4.1 Introduction
While defined by the presence of recurrent seizures, epilepsy can be associated with abnormalities in cognition, psychiatric status, and social-adaptive behaviors.1 These complications of epilepsy, especially alterations in cognition including memory, have been shown to be associated with alterations in brain structure and function.2 While the comorbidities of epilepsy exert major adverse effects on quality of life of people with epilepsy,3 it has proven difficult to fully understand the etiology, timing, and course of abnormalities in both brain structure and cognition. A growing opinion has been that to better understand these issues it would be useful to examine patients near the time of the onset of their epilepsy and follow patients prospectively with the hope of identifying biomarkers of problematic courses.4, 5
In that spirit, the focus here is on children with epilepsy and what has been learned about the origin, development, and course of abnormalities in brain structure and cognition. We will begin by first briefly reviewing several trends that have been identified regarding cognitive and other neurobehavioral comorbidities of epilepsy and their potential neurodevelopmental roots. We then move on to what has been learned about the origin and course of these problems through studying children at or near the time of the onset of their epilepsy and following them prospectively over time using both objective measures of cognition and neuroimaging.
4.2 Childhood Onset Epilepsy
As noted, given the diverse medical, social, psychiatric, cognitive, and quality of life complications of the chronic adult epilepsies6–11 and their associated direct and indirect costs,12, 13 an increasing focus of interest has been on when, why, and how these problems develop and progress. At least three sets of evidence suggest a significant neurodevelopmental contribution.
First there is the indirect evidence linking the risk of comorbidities to the age of onset of recurrent seizures. For example, studying adults with chronic epilepsy, many neuropsychological studies have shown that an earlier age of onset of recurrent seizures is associated with poorer cognitive function than is chronic epilepsy with a later onset.14–19 This same relationship has been reported in some but not all neuropsychological studies of younger patients with focal impaired awareness and other types of seizures.20–22 Complementing these cognitive studies are neuroimaging investigations of brain structure. Although this is a more limited literature, it has been shown that childhood onset epilepsy is associated with reductions in whole-brain volume among patients with a history of complicated febrile convulsions,23 altered volumes of the corpus callosum, whole-brain white and/or gray matter tissue, and specific disrupted patterns of gray and white matter connectivity in individuals with childhood compared to adult onset chronic partial epilepsy.17, 24–27
Second is the compelling evidence that childhood onset epilepsy adversely impacts life course. An important indication of the public health significance of childhood epilepsy is its association with problematic outcomes in later youth and adulthood. This impact is reflected in an international literature published over the course of decades with studies emanating from the United Kingdom, Canada, Finland, Japan, Australia, and the Netherlands. Collectively, these reports include both uncontrolled28–34 and controlled community- and population-based investigations8, 35–41 as well as long-term (adult) follow-up of national birth cohorts.42–46 Groups of children with epilepsy have been followed prospectively for up to 30+ years in controlled investigations,39, 40 which has yielded a number of insights: (1) problems in a diversity of major life outcomes have been reported including rates of marriage, employment, income, psychiatric status, independent living status, and other critical aspects of quality of life; (2) while it would not be surprising that children with severe and complicated epilepsies would exhibit poor life span psychosocial outcomes,30–32 many but not all34 investigations report adverse life span outcomes in persons with intact intelligence, so-called benign epilepsies, and even persons with remitted childhood epilepsy who are no longer being treated with antiepilepsy medications;28, 36, 40, 41 (3) the causes underlying these adult outcomes and the factors associated with favorable versus unfavorable outcomes remain to be fully clarified, and these problematic life span outcomes are variably associated with clinical epilepsy features (e.g., seizure control); and (4) adverse life span outcomes have been found to be associated with histories of neurobehavioral cognitive and psychiatric comorbidities,28, 30, 37, 38 including what is now called attention deficit hyperactivity disorder (ADHD).30
Third, there is an extensive and widely appreciated set of background data that involve the direct examination of comorbidities among children with established epilepsy.47–49 This literature benefits from both population-based and careful tertiary care investigations (see Lin et al.1 for review).
However, to directly assess neurodevelopmental contributions to specific comorbidities of epilepsy and anomalies in brain structure, connectivity, and function and their course over time, a useful line of research would be to examine children at or near the time of diagnosis and follow them prospectively. There is a developing literature on this topic in regard to both cognition and imaging that will be reviewed below.
4.2.1 Cognition
Prior to 2000 only a small number of studies examined cognition in children with new-onset epilepsy.50–53 Several of these early papers tested children at or near diagnosis in order to serve as a drug-naïve baseline with which to assess subsequent treatment or comparative drug effects.52, 53 Others focused solely on intelligence and were primarily interested in characterizing clinical seizure factors associated with prospective IQ changes, especially declines.50 These studies examined children with epilepsy of various types, age ranges, test batteries, and clinical seizure characteristics. Comments that follow focus only on the baseline findings.
Bourgeois et al.50 examined intelligence in 72 children with mixed epilepsies within two weeks of diagnosis, age 18 months to 16 years (with IQ 40+), compared to 45 siblings. The mean IQ of the children with epilepsy (98.0) was lower than that of matched siblings (104.4), although this difference was not statistically significant. Children with idiopathic epilepsy had a higher IQ than symptomatic epilepsy (102.5 vs. 89.1) at baseline.
Stores52 examined 63 children (ages 7–12) with new-onset epilepsy both before and after initiation of antiepileptic drugs (AED) treatment and 47 similarly aged healthy controls. Measures of specific cognitive abilities (attention, memory, motor, and psychomotor speed) and general intelligence as well as reading and arithmetic performance were administered. At baseline, prior to AED initiation, the focal epilepsy group exhibited significantly poorer attention (ability to establish and focus attention) as well as poorer motor and psychomotor ability. Children with absence and other genetic generalized epilepsies exhibited fewer significant baseline cognitive abnormalities, with differences limited to motor ability and psychomotor speed.
