Abnormalities of Movement and Posture Caused by Disease of the Basal Ganglia: Introduction
In this chapter, disorders of the automatic, static, postural, and other less-modifiable motor activities of the nervous system are discussed. They are an expression of what has come to be called the extrapyramidal motor system, meaning—according to S.A.K. Wilson, who introduced this term—the motor structures of the basal ganglia and certain related thalamic and brainstem nuclei.
The activities of the basal ganglia and the cerebellum are blended with and modulate the corticospinal system and the postural influence of the extrapyramidal system is indispensable to voluntary corticospinal movements. This close association of the basal ganglia and corticospinal systems becomes evident in the course of many forms of neurologic disease. In many aberrant motor patterns, one sees evidence not only of the activity of the basal ganglia but also of labyrinthine, tonic neck, and other postural reflexes that are mediated through nonpyramidal, bulbospinal and other brainstem motor systems. Observations such as these have blurred the original distinctions between pyramidal and extrapyramidal motor systems. Nevertheless, this division remains a useful concept in clinical work because it compels a distinction among several motor syndromes—one that is characterized by a loss of volitional movement accompanied by spasticity—the corticospinal syndrome; a second by bradykinesia, rigidity, and tremor without loss of voluntary movement—the hypokinetic basal ganglionic syndrome; a third by involuntary movements (choreoathetosis and dystonia)—the hyperkinetic basal ganglionic syndrome; and yet another by incoordination (ataxia)—the cerebellar syndrome. Table 4-1 summarizes the main clinical differences between corticospinal and extrapyramidal syndromes.
CORTICOSPINAL | EXTRAPYRAMIDAL | |
---|---|---|
Character of the alteration of muscle tone | Clasp-knife effect (spasticity) | Plastic, equal throughout passive movement (rigidity), or intermittent (cogwheel rigidity) |
Distribution of hypertonus | Flexors of arms, extensors of legs | Generalized but predominates in flexors of limbs and of trunk |
Involuntary movements | Absent | Presence of tremor, chorea, athetosis, dystonia |
Tendon reflexes | Increased | Normal or slightly increased |
Babinski sign | Present | Absent |
Paralysis of voluntary movement | Present | Absent or slight |
The Striatopallidonigral System (Basal Ganglia)
As an anatomic entity, the basal ganglia have no precise definition. Principally they include the caudate nucleus and the lentiform (lenticular, from its lens-like shape) nucleus with its two subdivisions—the putamen and globus pallidus. Insofar as the caudate nucleus and -putamen are really a continuous structure (separated only incompletely by fibers of the internal capsule) and are cytologically and functionally distinct from the pallidum, it is more meaningful to divide these nuclear masses into the striatum (or neostriatum), comprising the caudate nucleus and putamen, and the paleostriatum or pallidum, which has a medial (internal) and a lateral (external) portion. The putamen and pallidum lie on the lateral aspect of the internal capsule, which separates them from the caudate nucleus, thalamus, subthalamic nucleus, and substantia nigra on its medial side (Figs. 4-1 and 4-2). By virtue of their close connections with the caudate and lenticular nuclei, the subthalamic nucleus (nucleus of Luys) and the substantia nigra are included as parts of the basal ganglia. The claustrum and amygdaloid nuclear complex, because of their largely different connections and functions, are usually excluded.
Figure 4-2.
Diagram of the basal ganglia in the coronal plane, illustrating the main interconnections (see text for details). The pallidothalamic connections are illustrated in Fig. 4-3.
For reasons indicated further on, some physiologists have expanded the list of basal ganglionic structures to include the red nucleus, the intralaminar thalamic nuclei, and the reticular formations of the upper brainstem. These structures receive direct cortical projections and give rise to rubrospinal and reticulospinal tracts that run parallel to the corticospinal (pyramidal) ones; hence they also were once referred to as extrapyramidal. However, these nonpyramidal linkages are structurally independent of the major extrapyramidal circuits and are better termed parapyramidal systems. As the final links in this circuit—the premotor and supplementary motor cortices—ultimately project onto the motor cortex, they are more aptly referred to as prepyramidal (Thach and Montgomery).
