forgotten memories of complex experiences. That olfactory and emotional stimuli are strongly linked is not surprising in view of their common roots in the limbic system. Yet, paradoxically, the ability to recall an odor is negligible in comparison with the ability to recall sounds and sights. As Vladimir Nabokov has remarked, “Memory can restore to life everything except smells.” It is also interesting that dreams do not embody olfactory experiences.
The remarkable evolutionary role of olfactory receptors can be appreciated by the fact that about 2 percent of the human genome exists to express unique odorant receptors (over 500 distinct genes). The wide diversity of these transmembrane proteins permits subtle differentiation of thousands of different odorant molecules, as delineated by Young and Trask and the genetic basis for which Buck and Axel were awarded a Nobel Prize.
This specificity for molecules is encoded neuroanatomically. Different odorant molecules activate specific olfactory receptors. Each olfactory neuron expresses only one allele of one receptor gene. Moreover, each olfactory glomerulus receives inputs from neurons expressing only one type of odorant receptor. In this way, each of the glomeruli is attuned to a distinct type of odorant stimulus. Presumably, this encoding is preserved in the olfactory cortex.
Something is to be learned from a second, distinct olfactory system in many animals (the vomeronasal olfactory system or organ of Jacobson), in which the repertoire of olfactory receptors is much more limited than in their main olfactory system. This functionally and anatomically distinct olfactory tissue is attuned to pheromones and thereby importantly influences menstrual, reproductive, ingestive, and defensive behavior (see review of Wysocki and Meredith). The vomeronasal receptors employ different signaling mechanisms than other olfactory receptors and project to the hypothalamus and amygdala via a distinct accessory olfactory bulb.
Disturbances of olfaction may be subdivided into four groups, as follows:
Quantitative abnormalities: loss or reduction of the sense of smell (anosmia, hyposmia) or, rarely, increased olfactory acuity (hyperosmia)
Qualitative abnormalities: distortions or illusions of smell (dysosmia or parosmia)
Olfactory hallucinations and delusions caused by temporal lobe disorders or psychiatric disease
Higher-order loss of olfactory discrimination (olfactory agnosia)
This is the most frequent clinical abnormality and, if unilateral, usually is not recognized by the patient. Unilateral anosmia can sometimes be demonstrated in the hysterical patient on the side of anesthesia, blindness, or deafness. Bilateral anosmia, on the other hand, is a common complaint, and the patient is usually convinced that the sense of taste has been lost as well (ageusia). This calls attention to the fact that taste depends largely on the volatile particles in foods and beverages, which reach the olfactory receptors through the nasopharynx, and that the perception of flavor is a combination of smell, taste, and tactile sensation. This can be proved by demonstrating that patients with anosmia but without a complaint of ageusia are able to distinguish the elementary taste sensations on the tongue (sweet, sour, bitter, and salty). The olfactory defect can be verified readily enough by presenting a series of nonirritating olfactory stimuli (vanilla, peanut butter, coffee, tobacco) and asking the patient to sniff once and identify them. If the odors can be detected and described, even if they cannot be named, it may be assumed that the olfactory nerves are relatively intact (humans can distinguish many more odors than they can identify by name). If they cannot be detected, there is an olfactory defect. Ammonia and similar pungent substances are unsuitable stimuli because they do not test the sense of smell but have a primary irritating effect on the mucosal-free nerve endings of the trigeminal nerves.
Nasal |
Smoking |
Chronic rhinitis (allergic, atrophic, cocaine, infectious—herpes, influenza) |
Overuse of nasal vasoconstrictors |
Olfactory epithelium |
Head injury with tearing of olfactory filaments |
Cranial surgery |
Subarachnoid hemorrhage, meningitis |
Toxic (organic solvents, certain antibiotics—aminoglycosides, tetracyclines, corticosteroids, methotrexate, opiates, L-dopa) |
Metabolic (thiamine and B12 deficiency, adrenal and thyroid deficiency, cirrhosis, renal failure, menses) |
Wegener granulomatosis |
Compressive and infiltrative lesions (craniopharyngioma, meningioma, aneurysm, meningoencephalocele) |
Central |
Degenerative diseases (Parkinson, Alzheimer, Huntington) |
Temporal lobe epilepsy |
Malingering and hysteria |
The value of testing smell in one nostril at a time has been questioned, for example by Welge-Luessen and colleagues, who studied olfactory groove meningiomas. They found, contrary to expectations, that this test was not sensitive to the presence of a unilateral lesion, ostensibly because of mixing of air in the nasopharynx. Nonetheless, other experience suggests that rapidly sniffing through one nostril does briefly allow segregation of each side of the nasal cavities and can detect unilateral lesions.
A more elaborate scratch-and-sniff test has been developed and standardized by Doty and colleagues (University of Pennsylvania Smell Identification Test). In this test the patient attempts to identify 40 microencapsulated odorants, and olfactory performance is compared with that of age- and sex-matched normal individuals. Unique features of this test are a means for detecting malingering and amenability to self-administration. Air-dilution olfactory detection is an even more refined way of determining thresholds of sensation and of demonstrating normal olfactory perception in the absence of odor identification. The use of olfactory evoked potentials is being investigated in some electrophysiology laboratories, but their reliability is uncertain. These last two refined techniques are essentially research tools and are not used in neurologic practice.