Williams et al.53 compared 37 children with new-onset epilepsy (22 focal and 15 generalized epilepsy, ages 6–17) prior to initiation of AEDs and a control group of 26 children with new-onset diabetes on measures of attention and concentration, memory, complex motor processing speed, and behavior. The children with epilepsy performed more poorly than the diabetic children across 9 of 11 measures, but none of the group differences were statistically significant.
Kolk et al.51 compared 14 healthy controls, 12 children with focal seizures, and 18 with congenital hemiparesis and seizures (mean age = 6 years), the children with epilepsy said to be of normal psychometric intelligence. The NEPSY was used for cognitive assessment, which consisted of 37 tests falling into five cognitive domains (attention and executive function, language, sensorimotor, visuospatial, learning and memory). The children with new-onset partial epilepsy showed significantly poorer performance across four of the five composite cognitive domain scores.
This has become an area of increased interest and several recent investigations have appeared. In the seminal paper by Oostrom et al.,54 children (n = 51, ages 5–16) with newly diagnosed epilepsy were seen and assessed prior to the administration of antiseizure medications (tested within 48 hours of diagnosis). The children with epilepsy had at least two unprovoked nonfebrile seizures of idiopathic or unclear cause, attended mainstream schools, and were without any identified neurological disease, a diagnosis of another chronic illness, or previous use of AEDs. Controls were healthy classmates invited by the child with epilepsy and/or their family (n = 48). A comprehensive test battery was administered that was reduced through factor analysis to measures of attention, reaction time, intelligence, academic skills, learning, and behavior. Significantly poorer performance on the part of the children with new-onset epilepsy was observed in the areas of attention, reaction time, visual memory, and behavior with a trend (p = .07) of poorer academic achievement.54 From the time of this investigation there has been considerably more interest in children with new-onset epilepsies, and in the material to follow we will consider only those new-onset studies that included a control group for direct comparison.
Fastenau et al.55 examined a large community-based sample of children with first recognized seizures (n = 282 children, ages 6–14 years, IQ ≥ 70) and 147 sibling controls, characterizing their neuropsychological and academic status. They found that 27% of children with a single seizure and up to 40% of those with risk factors exhibited neuropsychological deficits at or near onset. Risk factors for neuropsychological deficit included multiple seizures (OR = 1.96), use of AEDs, symptomatic/cryptogenic etiology (OR = 2.15), and epileptiform activity on the initial EEG (OR = 1.9). Absence epilepsy also carried increased odds for neuropsychological impairment (OR = 2.0). Academic achievement appeared unaffected, suggesting an opportunity for early intervention (see Dunn et al.56 and McNelis et al.57 for additional information regarding academic achievement in both new-onset and chronic cases from the Indianapolis series).
Vintan et al.58 compared 18 children with drug-naïve new-onset benign epilepsy with centrotemporal spikes (BECTS) to 18 controls (mean age 8.88 and 8.22, respectively). Overall intellectual ability was assessed using Raven’s Progressive Matrices, and specific cognitive abilities were assessed using subtests from the Cambridge Neuropsychological Test Automated Battery (CANTAB), which included (1) induction: Motor Screening Test (MOT); (2) visual memory: Delayed Matching to Sample (DMS), Paired Associates Learning (PAL), Pattern Recognition Memory (PRM), and Spatial Recognition Memory (SRM) tests; and (3) executive functions: Spatial Span (SSP) and Spatial Working Memory (SWM) tests. The BECTS group exhibited normal performances for induction and executive functions, compared with control children. There were significant differences on the visual memory subtests (PRM percentage correct and SRM percentage correct), suggesting that children with BECTS had difficulties determining the accuracy of the pattern and spatial recognition memory.
Lee et al.59 examined 30 children with drug-naïve new-onset epilepsy (21 focal and 9 generalized epilepsies) and 25 health controls, ages 7–16, and compared them on the Korean version of the Wechsler Intelligence Scale for Children–III (WISC-III), Stroop Interference, and Trail Making Tests (A&B). The children with epilepsy had significantly worse WISC-III freedom from distraction and verbal IQ scores, as well as significantly longer response times on the Stroop Test color condition. Comparing the focal and generalized epilepsy groups, the only difference was lower verbal IQ in the focal epilepsy group.
Filippini et al.60 compared 15 children with drug-naïve new-onset BECTS and 15 healthy controls (mean ages 8.8 and 9.2 years, respectively) across a comprehensive test battery assessing language, executive function, academic skills, visuomotor and visuospatial skills, and short-term memory. The children with BECTS performed significantly worse on tests of language function (color naming, spoonerism, phonemic synthesis) and academic skills (word reading errors as well as mistakes in nonword writing and reading and nonword reading speed) and tests of executive function (five-point test correct and errors). They concluded that children with new-onset untreated BECTS showed a range of abnormalities in cognition, especially executive attention, despite normal IQ.
Cheng et al.61 examined 37 children with absence epilepsy (15 of whom were new-onset and drug-naïve) and 37 healthy controls (ages 8.0 and 8.5, respectively). A series of 10 cognitive tests were administered including Raven’s Progressive Matrices (fluid intelligence), Wisconsin Card Sorting Test (response inhibition and mental flexibility), visual tracing (visual attention), with control tasks including choice reaction time, number magnitude comparison, mental rotation, simple subtraction, word semantics, paired associate learning, and word rhyming. The drug-naïve group performed worse than controls on Raven’s Matrices, visual tracing, and the Wisconsin Card Sort. Interestingly, there were no differences between the drug-naïve group and the chronically treated patients.