Earlier views of basal ganglionic organization emphasized serial connectivity and the funneling of efferent projections to the ventrolateral thalamus and thence to the motor cortex (Fig. 4-3). This concept was based largely on the experimental work of Whittier and Mettler and of Carpenter, in the late 1940s. These investigators demonstrated, in monkeys, that a characteristic movement disorder, which they termed choreoid dyskinesia, could be brought about in the limbs of one side of the body by a lesion localized to the opposite subthalamic nucleus. They also showed that for such a lesion to provoke dyskinesia, the adjacent pallidum and pallidofugal fibers had to be preserved; that is, a second lesion—placed in the medial segment of the pallidum, in the fasciculus lenticularis, or in the ventrolateral thalamus—abolished the dyskinesia. This experimental hyperkinesia could also be abolished by interruption of the lateral corticospinal tract but not by sectioning of the other motor or sensory pathways in the spinal cord. These observations were interpreted to mean that the subthalamic nucleus exerts an inhibitory or regulating influence on the globus pallidus and ventral thalamus. Removal of this influence by selective destruction of the subthalamic nucleus is expressed physiologically by an irregular activity that is now identified as chorea, presumably arising from the intact pallidum and conveyed to the ventrolateral thalamic nuclei, thence by thalamocortical fibers to the ipsilateral premotor cortex, and from there, to the motor cortex, all in a serial manner.
Figure 4-3.
Schematic illustration of major efferent and afferent connections of the basal ganglia. The blue lines indicate neurons with excitatory effects, whereas the black lines indicate inhibitory influences. (See text for details; also Fig. 4-2.) (Reproduced with permission from Kandel ER, Schwartz JH, Jessell TM: Principles of Neural Science, 5th ed. New York: McGraw-Hill, 2013.)
New observations have made it apparent that there are instead, a number of parallel circuits as detailed further on. However, a general principle that has withstood the test of time is the central role of the ventrolateral and ventroanterior nuclei of the thalamus. Together, these nuclei form a vital link, not only from the basal ganglia but also from the cerebellum, to the motor and premotor cortex. Thus, both basal ganglionic and cerebellar influences are brought to bear, via thalamocortical fibers, on the corticospinal system and on other descending pathways from the cortex. Direct descending pathways from the basal ganglia to the spinal cord are relatively insignificant.
The foregoing view of basal ganglionic organization has been broadened considerably as a result of newer anatomic, physiologic, and pharmacologic data (see reviews of Gombart and colleagues, of DeLong, and of Penney and Young). Whereas earlier concepts emphasized the serial connectivity of the basal ganglionic structures as mentioned earlier, current evidence indicates an organization into several parallel basal ganglionic–cortical circuits. These circuits run parallel to the premotor pathway but remain separate anatomically and physiologically. At least five such anatomic circuits have been described, each projecting to a different portion of the frontal lobe: (1) the prototypical motor circuit, converging on the premotor cortex; (2) the oculomotor circuit, projecting onto the frontal eye fields; two prefrontal circuits: (3) one ending in the dorsolateral prefrontal and (4) the other on the lateral orbitofrontal cortex; and (5) a limbic circuit that projects to the anterior cingulate and medial orbitofrontal cortex.
An additional and essential feature of basal ganglionic structure is the nonequivalence of all parts of the striatum. Particular cell types and zones of cells within this structure appear to mediate different aspects of motor control and to utilize specific neurochemical transmitters, as detailed below under “Pharmacologic Considerations” (see also Albin et al and DeLong). This specialization has taken on further importance with the observation that one or another cell type is destroyed preferentially in degenerative diseases such as Huntington chorea.
