5.1 Introduction
Temporal lobe epilepsy (TLE) represents the most common type of focal epilepsy in adulthood.1 Based on seizure semiology, TLE is divided into two main broad categories, the more common form with mesial temporal lobe symptoms, and the second with lateral temporal lobe symptoms. Traditionally, mesial TLE with hippocampal sclerosis (MTLE-HS) has been viewed as acquired, usually drug-resistant epilepsy associated with a history of prolonged febrile convulsions and usually drug-resistant epilepsy, which often requires surgical treatment.2 According to literature, MTLE with MTLE-HS carries a worse prognosis than other forms of epilepsy, reinforced by intensive investigation of refractory epilepsy patients for consideration of surgery.3 Indeed, long-term outcome studies from tertiary care centers provided evidence that only 10% of MTLE-HS patients were seizure-free, demonstrating the importance of HS as a major prognostic factor.4 This finding concurs with another study showing that with 10 years or more of follow-up about 50% of children with new-onset TLE became seizure-controlled with medications if the magnetic resonance imaging (MRI) was normal, and of those with lesions on neuroimaging none was likely to be seizure-free.5
Over the past two decades, studies from nonsurgical series of MTLE patients confirmed the existence of a benign form of MTLE (bMTLE), which is characterized by at least 24 months of seizure freedom with or without antiepileptic medication.6, 7 This entity of bMTLE appears to be a common and often unrecognized clinical entity, in which genetic factors play a major etiopathogenetic role.8 Moreover, the identification of MRI evidence of Hs in many cases with bMTLE has shown that Hs itself does not always mean intractable epilepsy.9 Very recently, however, a prospective longitudinal cohort study illustrated that bMTLE patients with radiologic evidence of HS since recruitment carried a three times higher likelihood of becoming refractory later in life than those without HS.10 Additional risk factors that contributed to refractoriness at long-term follow-up were earlier age of onset, longer duration of epilepsy, and antecedents with febrile seizures.10 These findings indicate that bMTLE is a syndrome representing the mildest form of the wide spectrum of MTLE, which includes both drug-responsive and refractory MTLE along a biological continuum.10 Most importantly, bMTLE does not symbolize a prerefractory stage of MTLE.
This group of bMTLE patients offers a superb opportunity not only to determine the biologic substrates underlying MTLE, but also to identify biomarkers for this epilepsy syndrome. Predictive biomarkers are becoming increasingly important tools in drug development and clinical research, and represent the new frontier for researchers in epilepsy to definitively improve the management of people with epilepsy.11 It has been recently claimed that a first step to identify potential biomarkers for pharmacoresistance may be to classify several well-defined epilepsy syndromes that are associated with drug resistance but in which there are also patients who are well controlled, which this cohort of bMTLE suitably symbolizes.12 This would, in turn, allow the development of biomarkers that can determine future drug response in new-onset cases, thus helping specialists to guide more precise decision making.
5.2 Genetics of bMTLE
Familial MTLE was first recognized in twin families, where its strong genetic basis was demonstrated by high concordance in monozygotic twins.13 It is noteworthy that the clinical, EEG, and imaging features, as well as seizure outcome of familial MTLE, where cases were selected because of a family history, are very similar to those described in unrelated cases with bMTLE.6, 8, 9 Indeed, the clinical features of familial MTLE included onset typically during the teenage years or early adult life, with no antecedent factors for epilepsy.13, 14 Febrile seizures occurred in only 2.7% of family members of the familial MTLE subjects, a frequency similar to that reported (3–4%) in the general population.14 The nature of the aura suggested mesial temporal origin, and déjà vu and jamais vu appeared to be overrepresented in this group of patients.14 Focal seizures with impaired awareness and especially focal to bilateral tonic-clonic seizures were infrequent, and the EEG recordings often showed no epileptiform abnormalities. Similar to sporadic bMTLE, the mild nature of this hereditary epilepsy may well explain why it could so often be undiagnosed. Similar families were also reported by other authors,15 with an overrepresentation of migraine in some of them. Also, in some of these patients with familial MTLE Hs was later found on MRI. Similar to sporadic MTLE, there may be a remarkable intra- and interfamilial phenotypic heterogeneity with respect to history of febrile seizures, severity of the epilepsy, and presence of Hs.16
All these clinical similarities between sporadic and familial bMTLE are in line with the overwhelming evidence suggesting that familial MTLE is mainly a polygenic disorder with a high heritability but absence of dominant segregation that shares the genetics determinants with apparently sporadic bMTLE beginning in adolescence or adulthood.7, 14 Such a proposal of common polygenic and rare dominant inheritance in MTLE predicts the allelic structure of bMTLE to be similar to that of idiopathic generalized epilepsies.14 Therefore, the common approaches of chromosomal linkage mapping in affected families and further in identification of candidate genes have so far not been doing well for heritable diseases like bMTLE that involves multiple genes and their interactions, and also the gene-environment interactions.