The loss of smell usually falls into one of three categories: nasal (in which odorants do not reach the olfactory receptors), olfactory neuroepithelial (caused by destruction of receptors or their axon filaments), and central (olfactory pathway lesions).
Viewed from another perspective, in an analysis of 4,000 cases of anosmia from specialized clinics, Hendriks
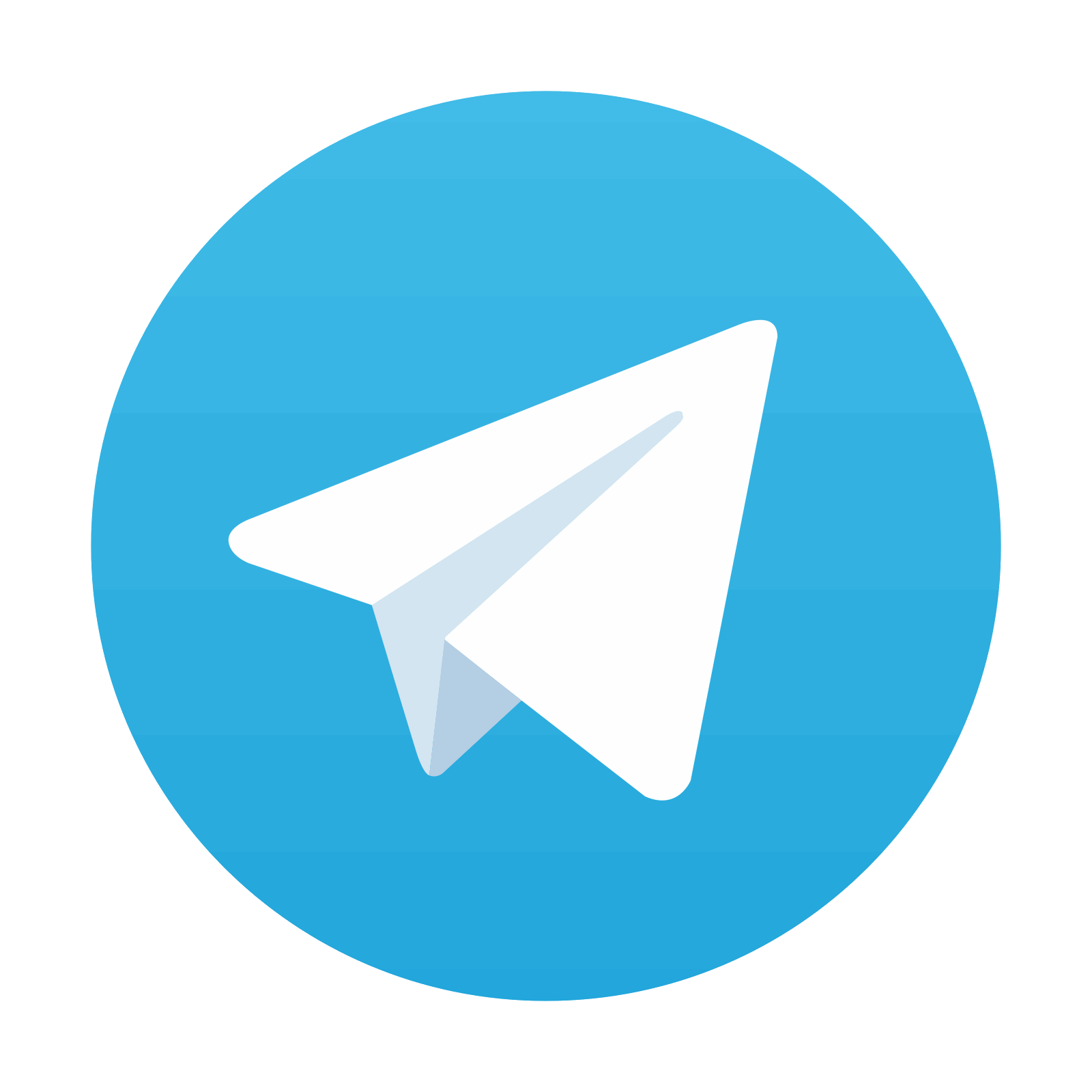
Stay updated, free articles. Join our Telegram channel

Full access? Get Clinical Tree
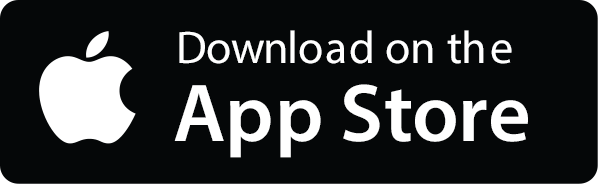
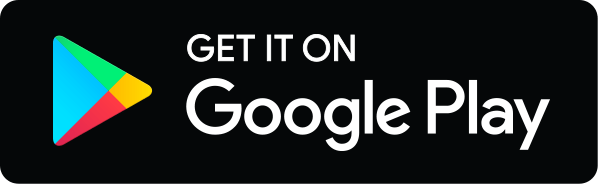