Matricardi et al.62 examined 23 children with cryptogenic frontal lobe epilepsy and 23 children with idiopathic generalized epilepsy—both groups new-onset and drug-naïve in nature, and 18 healthy controls (ages 7.4 and 7.7, respectively). Participants were assessed on the WISC-IV and the NEPSY, which consisted of 32 subtests and 4 delayed tasks. All composite scores of the WISC-IV (FSIQ, VCI, PRI, WMI, PSI) were significantly lower in the children with frontal lobe epilepsy compared to the generalized epilepsy and control groups, who did not differ from one another. On the NEPSY, the frontal lobe group performed more poorly in almost all areas assessed including visuospatial processing skills, working memory, short- and long-term verbal and visuospatial memory, linguistic abilities, inhibition, and sustained attention.
Vannest et al.63 compared 34 drug-naïve children with new-onset BECTS with 48 typically developing children (ages = 7.9 and 8.08 years, respectively). The primary cognitive outcomes included the core subtests of the Clinical Evaluation of Language Fundamentals (CELF) to assess language skill, the Developmental Test of Visuomotor Integration (VMI), and the Grooved Pegboard Test to assess fine motor skill. Secondary outcomes included the Wechsler Abbreviated Scale of Intelligence (WASI) to measure general intelligence (5-year-olds were administered the Wechsler Preschool and Primary Scale of Intelligence–III [WPPSI-III]). Phonologic language skills were assessed using the Phonological Awareness and Rapid Naming subtests of the Comprehensive Test of Phonological Processing (CTOPP), and speed of processing was assessed using the Symbol Search and Coding subtests from the WISC-IV or from the WPPSI-III for 5-year-olds. Not all participants completed all testing. The authors found no significant differences between BECTS patients and controls on measures of general intellectual function, or visuospatial or language testing. There were significant differences in processing speed index and nondominant hand fine motor scores between groups with the BECTS group performing worse.
In a related later paper, Vannest et al.64 compared a subset of 24 children with recently diagnosed BECTS and 40 typically developing children. Tests included the WASI (younger subjects [5-year-olds] completed the WPPSI-III); language was assessed using core subtests from the CELF-IV. Nonlanguage tests included the Developmental Test of Visuomotor Integration–VI and the Symbol Search and Coding subtests from the WPPSI-III and the WISC-IV. There were no differences between the BECTS and control groups.
Wang et al.65 reported on full-scale IQ (WISC-CR FSIQ) in 20 drug-naïve children with new-onset childhood absence epilepsy (CAE) and 20 healthy age- and gender-matched controls (mean age 8.65 and 8.83, respectively). Compared to controls, there was no difference in FSIQ (105.4 and 106.9, respectively).
Ekmekci et al.66 examined 24 youth with new-onset, but not drug-naïve juvenile myoclonic epilepsy (JME) and 28 healthy control children (mean ages = 14.9 and 14.7, respectively). The cognitive tests included subtests from the Wechsler Intelligence Scale for Children–Revised (Information, Similarities, Arithmetic, Digit Span, Block Design, Object Assembly, Coding, and Picture Completion subtests); the California Verbal Learning Test–Children’s version (CVLT-C) for verbal memory; Wechsler Memory Scale–III (WMS-III) for visual memory; and the Trail-Making Test (A&B), Stroop Test (Color, Word, Color-Word Interference), and phonemic fluency (F, A, S) for executive function. There was no difference between the two groups in verbal learning/memory (CVLT) but the JME group performed significantly worse on measures of letter fluency, Trails B, and Stroop Test. The JME group also performed worse on Trails A, WMS-III Visual Memory subtest.
Cheng et al.67 compared 35 drug-naïve children with new-onset epilepsy (CAE) and 33 healthy controls (ages 7.3 and 6.8, respectively) on measures of general cognitive processing (i.e., psychomotor speed, spatial ability, memory, general intelligence, attention, and executive function), language processing (i.e., semantic comprehension), and mathematical processing (i.e., arithmetic and numerical magnitude processing). The new-onset CAE group had impaired performance compared to the control group on tests of general cognitive processing including Raven’s Progressive Matrices, visual tracing, and the Wisconsin Card Sorting Test, as well as on two arithmetic performance tests including number magnitude and simple subtraction. The authors concluded that children with new-onset CAE exhibited cognitive deficits in general intelligence, attention, executive functioning, and mathematical abilities; with subsequent analyses suggesting that attention abnormalities may be responsible for arithmetic performance deficits in patients with new-onset CAE.
In summary, cognitive and (at times) academic abnormality could be identified at or shortly after the onset of childhood epilepsy, even among children with uncomplicated idiopathic epilepsies—children with “epilepsy-only” per Oostrom et al.54 Findings varied across studies that varied in sample size, clinical seizure population, details of administered cognitive tests, and thoroughness of assessment. The general trend of detectable abnormality in cognition at onset, especially in drug-naïve patients, suggests that careful attention to neurobehavioral status is appropriate when treating children with new-onset epilepsies. These findings also suggest that there are early neurodevelopmental alterations occurring antecedent to epilepsy diagnosis and treatment which can be detected. How these cognitive problems may evolve over time is a topic that will be addressed later in this chapter.
4.2.2 Imaging
Similar to the cognitive literature, a limited number of neuroimaging studies of children with new-onset epilepsy have shown early abnormalities in brain structure. Our own group has examined brain structure,68, 69 white matter integrity,70 and ventricular volume71 in children with new-onset epilepsies at baseline and has detected abnormalities, and here we focus on investigations examining abnormalities in metrics of brain structure (e.g., cortical thickness or volume, subcortical volumes) and white matter integrity at baseline.