The most important basal ganglionic connections and circuitry are indicated in Figs. 4-1, 4-2, and 4-3. The striatum, mainly the putamen, is the receptive part of the basal ganglia, receiving topographically organized fibers from all parts of the cerebral cortex and from the pars compacta (pigmented neurons) of the substantia nigra, and that the output nuclei of the basal ganglia consist of the medial (internal) pallidum and the pars reticulata (nonpigmented portion) of the substantia nigra (Fig. 4-3).
It has been proposed on the basis of physiologic, lesional, and pharmacologic studies, that there are two main efferent projections from the putamen; but these models are still in evolution. Nonetheless, there are reasons to conceptualize 1) a direct efferent system from the putamen to the medial (internal) pallidum and then to the substantia nigra, particularly to the pars reticulata, and 2) an indirect system originating in the putamen that traverses the lateral (external) pallidum and continues to the subthalamic nucleus, with which it has strong reciprocal connections.
In most ways, the subthalamic nucleus and lateral pallidum operate as a single functional unit, (at least in terms of the effects of lesions in those locations on parkinsonian symptoms and the neurotransmitters involved. The medial pallidum and reticular part of the substantia nigra can be viewed in a similar unitary way, sharing the same input and output patterns. Within the indirect pathway, an internal loop is created by projections from the subthalamic nucleus to the medial segment of the pallidum and pars reticulata. A second offshoot of the indirect pathway consists of projections from the lateral pallidum to the medial pallidonigral output nuclei. A complete account of this intricate connectivity cannot be given, but the main themes outlined here seem valid.
From the internal pallidum, two bundles of fibers reach the thalamus—the ansa lenticularis and the fasciculus lenticularis. The ansa sweeps around the internal capsule; the fasciculus traverses the internal capsule in a number of small fascicles and then continues medially and caudally to join the ansa in the prerubral field. Both of these fiber bundles join the thalamic fasciculus, which then contains not only the pallidothalamic projections but also mesothalamic, rubrothalamic, and dentatothalamic ones. These projections are directed to separate targets in the ventrolateral nucleus of the thalamus and to a lesser extent in the ventral anterior and intralaminar thalamic nuclei. The centromedian nucleus of the intralaminar group projects back to the putamen and, via the parafascicular nucleus, to the caudate. A major projection from the ventral thalamic nuclei to the ipsilateral premotor cortex completes the large cortical–striatal–pallidal–thalamic–cortical motor loop, with conservation of the somatotopic arrangement of motor fibers, again emphasizing the nexus of motor control at the thalamic nuclei.
In simplest physiologic terms, Denny-Brown and Yanagisawa, who studied the effects of ablation of individual extrapyramidal structures in monkeys, concluded that the basal ganglia function as a kind of clearinghouse where, during an intended or projected movement, one set of activities is facilitated and all other unnecessary ones are suppressed. They used the analogy of the basal ganglia as a brake or switch, the tonic inhibitory (“brake”) action preventing target structures from generating unwanted motor activity and the “switch” function referring to the capacity of the basal ganglia to select which of many available motor programs will be active at any given time. Still other theoretical constructs focus on the role of the basal ganglia in the initiation, sequencing, and modulation of motor activity (“motor programming”). Also, it appears that the basal ganglia participate in the constant priming of the motor system, enabling the rapid execution of motor acts without premeditation—e.g., hitting a baseball. In most ways, these conceptualizations restate the same notions of balance and selectivity imparted to all motor actions by the basal ganglia.