Indeed, no genes responsible for familial MTLE have been so far identified, and only one genetic locus has been mapped to chromosome 4q in a family with evidence of autosomal dominant inheritance.17 Occasionally, familial MTLE can occur in the context of familial focal epilepsy with variable foci related to mutations of the mTORC1-repressor DEPDC5 (DEP domain-containing protein 5) gene.18 It can also coexist in the context of families with dominant inheritance of febrile seizure syndromes including genetic epilepsy with febrile seizures plus. These are genetically heterogeneous with a variety of loci being reported and, in some families, the sodium channel genes SCN1A and SCN1B have been implicated.19, 20 Very rarely, familial MTLE may also be the presenting feature of choreoacanthocytosis and may delay its diagnosis.21 Although some polymorphisms (prodynorphin gene, Apoɛ gene, GABA B receptor gene, prion protein gene) have been described in MTLE patients,8 there are no such data deriving from kindreds with familial MTLE. No families with MTLE were found to have an LGI-1 mutation, even though some had affected family members with both mesial symptoms and auditory features. This further supports the notion that familial MTLE and familial lateral TLE constitute separate genetic syndromes.
5.3 bMTLE and Imaging Genetics
Identifying genes in complex disorders is a major current challenge across all diseases including MTLE, and the major strategies being used to explore their molecular basis are genome-wide association studies for common genetic variants or deep sequencing for rare variants.14 These types of approach are likely to be complemented by endophenotype studies, including brain imaging, which combines genetic assessment with multimodal neuroimaging to discover neural systems linked to genetic abnormalities or variation. The concept of endophenotype, first introduced in the psychiatric genetic literature, is extremely attractive.22 Endophenotypes are relatively simple, stable biologic phenomena that can be measured objectively and are genetically determined. In theory, although the disorder itself will usually result from a mixture of multiple genetic and nongenetic abnormalities, the endophenotype, as a simpler biologic phenomenon, will have a more straightforward genetic and pathophysiological basis.22
This method may be constructively applied to all epilepsies, especially MTLE. Epilepsy, indeed, encompasses a spectrum of clinical phenotypes, defined by EEG, seizure type, and brain imaging criteria. Reducing such complex phenotypes into components, whether they are neurophysiological, biochemical, endocrine, neuroanatomical, cognitive or neuropsychological, is described as an endophenotype strategy or approach.22, 23 Since there is an imperfect relationship between genes and epilepsy phenotypes so that different combinations of genes (and resultant changes in neurobiology) may contribute to any complex epilepsy, it may be possible to assay the result of aberrant genes through more biologically “simple” approaches. In theory, although the disorder itself will usually result from a mixture of multiple genetic and nongenetic abnormalities, the endophenotype, as a simpler biologic phenomenon, will have a more straightforward genetic and pathophysiological basis.
There is no reliable method to select behavioral or biological markers for studies aimed at evaluating endophenotypes. Generally, a marker should be selected with respect to several criteria including feasibility and reliability of its measurement, high heritability, availability of knowledge on its underlying neurobiology/genetics, and possible relevance for the disorder under study.23 To address that endophenotype identified in probands is highly heritable, a study design that compares prevalence rates of a marker between subjects at familial risk of the disease and those without the risk is a powerful strategy.23 In this way, to function as an endophenotype, a marker must be found in the unaffected relatives at a higher rate than in the general population.