Widjaja et al.72 examined a mixed group of new-onset epilepsy (n = 29) and healthy controls (n = 23) focusing on cortical thickness (lobar) and subcortical volumes. They found reduced cortical thickness of the right cingulate, right medial temporal, and left frontal cortices in patients with new-onset seizures. Patients with generalized seizures did not demonstrate a significant reduction in cortical thickness; however, patients with focal seizures showed significant reduction in cortical thickness in these brain regions.
Yang et al.73 examined white matter integrity using DTI in 9 drug-naïve children (ages 5–12), not necessarily with new-onset epilepsy (duration from 0.4 to 4 years), with absence epilepsy and nine age- and sex-matched healthy controls. The untreated CAE patients exhibited significantly higher fractional anisotropy (FA) in the bilateral thalamus, anterior corpus callosum, and upper brainstem, with lower FA value in prefrontal white matter, anterior cingulate, and bilateral posterior limbs of the internal capsule compared to control subjects. Increased mean diffusivity (MD) was observed in parietal lobe white matter, prefrontal white matter, and posterior cerebellar hemispheres, as well as subcortical structures including bilateral putamen and posterior limb of the internal capsule.
Widjaja et al.74 examined white matter integrity using diffusion tensor imaging (DTI) in 35 children with new-onset epilepsy (12 with generalized and 23 with focal seizures, 17 of whom were not on medications) and 31 healthy controls (mean ages 9.4 and 10.7, respectively). Whole-brain FA, apparent diffusion coefficient (ADC), radial diffusivity (RD), and axial diffusivity (AD) maps were compared between groups. Evident was significantly reduced FA in left postcentral, elevated RD in left posterior cingulum and right external capsule, elevated AD in left middle temporal white matter and left thalamus, and reduced AD in left anterior cingulum, left temporal, and right supramarginal white matter in patients relative to controls. Children with focal epilepsy showed elevated RD in bilateral posterior cingulum; increased AD in left middle frontal; reduced AD in left temporal, right parietal, and right supramarginal white matter. Children with generalized epilepsy demonstrated increased AD in right cerebellum and reduced AD in left anterior cingulum and left middle temporal lobe white matter. Thus, white matter integrity was affected in children with new-onset seizures.
Kim et al.75 examined cortical thickness in children with new-onset BECTS (n = 20) and healthy controls (n = 20), with a subanalysis comparing BECTS participants with and without ADHD. Regional analysis of cortical thickness revealed that children with BECTS exhibited significantly greater cortical thickness in the right superior frontal gyrus, right superior and middle temporal gyri, and left pars triangularis gyrus. Children with BECTS also presented larger volumes of cortical gray matter of the right precuneus gyrus, left orbitofrontal gyrus, left pars orbitalis gyrus, and left precentral gyrus. In addition, patients with BECTS displayed significantly larger volumes of bilateral putamen and amygdala compared to healthy controls. Finally, BECTS patients with ADHD (n = 8) were shown to possess significantly thicker left caudal anterior and posterior cingulate gyri and a significantly larger left pars opercularis gyral volume compared to BECTS patients without ADHD.
Luo et al.76 examined 21 children with BECTS (average of 9 years old), 12 of whom were new-onset, and 20 healthy controls. Cortical and subcortical volumes were compared using voxel-based morphology (VBM) and resting-state functional connectivity analyses. Relative to controls, patients with BECTS showed significantly increased volume in the bilateral putamen, paracentral lobule, right anterior insula/frontal operculum, right supplementary motor area (SMA), right inferior temporal gyrus, and left cerebellum. Negative correlations were found between bilateral putamen volume and onset age of epilepsy; positive correlations were apparent between the volume of the right anterior insula/frontal operculum with the verbal and full-scale IQ scores.
Lee et al.59 examined children with new-onset epilepsy, average 3.4 months duration including 21 focal epilepsy (13 BECTS), 9 generalized epilepsy (9 JME), and 25 controls (aged ~10.5 years) and focused on gray and white matter volumes using VBM. They reported GM volume decreases in the left inferior frontal and right middle frontal gyri of patients compared with controls. Correlations with cognition found that lower verbal IQ scores were associated with decreased GM volumes in the left superior temporal and anterior cingulate gyri and decreased WM volumes in the left superior temporal and right parahippocampal gyri in the control group. However, no correlations were found between the verbal IQ score and GM/WM volumes in the epilepsy group. In addition, lower scores in freedom from distractibility were correlated with decreased WM volumes in the left frontal subgyral area, precuneus, and the superior parietal lobule as well as the right parahippocampal and middle temporal gyri in the control group. Lower scores in freedom from distractibility also were correlated with decreased GM volumes in the left postcentral gyrus in the epilepsy group. A longer response time on the Stroop Color Test was correlated with decreased GM volume of the right posterior lobe and decreased WM volumes of the right frontal subgyral and insular areas and part of the left sublobar region in the control group. In contrast, a correlation between Stroop Color Test response time and GM volume was observed only in the right superior temporal gyrus in the epilepsy group.
Wang et al.65 examined regional gray matter volume differences (VBM) in 20 drug-naïve children with new-onset CAE and 20 healthy age- and gender-matched controls (mean age 8.65 and 8.83, respectively). Compared to controls, the children with CAE demonstrated reduced gray matter volume in the bilateral thalami.