Physiologic evidence indicates that a balanced functional architecture, one excitatory and the other inhibitory, is operative within the individual circuits. The direct striatomedial pallidonigral pathway is activated by glutaminergic projections from the sensorimotor cortex and by dopaminergic nigral (pars compacta)–striatal projections. Activation of this direct pathway inhibits the medial pallidum, which, in turn, disinhibits the ventrolateral and ventroanterior nuclei of the thalamus. As a consequence, thalamocortical drive is enhanced and cortically initiated movements are facilitated. The indirect circuit arises from putaminal neurons that contain gamma-aminobutyric acid (GABA) and smaller amounts of enkephalin. These striatal projections have an inhibitory effect on the lateral pallidum, which, in turn, disinhibits the subthalamic nucleus through GABA release, providing subthalamic drive to the medial pallidum and substantia nigra pars reticulata. The net effect is thalamic inhibition that reduces thalamocortical input to the precentral motor fields and impedes voluntary movement. These complex anatomic and physiologic relationships have been summarized in numerous schematic diagrams similar to Fig. 4-4 and those by Lang and Lozano and by Standaert and Young.
Figure 4-4.
A. Schematic diagram of the main neurotransmitter pathways and their effects in the cortical–basal ganglia–thalamic circuits. The blue lines indicate neurons with excitatory effects; the black lines indicate inhibitory influences. The internal (medial) segment of the globus pallidus (MGP) and the zona reticulata of the substantia nigra (SNpr) are believed to act as one entity that projects via GABA-containing neurons to the thalamus (ventrolateral and ventroanterior nuclei) and to the pedunculopontine nuclei (not shown). Dopaminergic neurons (DA) arising in the pars compacta of the substantia nigra (SNpc) have an excitatory influence on the direct striatopallidal fibers (via D1 receptors) and an inhibitory effect on the indirect striatopallidal fibers (via D2 receptors) that project to the external (lateral) pallidum (LGP) and subthalamic nucleus (STN). Dotted lines in the subsequent figures denote a reduction in activity of the pathway. (See text.) B. Corresponding physiologic state as conceptualized in Parkinson disease, in which hypokinesia is the main finding as a result of reduced dopamine input from the substantia nigra and pars compacta to the striatum via the direct pathway, which results in withdrawal of inhibitory activity of the globus pallidus and, in turn, increased inhibitory drive on the thalamic nuclei, which reduces input to the cortical motor system. C. Schematic diagram of the theorized mechanism in Huntington disease, a hyperkinetic movement disorder resulting from reduced inhibition by the striatum within the indirect pathway, overdriving of the subthalamic nucleus, and causing excess activity in thalamocortical circuits. (See text.)
Restated, the current view is that enhanced conduction through the indirect pathway leads to hypokinesia by increasing pallidothalamic inhibition, whereas enhanced conduction through the direct pathway results in hyperkinesia by reducing pallidothalamic inhibition. The direct pathway has been conceived by Marsden and Obeso as facilitating cortically initiated movements and the indirect pathway as suppressing potentially conflicting and unwanted motor patterns. In Parkinson disease, e.g., loss of dopaminergic input from the substantia nigra diminishes activity in the direct pathway and increases activity in the indirect pathway; the net effect is to increase inhibition of the thalamic nuclei and to reduce excitation of the cortical motor system.
Further insight into these systems and into the mechanism of Parkinson disease has come from the discovery that the parkinsonian syndrome is reproduced in humans and primates by the toxin 1-methyl-4-phenyl-1,2,3,6-tetrahydropyridine (MPTP). This toxin was discovered accidentally in drug addicts who self-administered an analogue of meperidine. The toxin binds with high affinity to monoamine oxidase (MAO), an extraneural enzyme that transforms it to pyridinium, a toxic metabolite that is bound by melanin in the dopaminergic nigral neurons in sufficient quantities to destroy the cells, probably by interfering with mitochondrial function. In monkeys made parkinsonian by the administration of MPTP, electrophysiologic studies have shown increased activity in the medial globus pallidus and decreased activity in the lateral globus pallidus, as predicted from the above described models. This comes about because of the differential loss of activity of dopaminergic striatal neurons that project to each of these parts of the pallidum. The end result is increased inhibition of thalamocortical -neurons. It is, however, difficult to explain why medial pallidal lesions do not regularly cause parkinsonism. Perhaps it is because the subtle imbalance between the medial and lateral pallidal circuits that exists in Parkinson disease is not reproduced. This subtlety may also explain why crude lesions, such as infarcts, hemorrhages, and tumors, rarely produce the complete parkinsonian syndrome of tremor, bradykinesia, and rigidity. Indeed, striking improvements in parkinsonian symptoms are obtained, paradoxically, by placing lesions in the medial pallidum (pallidotomy) as discussed in Chap. 39.