In the field of epilepsy, potential endophenotypes may include electroencephalographic features and abnormal responses to provocation tests such as the intermittent photic stimulation, structural, or functional imaging findings. Much work had been done on the genetics of epilepsy-related endophenotypes in the 1980s and 1990s, but success in identifying relevant disease genes had been very unsatisfactory. Novel impetus for epilepsy-related endophenotypes is now coming from advanced imaging studies including functional MRI and connectivity studies, and the endophenotypes used in this context are usually called intermediate phenotypes. The intermediate phenotype strategy is based on the assumption that gene effects at the level of the brain are a more direct effect of genetic variation than is complex behavior, and will show association in carriers of risk alleles even if the carriers show no clinical diagnostic characteristics.24 There are two concepts of imaging genetics as an intermediate phenotype that may be applied to MTLE.24, 25 One model is that imaging genetics may allow the discovery of risk genes for MTLE; the second assumption is that imaging genetics may allow discovering reproducible effects of single gene variants on simple brain measures,25 for example, the hippocampus volume or other subcortical regions implicated in MTLE. These two concepts have different hypotheses, but the methods to test the hypotheses are the same and include association analyses between neuroimaging data and genetic variations.24, 25
5.3.1 Imaging Genetics of Hippocampal Morphology in MTLE
The mesolimbic system, especially the hippocampus, is well known for being involved in the pathogenesis of bMTLE. Notably, size and morphology of the hippocampus shows considerable variation in the general population and is estimated to be sufficiently heritable.26 Therefore, this structure may be an attractive candidate for genetic correlation based on the approach of an intermediate phenotype/endophenotype. Accordingly, results from neuroimaging studies have illustrated that genetic factors may contribute to altered hippocampal morphology in MTLE and may thus serve as promising phenotypes in the search for TLE genes. Indeed, a recent advanced neuroimaging study has shown that first-degree relatives of MTLE-HS patients, who have never had seizures, have smaller and more asymmetric hippocampi compared with age- and sex-matched controls.27 Conversely, none of them had Hs on careful qualitative and quantitative analyses, consistent with previously published data showing discordance for Hs in monozygotic twins.28 The latter observation would imply that the heritability of Hs itself may be very low. Important, the results of the advanced neuroimaging study are largely compatible with observations from other two studies of families with MTLE. A study of 23 individuals from two German families with MTLE showed that many members had febrile seizures, and all of them had hippocampal atrophy, as did 6 of 10 asymptomatic individuals.29 Similarly, in a series of 11 Brazilian kindreds with familial MTLE, about one-third of asymptomatic relatives had evidence of hippocampal volume loss.30 Moreover, small hippocampus with incomplete rotation and deformation was also shown to be related to haploinsufficiency of the SOX2 gene.31 Even though no evidence emerged that SOX2 mutation or variation could contribute commonly to MTLE or Hs in humans,31 SOX2 dysfunction might also be an additional candidate mechanism for mesial temporal abnormalities associated with MTLE.
Taken together, these results support a nontrivial association between morphometric brain abnormalities of the anterior-limbic neural substrate and family history of MTLE, which may become a potential candidate as a morphological endophenotype of MTLE (Figure 5.1). Since there is good evidence that hippocampal shape and positioning abnormalities are more prevalent in MTLE patients,32 it is tantalizing to hypothesize that, in either sporadic or familial bMTLE, patients may carry subtle morphologic abnormalities of the hippocampus with susceptibility for developing the disease including Hs in the presence of environmental or additional genetic factors, resembling complex inheritance. In this way, it is reasonable to hypothesize that hippocampal shape and positioning abnormalities may be used to help identify susceptibility alleles for Hs. Obviously, there is the need to determine the relationship between MRI and histological findings in order to clarify the spectrum of hippocampal developmental abnormalities and their significance in the genesis of MTLE or Hs.
Figure 5.1. (a, b) Brain MRI of a 31-year-old woman with bMTLE. (a) Coronal T1-weighted image shows left deeper and vertical collateral sulcus together with the reduction of the horizontal part of the parahippocampal gyrus. (b) Coronal FLAIR image shows left hippocampal hypointensity. (c, d) Brain MRI of the woman’s unaffected 59-year-old mother. (c) Coronal T1-weighted image shows signs of left hippocampal malrotation with deeper and vertical collateral sulcus together with the reduction of the horizontal part of the parahippocampal gyrus. (d) Coronal FLAIR image shows a regular signal intensity of the hippocampal formation bilaterally.