Ekmekci et al.66 examined white matter integrity (DTI) in 24 youth with new-onset, but not drug-naïve JME and 28 healthy control children (mean ages = 14.9 and 14.7, respectively). The investigators found significant decreases in FA value in dorsolateral prefrontal cortex, SMA, right thalamus, posterior cingulate, anterior corpus callosum, anterior corona radiata, and middle frontal white matter. Increased ADC was less significant in uncinate fasciculus and anterior. Furthermore, the authors found associations between cognitive measures and white matter integrity, which speaks to the clinical significance of the findings.
Perani et al.77 examined quantitative measures of gray matter volume in the thalamus, putamen, caudate, pallidum, hippocampus, precuneus, prefrontal cortex, precentral cortex, and cingulate in 29 AED-naïve patients with new-onset genetic generalized epilepsies and 32 age-matched healthy controls (mean ages 15.07 and 16.9, respectively). The thalamus was the only region that demonstrated reduced gray matter volume and shape analysis showed deflation which particularly affected a circumferential strip involving anterior, superior, posterior, and inferior regions with sparing of medial and lateral regions.
In summary, comparable to studies of cognition in children with new-onset epilepsies, a limited number of studies have demonstrated that brain abnormalities are present very early in the course of the disorder.
4.3 Tracking the Course of Cognition and Brain Development
Over the last 15 years we have been investigating a large cohort of children with new and recent onset idiopathic epilepsies using objective neuropsychological assessment, diverse neuroimaging techniques, and psychiatric assessment. These procedures have been repeated over time (2 years, 5 years) with examination of longer term (10 years) behavioral and psychosocial outcomes. By comparing the results to normally developing children, we have attempted to better characterize the presence, nature, and course of abnormalities in cognitive and brain development and their prognostic significance. Here we present direct comparisons of baseline and prospective cognitive and brain development in controls and participants with epilepsy.
4.3.1 Participants
Research participants consisted of 241 youth aged 8–18, including 134 with new and recent-onset epilepsy and 107 healthy first-degree cousin controls (see Table 4.1). The groups did not differ in terms of age, sex, or grade level. Full-scale IQ was lower in children with epilepsy (mean = 101.93, SD = 13.66) than in controls (mean = 109.35, SD = 11.31; p < .001), and academic problems were more common (epilepsy group = 48.5%, control group = 18.7%; p < .001; see Table 4.1 for demographic and clinical variables broken down by epilepsy subsyndrome). At baseline, all participants attended regular schools. Children with epilepsy were recruited from pediatric neurology clinics at three Midwestern medical centers (University of Wisconsin–Madison, Marshfield Clinic, Dean Clinic) and met the following inclusion criteria: (1) diagnosis of epilepsy within the past 12 months, (2) no other developmental disabilities (e.g., intellectual impairment, autism), (3) no other neurological disorder, and (4) normal clinical MRI. All children entered the study with active epilepsy diagnosed by their treating pediatric neurologists and confirmed by medical record review of the research study pediatric neurologist. We did not exclude children on the basis of psychiatric comorbidities (including ADHD) or learning disabilities. We did however exclude children with intellectual disorder, autism, and/or other neurological disorders. Details regarding the subject selection process have been described in detail in previous publications.68 In general, we tried to stay true to the concept of “epilepsy only” as defined broadly in the literature: normal neurological exams, intelligence, and attendance at regular schools. Each child’s epilepsy syndrome was defined in a research consensus meeting by the research pediatric neurologist who reviewed all available clinical data (e.g., seizure description and phenomenology, EEG, clinical imaging, neurodevelopmental history) while blinded to all research cognitive, behavioral, and neuroimaging data.
Table 4.1 Participant Characteristics of Controls and Epilepsy Participants by Syndrome
Variable | HC (n = 107) | BECTS (n = 41) | Focal (n = 51) | JME (n = 42) |
---|---|---|---|---|
Age (years) | 12.46 (3.00) | 10.32 (1.67) | 12.36 (3.01) | 14.85 (2.75) |
Gender (#/% female) | 54 (51%) | 15 (37%) | 24 (47%) | 25 (60%) |
FSIQ | 109.35 (11.31) | 104.44 (15.08) | 99.55 (12.83) | 102.38 (12.99) |
Academic problems | 19 (18%) | 22 (38%) | 27 (53%) | 19 (45%) |
Age of seizure onset (years) | — | 9.55 (1.68) | 11.34 (2.98) | 14.03 (2.88) |
Epilepsy duration (months) | — | 7.49 (3.33) | 8.37 (3.47) | 8.36 (3.44) |
Antiepileptic drugs (0/1/2+) | — | 17/24/0 | 8/38/5 | 1/39/2 |
Values are means/standard deviations or frequencies/%. HC = healthy controls; BECTS = benign epilepsy with centrotemporal spikes; focal = benign occipital epilepsy (n = 3), temporal lobe epilepsy (n = 20), frontal lobe epilepsy (n = 8), focal epilepsy NOS (n = 20); JME = juvenile myoclonic epilepsy.
First-degree cousins were used as controls and exclusion criteria were as follows: (1) history of any initial precipitating insult (e.g., simple or complex febrile seizures, cerebral infections, perinatal stroke), (2) any seizure or seizure-like episode, (3) diagnosed neurological disease, (4) loss of consciousness for a period greater than 5 min, (5) history of a first-degree relative with epilepsy or febrile convulsions. We used cousin controls rather than siblings or other potential control groups for the following reasons: (1) first-degree cousins are more genetically distant from the participants with epilepsy and thus less predisposed than siblings to shared genetic factors that may contribute to anomalies in brain structure and cognition, (2) a greater number of first-degree cousins are available than siblings in the target age range, and (3) the family link was anticipated to facilitate participant recruitment and especially retention over time (which is our intent) compared to more general control populations (e.g., unrelated school mates).