It is likely that the static model of inhibitory and excitatory pathways and the parsing of a direct and an -indirect pathway, as useful as it is as a mnemonic, does not account well for the dynamic activities of the basal ganglia. In particular, the electrical activity of the neurons in these systems oscillate and influence the frequency of oscillations in other parts of the system, as well as bringing individual cells closer to firing. Another deficiency of currently conceived models is that they do not account for the tremor of Parkinson disease. To further complicate matters, the various subtypes of dopamine receptors act in both excitatory and inhibitory ways under different circumstances depending on their location as discussed below.
The manner in which excessive or reduced activity of various components of the basal ganglia gives rise to hypokinetic and hyperkinetic movement disorders is discussed further on, under “Symptoms of Basal Ganglia Disease.”
A series of pharmacologic observations have considerably enhanced our understanding of basal ganglionic function and led to a rational treatment of Parkinson disease and other extrapyramidal syndromes. Whereas physiologists had for years failed to discover the functions of the basal ganglia by stimulation and crude ablation experiments, clinicians became aware that certain drugs, such as reserpine and the phenothiazines, could produce extrapyramidal syndromes (e.g., parkinsonism, choreoathetosis, dystonia). These observations stimulated the study of central nervous system (CNS) transmitter substances in general. The current view is that the integrated basal ganglionic control of movement can be best understood by considering, in the context of the anatomy described above, the physiologic effects of neurotransmitters that convey the signals between cortex, striatum, globus pallidus, subthalamic nucleus, substantia nigra, and thalamus.
The most important neurotransmitter substances from the point of view of basal ganglionic function are glutamate, GABA, dopamine, acetylcholine, and serotonin. Figure 4-4 summarizes their roles. A more complete account of this subject may be found in the reviews of Penney and Young, of Alexander and Crutcher, and of Rao.
The following is what is known with a fair degree of certainty. Glutamate is the neurotransmitter of the excitatory projections from the cortex to the striatum and of the excitatory neurons of the subthalamic nucleus. GABA is the inhibitory neurotransmitter of striatal, pallidal, and substantia nigra (pars reticulata) projection neurons.
Acetylcholine (ACh), long established as the neurotransmitter at the neuromuscular junction and the autonomic ganglia, is also physiologically active in the basal ganglia. The highest concentration of ACh, as well as of the enzymes necessary for its synthesis and degradation (choline acetyl transferase and acetylcholinesterase), is in the striatum. Acetylcholine is synthesized and released by the large but sparse (Golgi type 2) nonspiny striatal neurons. It has a mixed but mainly excitatory effect on the more numerous spiny neurons within the putamen that constitute the main origin of the direct and indirect pathways described above. It is likely that the effectiveness of atropinic agents—which have been used empirically for many years in the treatment of Parkinson disease and dystonia—depends on their capacity to antagonize ACh at sites within the basal ganglia and in projections from the pedunculopontine nuclei. Acetylcholine also appears to act on the presynaptic membrane of striatal cells and to influence their release of neurotransmitters, as discussed below. In addition, the basal ganglia contain other -biologically active substances—substance P, enkephalin, cholecystokinin, somatostatin, and neuropeptide Y—which enhance or diminish the effects of other neurotransmitters, i.e., they act as neuromodulators.