5.3.2 Imaging Genetics of Cortical and Subcortical Structures in MTLE
Several advanced morphometric and functional MRI studies have clearly demonstrated that extrahippocampal and extratemporal regions are involved in the epileptic network underlying MTLE, as well as that alterations of brain tissue integrity in gray and white matter are found in patients with MTLE regardless of the presence of Hs. Cross-sectional and longitudinal structural MRI studies in drug-resistant MTLE have shown correlations with disease duration in both neocortical and mesiotemporal regions, in contrast to work in bMTLE, likely representing seizure-induced damage.33 Conversely, there seems to be a resemblance of findings in benign and refractory cases with regard to cortical atrophy in sensorimotor regions. Indeed, the involvement of neocortical, especially sensorimotor, regions remote from the seizure focus was initially reported in refractory MTLE groups regardless of the presence of Hs.34 Afterward, an advanced imaging study illustrated significant cortical thinning within the sensorimotor cortex, principally in the postcentral gyrus bilaterally in patients with bMTLE regardless of MRI signs of Hs as well as the side of Hs.35 Thus, the sensorimotor cortex may be involved in the epileptogenic network underlying MTLE, as suggested by experimental data demonstrating that the hippocampus is associated with sensorimotor processes, especially innate processes involving control of motor responses to sensory stimuli, and that hippocampal mechanisms can directly influence locomotor activity.36
Likewise, a voxel-based morphometry (VBM) MRI study in patients with bMTLE demonstrated structural abnormalities located in the hippocampus and thalamus that were strikingly similar to previous findings in VBM studies of patients with refractory MTLE.37 The severity and extent of volume loss seen on brain VBM was found to parallel the volume loss detected on routine MRI study, being more severe in patients with routine MRI evidence of Hs. In an updated series of 85 sporadic bMTLE patients with normal MRI, VBM analysis confirmed a reduction in gray matter volume in the thalamus, bilaterally (Figure 5.2). Since these patients with bMTLE had a very mild epileptic disorder with no seizures at long-term follow-up, it is tempting to hypothesize that the thalamus is primarily caught up in the epileptogenic network underlying the disease. This view is supported by the observation of no significant correlation between either the duration of the epilepsy or the age at onset and extent of brain damage in these patients with MTLE.37
Figure 5.2. Voxel-based-analysis of the whole brain shows gray matter decrease of the bilateral thalami in a series of 85 patients with bMTLE.
Overall, these findings suggest that cortical and subcortical brain structures might represent attractive candidate endophenotypes reflecting particular genetic risk factors highly relevant to MTLE. A recent study examined volume deficits for many subcortical structures traits in unrelated patients with sporadic MTLE and their unaffected siblings with the aim of studying the suitability of these traits as endophenotypes.38 The advanced neuroimaging study, however, failed to depict any subcortical volume deficits in the unaffected individuals, suggesting that volume deficits in subcortical structures might be largely determined by nongenetic factors in sporadic MTLE.38 To the best of our knowledge, no study has assessed MTLE-related cortical gray matter alterations, which may represent a more suitable endophenotype in MTLE.
Interestingly, very recently a significant reduction of fractional anisotropy along the white matter of the temporal lobes in drug-resistant MTLE has been found, implying it as a valuable biological marker of refractoriness.39 Furthermore, the evidence of both diffusion abnormalities and reduced cortical thickness of the corpus callosum only in patients with refractory MTLE suggested that differences in the distribution of such alterations might be also related to refractoriness.40
5.4 Conclusions and Future Directions
Brain images offer a powerful means to assess the genetic effects, so the expectation is that the combination of the genetic and neuroimaging approaches has introduced a new era of imaging genetics that will allow an unprecedented amount of novel scientific insights related to the underlying biology and genetics of epilepsies, especially MTLE. Nonetheless, since brain imaging can measure signals at over 2 million locations in the brain, and each signal is surveyed while screening the genome as well, the resulting statistical search is so vast that very large sample sizes are needed to achieve meaningful results and depict genetic variants that influence the brain.25, 26 A relatively limited sample size was also a major limit of recent attempts to elucidate the genetic architecture of complex epilepsies other than a variety of issues including phenotypic ambiguities and restricted genetic scope.41 Most important, significantly associated single nucleotic polymorphisms have an expected effect size <0.5% of the phenotypic variance in quantitative traits, even for measures extracted from MRI.25, 26
Therefore, only a large sample size achieved through international collaboration and new methods might overcome the difficulty in detecting a true signal of a small effect size. So, with the recent advent of global neuroimaging consortia, like the ENIGMA Consortium (http://enigma.ini.usc.edu), imaging studies are now becoming well powered to depict genetic variants that reliably affect the brain.25, 42 Another challenge is that most genetic variations found by genome-wide association studies are located in regions where the functional consequences of the variants are unknown, so there is the need on turning new gene variants related to the disease into biological pathways linking associated genes, and the identification of functional annotations, some of which are cell type specific, enriched in disease associations.
Even so, imaging studies can already provide information that is not available in genetic comparison of patients and controls. Imaging can identify potential mechanisms and circuits promoting disease risk, making it easier to develop and evaluate treatments.42 A deeper understanding of disease-modifying pathways in MTLE should also make it easier to discover disease-modifying factors that drive resilience and risk for MTLE, which could lead to clinically relevant markers of prognosis and outcome.