4.3.2 Neuropsychological Assessment
All participants were administered a comprehensive test battery that included measures of intelligence, academic achievement, language, immediate and delayed verbal memory, executive function, and speeded fine motor dexterity (see Table 4.2). Tests were selected not only for their pertinence to the cognitive domains of interest, but also for their broad applicable age ranges, so that the item pools were identical across the broad age range investigated here (as opposed to administering different versions of a test containing varying item pools to children across age categories), ensuring ability to directly and quantitatively characterize cognitive development. For the purposes of this presentation, all groups were compared across a subset of administered tests that were selected as representative of fundamental cognitive domains. Psychomotor function was assessed using a symbol substitution task (WISC-III Digit Symbol–Coding) and a simple motor task (Grooved Pegboard-dominant hand). The language domain was represented by the Boston Naming Test, a confrontation naming task. Verbal memory was assessed via a list-learning task from the Children’s Memory Scale. Finally, executive function was examined by measuring ability to inhibit prepotent responses on a Stroop-like task from the Delis-Kaplan Executive Function System (see Table 4.2 for full citations of all tests). All analyses used raw test scores and covaried age and sex. Patient performance in each group (BECTS, Focal, JME) were compared to controls using a 2 (Group: Patient vs. Control) × 2 (Time: Baseline vs. Follow-Up) mixed design ANCOVA for each of the six selected tests. Differences between patients and controls were examined at each time point. Significance was evaluated at p < .05; for all tests reaching significance, p values are given in parentheses. In all cases, patient performance was worse than that of control participants.
Table 4.2 Neuropsychological Tests by Domain
Domain | Ability | Test |
---|---|---|
Academic achievement | Letter/word recognition | WRAT-3 (reading)78 |
Letter and word writing | WRAT-3 (spelling) | |
Basic arithmetic | WRAT-3 (arithmetic) | |
Intelligence | Verbal | WASI (verbal IQ)79 |
Nonverbal | WASI (performance IQ) | |
Language | Confrontation naming | Boston Naming Test80 |
Expressive naming | Expressive Vocabulary Test81 | |
Receptive language | Peabody Picture Vocabulary Test–III82 | |
Generative naming | D-KEFS (letter fluency)83 | |
Memory | Verbal memory | CMS (word lists learning)84 |
CMS (word lists delayed) | ||
Executive function | Problem solving | D-KEFS (confirmed correct sorts) |
Response inhibition | D-KEFS (color-word interference test Inhibition) | |
Divided attention | D-KEFS (category switching accuracy) | |
Inattentiveness | CCPT-II (omission and commission errors)85 | |
Motor function | Speeded fine motor dexterity | Grooved Pegboard86 |
Psychomotor speed | WISC-III (digit symbol coding)87 |
4.3.3 Neuroimaging
Images were obtained on a 1.5 T GE Signa MRI scanner (GE Healthcare, Waukesha, WI, USA). Sequences acquired for each participant included (1) T1-weighted, three-dimensional spoiled gradient recall (SPGR) acquired with the following parameters: TE = 5 ms, TR = 24 ms, flip angle = 40 degrees, NEX = 1, slice thickness = 1.5 mm, slices = 124, plane = coronal, FOV = 200 mm, matrix = 256 × 256; (2) proton density (PD); and (3) T2-weighted images acquired with the following parameters: TE = 36 ms (for PD) or 96 ms (for T2), TR = 3,000 ms, NEX = 1, slice thickness = 3.0 mm, slices = 64, slice plane = coronal, FOV = 200 mm, matrix = 256 × 256. Images were transferred to a Mac OS X computer for processing with the FreeSurfer image analysis suite, a set of software tools for the study of cortical and subcortical anatomy that is documented and freely available for download online (http://surfer.nmr.mgh.harvard.edu). A brief technical presentation of these procedures as used in our laboratory is presented in Dabbs et al.88
4.4 Cognitive and Imaging Findings
In the material to follow we review the baseline cognitive and imaging findings at baseline and over a prospective 2-year period. This information will be reviewed by epilepsy syndrome, examining BECTS first, then JME, followed by a group of children with focal epilepsies (FE).
4.4.1 BECTS
Figures 4.1 and 4.2 depict the cognitive performances for children with BECTS and controls across all six measures of interest. Significant group main effects were found for tests of verbal learning (.032), coding (.027), and Grooved Pegboard (.006). No Group × Time interactions were found. Children with BECTS showed baseline deficits in confrontation naming (.037) and psychomotor speed/coding (.005), which were no longer significantly different from controls at follow-up. Deficits in verbal learning (.012) and simple motor function (Grooved Pegboard; .015), conversely, were present at both baseline and follow-up.
Figure 4.1. Plot of prospective changes in age- and gender-adjusted raw scores in intelligence, confrontation naming, and verbal learning for BECTS patients and healthy controls.
Figure 4.2. Plot of prospective changes in age- and gender-adjusted raw scores in inhibition (time to complete task), coding (number of correct responses), and motor sequencing (time to complete task) for BECTS patients and healthy controls.