Of the catecholamines, dopamine has the most -pervasive role but its influence can be excitatory or inhibitory depending on the site of action and the subtype of dopamine receptor. Disturbances of dopamine signaling are essential abnormalities of several CNS -disorders including parkinsonism, schizophrenia, attention deficit hyperactivity disorder, and drug abuse. Within the basal ganglia, the areas richest in dopamine are the substantia nigra, where it is synthesized in the nerve cell bodies of the pars compacta, and the termination of these fibers in the striatum. In the most simplified models, stimulation of the dopaminergic neurons of the substantia nigra induces a specific response in the striatum—namely, an inhibitory effect on the already low firing rate of neostriatal neurons. However, the effects of dopamine have proved even more difficult to resolve, in large part because there are now five known types of postsynaptic dopamine receptors (D1 to D5), each with a particular anatomic distribution and pharmacologic action. This heterogeneity is exemplified in the excitatory effect of dopamine on the small spiny neurons of the putamen and an inhibitory effect on others.
The five types of dopamine receptors are found in differing concentration throughout various parts of the brain, each displaying differing affinities for dopamine itself and for various drugs and other agents (Table 4-2; also see Jenner). The D1 and D2 receptors are highly concentrated in the striatum and are the ones most often implicated in diseases of the basal ganglia; D3 in the nucleus accumbens, D4 in the frontal cortex and certain limbic structures, and D5 