References




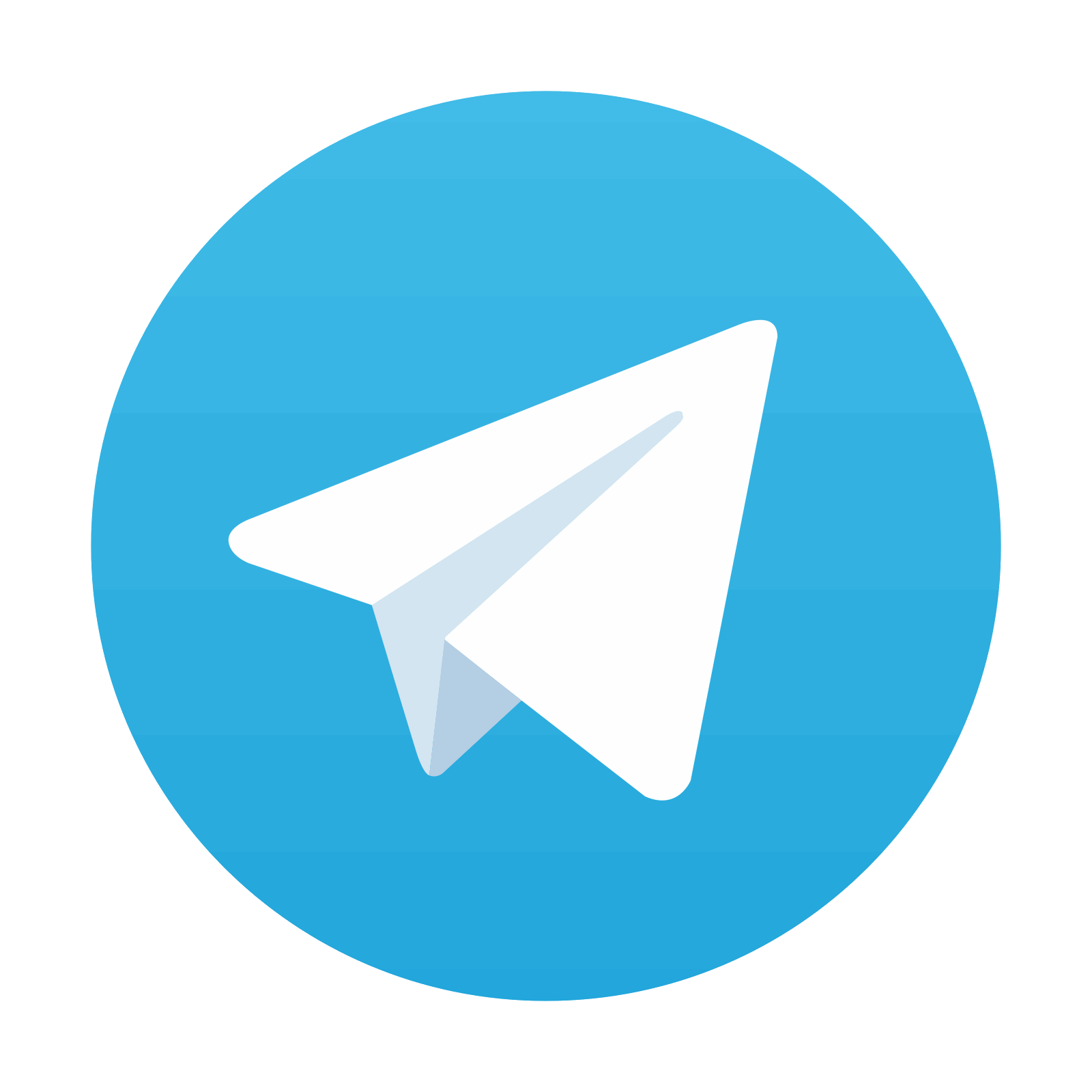
Stay updated, free articles. Join our Telegram channel

Full access? Get Clinical Tree
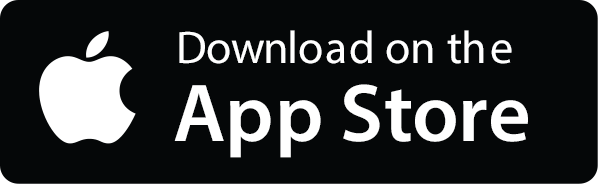
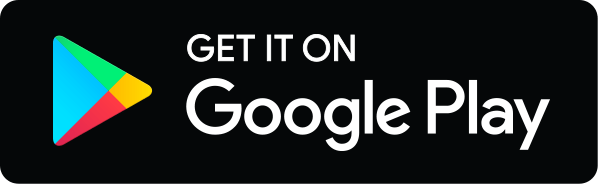