In terms of imaging results, multiple regions of thinner cortex in BECTS subjects compared to control participants are shown in blue-cyan (Figure 4.7, top row). At baseline, thinner regions include bilateral rostral middle frontal gyrus (.005), right inferior frontal gyrus (.01), left inferior temporal gyrus (.05), left lateral occipital (.001), and right cuneus (.005). Figure 4.8 presents the annualized percent change in cortical thickness over two years for each group. Control subjects (top row) demonstrate widespread cortical thinning (blue-cyan). In contrast, BECTS subjects had only one small region of cortical thinning in the left isthmus cingulate (.020) and one region of cortical thickening (red) in the right precentral gyrus (.025). For the subcortical structures, a main effect of Time (Baseline, Follow-up) was found for the right putamen (.028), which represented a reduction in volume over time. Main effects of Group (BECTS, HC) were found for the left putamen (.010) and the right putamen (.017), with the children with BECTS exhibiting increased putamen volumes compared to HC. No significant Group × Time interactions were found for either left or right putamen.
4.4.2 JME
Figures 4.3 and 4.4 show the cognitive performance of the children with JME and controls. Significant main effects for Group were found for all tests (p values ranging from .001 to .029). There were no Group × Time interactions. JME patients performed significantly worse than controls on tests of inhibitory control (.003) and speeded motor performance (Coding, < .001; Grooved Pegboard, < .001) in addition to general IQ (.015). Performance on these tests continued to differentiate children with JME from healthy controls at follow-up (Inhibition, .046; Coding, .004; Grooved Pegboard, .023; IQ, .004).
Figure 4.3. Plot of prospective changes in age- and gender-adjusted raw scores in intelligence, confrontation naming, and verbal learning for JME patients and healthy controls.
Figure 4.4. Plot of prospective changes in age- and gender-adjusted raw scores in inhibition (time to complete task), coding (number of correct responses) and motor sequencing (time to complete task) for JME patients and healthy controls.
Turning to imaging findings, multiple regions of thinner cortex in JME subjects compared to control participants are shown in blue-cyan (Figure 4.7, bottom row). At baseline, thinner regions include left lateral occipital (.0001), left precentral (.015), and right superior parietal (.0008) regions. The cortical thinning over two years so evident in the control population is again absent in the JME subjects (Figure 4.8 top and bottom rows, respectively).
4.4.3 Focal Epilepsies
Figures 4.5 and 4.6 show performance of children with focal (temporal, frontal, benign occipital) epilepsy and controls. Significant main effects for Group were found for all tests (p values < .003) but inhibitory control. There were no Group × Time interactions. Focal patients scored lower than patients on each of these measures at both time points (ps from < .001 to – .049).
Figure 4.5. Plot of prospective changes in age- and gender-adjusted raw scores in intelligence, confrontation naming, and verbal learning for children with focal epilepsy and healthy controls.
Figure 4.6. Plot of prospective changes in age- and gender-adjusted raw scores in inhibition (time to complete task), coding (number of correct responses), and motor sequencing (time to complete task) for children with focal epilepsy and healthy controls.
In terms of imaging, compared to control subjects, the FE group exhibited thinner cortex in an area extending from inferior temporal to the fusiform gyrus (.009) (Figure 4.7, second row). There was bilateral cortical thinning with FE, albeit more restricted than in the controls. Cortical thinning regions are found in the left lateral occipital (.0001), left middle temporal (.038), right lateral occipital (.0001), right posterior cingulate (.033), and right caudal middle frontal (.0001) regions.
Figure 4.7. Thickness differences at baseline. Areas of thinner cortex compared to controls for the left and right hemispheres are indicated by the blue regions. Regions are found by a whole-brain vertex-wise analysis. Vertex-wise results have been cluster corrected while controlling for age and gender. Top row is BECTS, second row focal, and bottom row for the JME subjects.
Children with FE also exhibited larger bilateral putamen and right pallidum than controls (p < .05) at baseline. A significant Group × Time interaction was found for the right hippocampus (.011). The control subjects increased right hippocampus volume faster than the FE subjects.
Figure 4.8. Prospective change in cortical thickness for each group. Areas of the percentage change over two years for the left and right hemispheres are indicated by the colored regions. Blue represents cortical thinning; red represents cortical thickening. Regions are found by a whole-brain vertex-wise analysis. Vertex-wise results have been cluster corrected while controlling for age and gender. Top row is healthy controls, second row BECTS, third row focal, and bottom row for the JME subjects.
4.5 Conclusions
Across these common childhood epilepsy syndromes, cognitive abnormalities are present from the outset/very early in the course of the disorder. While classically there has been considerable interest in the unique cognitive effects of discrete epilepsy syndrome, these data help to appreciate the overlap that may be present and adversely affect cognition across epilepsy syndromes. While there appear to be few syndrome-specific effects, there are differences in the apparent magnitude of impact across syndromes. More importantly, following the children prospectively it is clear that there is little in the way of adverse progressive cognitive effects over time. That is, a common finding is that the differences between healthy controls and children with epilepsy present at baseline, the differences maintained two years later, with both groups often showing normal developmental change over time. Hence the cognitive “offset” noted at baseline is observed two years later to a similar degree, this seen in the context of normal cognitive development (or performance improvement) over the two-year interval. This pattern of results applies of course only to the syndromes examined here. It is surely possible and even likely that children with more severe and intractable epilepsies may show different prospective courses. At a minimum, however, these results imply that cognitive challenges are evident in children with even uncomplicated epilepsies early in the course of the disorder.
The pattern of imaging results is different compared to cognition. Differences in cortical thickness are present to a modest degree in all syndromes presented with an arguably modest degree of syndrome specificity. A primary signal is that there is a difference in the prospective trajectories of brain development in the children with epilepsy compared to normally developing children with the diffuse pattern of “cortical thinning” observed in controls being markedly attenuated in the children with active epilepsy, with some differences in these patterns across syndromes. This was found to be the case across the common childhood epilepsy syndromes examined here, true for JME, BECTS, and FE.