in the hippocampus. In the striatum, the effects of dopamine act as a class of “D1-like” (D1 and D5 subtypes) and “D2-like” (D2, D3, and D4 subtypes) receptors. Activation of the D1 class stimulates adenyl cyclase, whereas D2 receptor binding inhibits this enzyme. Whether dopamine functions in an excitatory or inhibitory manner at a particular synapse is determined by the local receptor. As mentioned earlier, excitatory D1 receptors predominate on the small spiny putaminal neurons that are the origin of the direct striatopallidal output pathway, whereas D2 receptors mediate the inhibitory influence of dopamine on the indirect striatopallidal output, as indicated in Fig. 4-4.
CLASSES OF DOPAMINERGIC RECEPTORS | |||||
---|---|---|---|---|---|
D1 | D2 | D3 | D4 | D5 | |
Within basal ganglia | |||||
Striatum | +a | +b | + | + | + |
Lateral GP | + | + | |||
Subthalamic nucleus | + | + | + | ||
Medial GP/SN pars reticulata | + | ||||
SN pars compacta | + | + | + | ||
Outside basal ganglia | |||||
Nucleus accumbens | + | + | |||
Frontal cortex | + | + | |||
Limbic structures | + | ||||
Hippocampus | + | ||||
Hypothalamus | + | + | |||
Olfactory tubercle | + | ||||
Pituitary | + | ||||
Brainstem | + | ||||
Drug affinities | |||||
Dopamine | ++ | +++ | ++++ | N/A | N/A |
Bromocriptine | — | ++ | ++ | N/A | N/A |
Pergolide | + | ++++ | +++ | N/A | N/A |
Ropinirole | 0 | +++ | ++++ | N/A | N/A |
Pramipexole | 0 | +++ | ++++ | N/A | N/A |
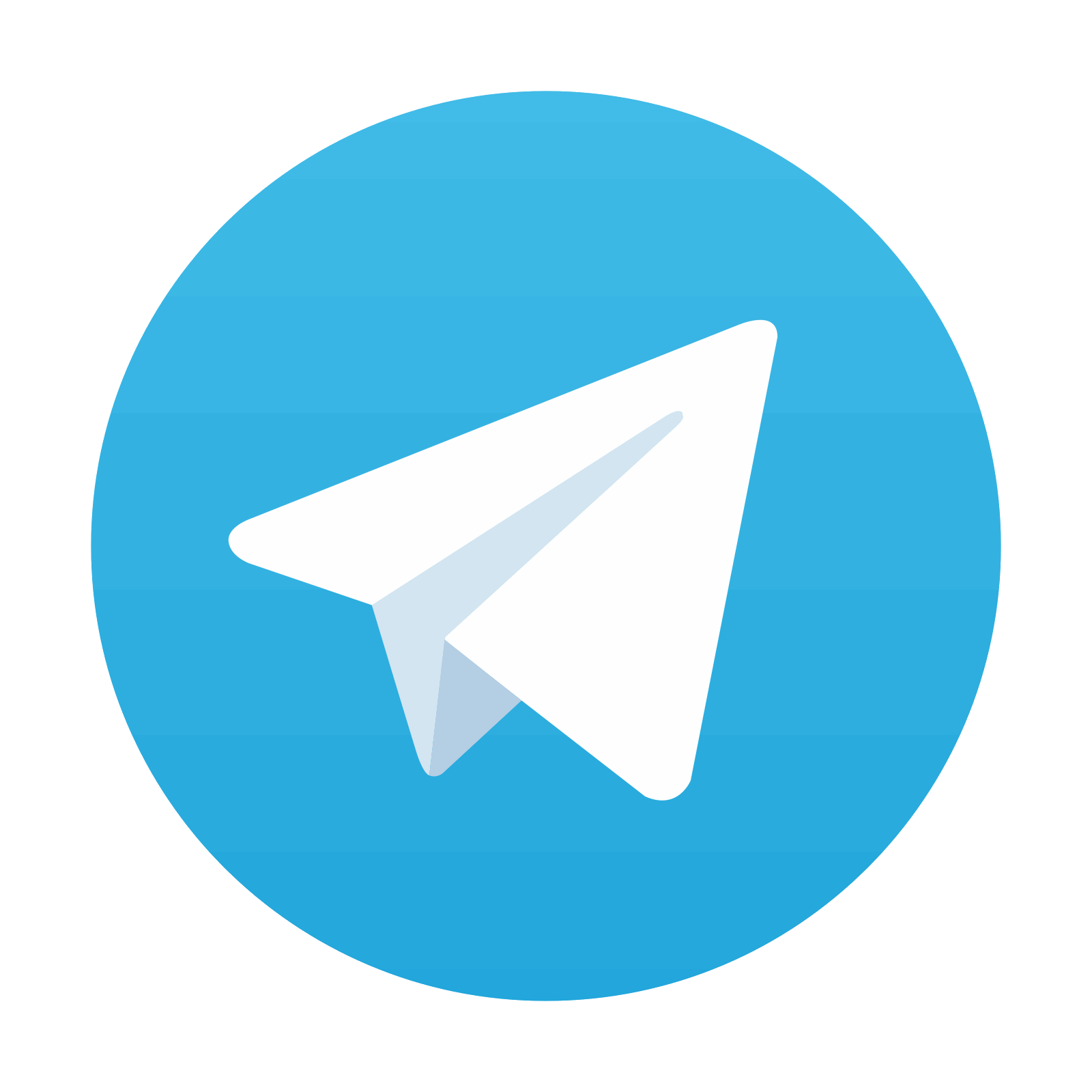
Stay updated, free articles. Join our Telegram channel

Full access? Get Clinical Tree
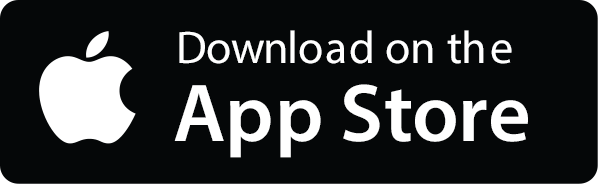
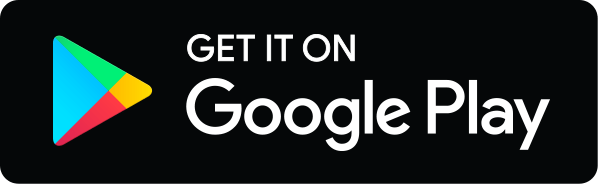