Interesting is the “dissociation” between the trajectories of the imaging and cognitive data. The imaging results in isolation would lead one to suspect that the cognitive trajectories would be quite different in the children with epilepsy, perhaps reflected in relatively arrested cognitive development, or slowed slopes of cognitive development over time, something clearly not the case here.
The long-term implications of these findings remain to be determined. For example, do patterns of cognitive impairment at baseline (or their short-term [2-year] trajectories), or imaging abnormalities, speak to epilepsy course and the potential for epilepsy remission in the future? Do the baseline and/or prospective imaging results predict longer term academic, psychiatric, or social outcomes?
The mean or average cognitive and imaging findings for the epilepsy syndromes examined here are just that, mean profiles. Within these average profiles is of course considerable individual variability, with some children more adversely affected than others, and some children not adversely affected at all. Of interest is the presence and significance of underlying cognitive or imaging phenotypes that may be present within the epilepsy syndromes and their implications. These and many other issues require careful investigation as we search for biomarkers of the neurobehavioral complications of epilepsy.
References







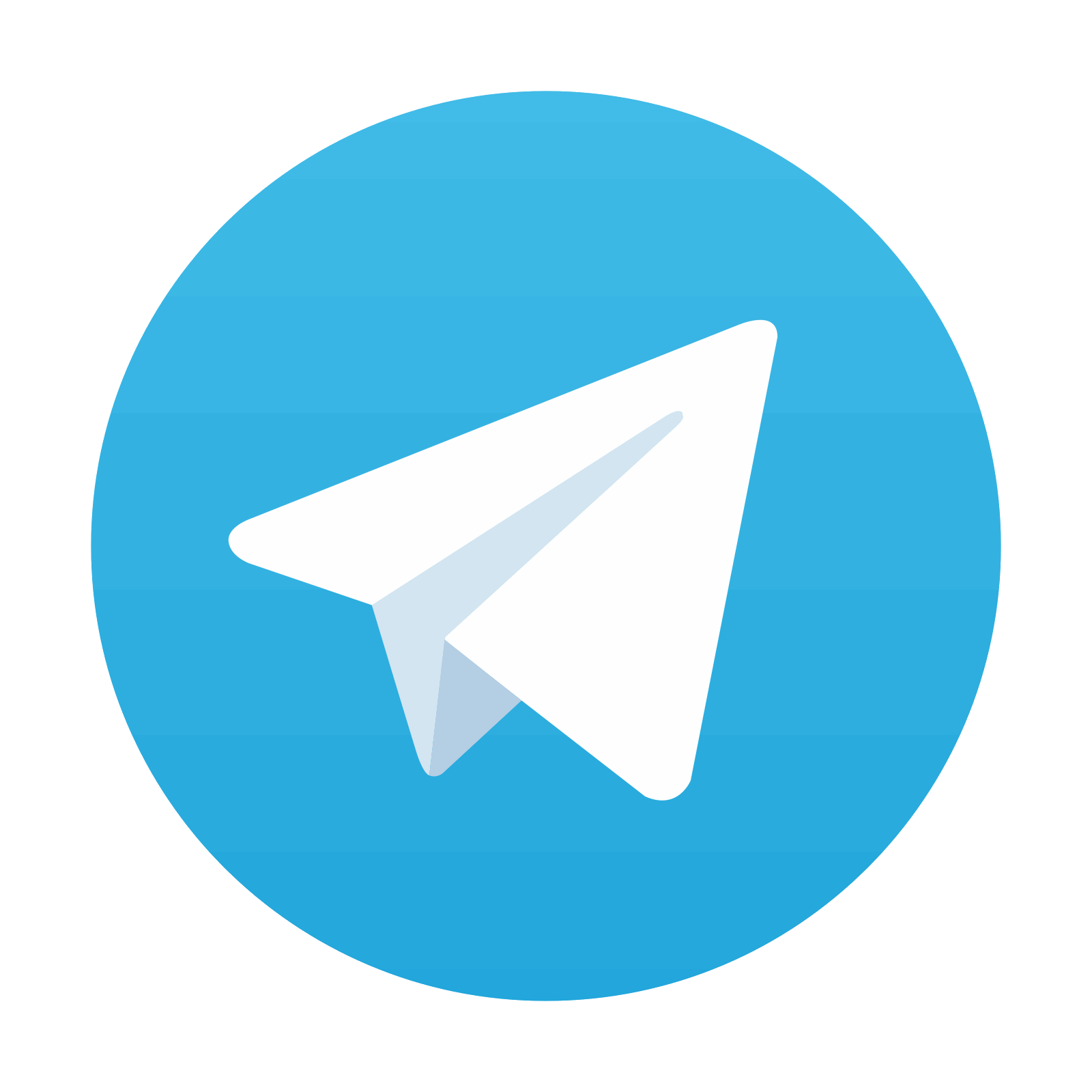
Stay updated, free articles. Join our Telegram channel

Full access? Get Clinical Tree
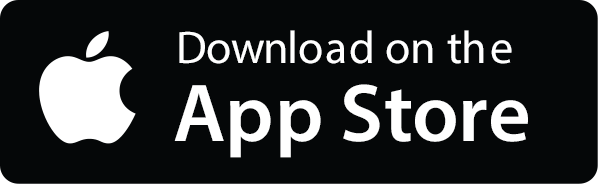
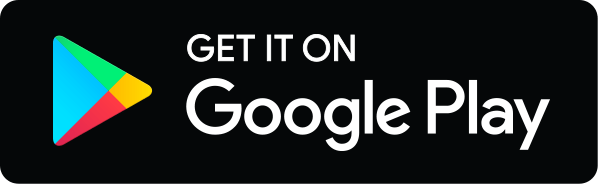
