The term electromyography refers to methods of studying the electrical activity of muscle. Over the years, such methods have come to be recognized as an invaluable aid to the diagnosis of neuromuscular disorders. As is discussed in this chapter, electromyography (EMG) has been used to detect and characterize disease processes affecting the motor units and to provide a guide to prognosis. Electromyographic examination is often particularly helpful when clinical evaluation is difficult or equivocal. The findings commonly permit the underlying lesion to be localized to the neural, muscular, or junctional component of the motor units in question. Indeed, when the neural component is involved, the nature and distribution of EMG abnormalities may permit the lesion to be localized to the level of the cell bodies of the lower motor neurons or to their axons as they traverse a spinal root, nerve plexus, or peripheral nerve. The EMG findings per se are never pathognomonic of specific diseases and cannot provide a definitive diagnosis, although they may be used justifiably to support or refute a diagnosis advanced on clinical or other grounds.
Electromyography is also used in conjunction with nerve conduction studies to obtain information of prognostic significance in the management of patients with peripheral nerve lesions. For example, EMG evidence of denervation implies a less favorable prognosis than otherwise in patients with a compressive or entrapment neuropathy. Again, evidence that some motor units remain under voluntary control after a traumatic peripheral nerve lesion implies a more favorable outlook than otherwise for ultimate recovery, indicating as it does that the nerve remains in functional continuity, at least in part.
The clinical utility of electrodiagnostic testing in patients presenting with a chief complaint of weakness has been examined. The referring diagnosis was compared with the diagnosis immediately after electrophysiologic evaluation, and then with the final diagnosis as recorded 9 months later. This revealed that the testing had resulted in a single correct diagnosis in 73 percent of patients; where it resulted in more than one possible diagnosis, one of them was ultimately confirmed as correct in another 18 percent of patients, to yield an overall diagnostic accuracy of 91 percent. The electrophysiologic diagnosis was unsuspected by the referring physician, regardless of his or her specialty, in approximately one-third of cases.
Over the years, the activity of individual muscles in the maintenance of posture and during normal or abnormal movement has also been studied by EMG. Such studies are of considerable academic interest, and their clinical relevance is considered further in Chapter 20 . The interested reader is also referred to a glossary of terms commonly used in electromyography.
Practical aspects
The electrical activity of muscle is studied for diagnostic purposes by inserting a recording electrode directly into the muscle to be examined. The bioelectric potentials that are picked up by this electrode are amplified, then displayed on a cathode ray oscilloscope for visual analysis and fed through a loudspeaker system so that they can be monitored acoustically. A permanent photographic record of the oscilloscope trace can be made, if desired, or the amplified bioelectric signals can be recorded for retrieval at a later date. Modern, commercially available equipment includes analog-to-digital converters that permit the easy storage of data, advanced signal processing and analysis, and alteration in the display characteristics (e.g., time base and sensitivity) of stored potentials. Signal averaging is possible, and voltage trigger and delay lines facilitate the viewing of potentials.
The concentric needle electrode is a convenient recording electrode for clinical purposes. It consists of a pointed steel cannula within which runs a fine silver, steel, or platinum wire that is insulated except at its tip. The potential difference between the outer cannula and the inner wire is recorded, and the patient is grounded by a separate surface electrode. Alternatively, a monopolar needle electrode can be used. This consists of a solid needle (usually of stainless steel) that is insulated, except at its tip. The potential difference is measured between the tip of the needle, which is inserted into the muscle to be studied, and a reference electrode (e.g., a conductive plate attached to the skin or a needle inserted subcutaneously). The pick-up area of the concentric needle electrode is smaller than that of the monopolar electrode, and it is asymmetric as opposed to the more circular pick-up area of the monopolar electrode. Both concentric and monopolar electrodes are available in disposable or reusable forms. There is no evidence that one type of electrode is more painful to patients than the other.
An electrode exhibits some opposition or impedance to the flow of an electric current, and it is therefore important that it is connected to an amplifier having a relatively high input impedance to prevent loss of the signal. The amplifier, and the recording system to which it is connected, should have a frequency response of 2 or 20 to 10,000 Hz so that signals within this frequency spectrum are amplified uniformly without distortion. When necessary, however, the frequency response of the amplifier can be altered by the use of filters. This allows the attenuation of noise or interference signals that have a frequency different from that of the potentials under study.
Noise, which appears as a random fluctuation of the baseline, is generated within the amplifier and by movement of the recording electrodes or their leads. It can obscure the bioelectric signals to be studied, as can any unwanted interference signals that are picked up by the recording apparatus. Interference signals usually are generated by the alternating-current (AC) power line, by appliances such as radios, or by paging systems; occasionally, however, they are biologic in origin. Technical and safety factors are important when the electrical activity of muscle is to be recorded. They are considered in detail in Chapter 2 and the review by Gitter and Stolov.
Procedure
The patient is examined in a warm, quiet room. The time base of the oscilloscope is allowed to sweep freely from left to right with a speed of 10 msec/cm, or with a slower sweep speed when firing patterns are to be characterized. The gain is set commonly at 50 or 100 μV/cm for examining insertion and spontaneous activity, and at 200 or 500 μV/cm for studying motor unit action potentials. Appropriate filter settings were discussed earlier. Proper grounding is essential, as discussed in Chapter 2 . Muscles are selected for examination on the basis of the patient’s symptoms and signs, and the diagnostic problem that they raise. A ground lead is attached to the same limb as the muscles that are to be examined. The skin overlying the relaxed muscle to be examined is cleaned with an alcohol swab, allowed to dry, and then held taut while the needle electrode is inserted into the muscle. The presence and extent of any insertion activity are noted. The muscle is then explored systematically with the electrode for the presence of any spontaneous activity. Following this, the parameters of individual motor unit action potentials are defined in different sites during graded muscle contraction, attention being directed not only to the shape and dimensions of the potentials, but also to their initial firing rate and the rate at which they must fire before additional units are recruited. Finally, the interference pattern is compared with the strength of contraction during increasingly powerful contractions, until full voluntary power is being exerted.
Needle EMG is an invasive procedure, and concern has increased about infective complications, involving both patients and electromyographers, especially as regards human immunodeficiency virus (HIV), hepatitis virus, or Creutzfeldt–Jakob disease. It is wise for the physician to wear latex or rubber gloves during the examination and then to discard them in an appropriate receptacle once the procedure is over. Disposable needle electrodes are preferable for patient safety; they must be handled with care and disposed of in proper containers after use. Needles should not be inserted through an infected space.
Other complications are mild or rare. Pain at the site of needle insertion sometimes lasts for 1 or 2 days, and bruising may occur at the site of needle insertion. Pneumothorax is a rare complication of diaphragmatic EMG or of examination of the chest wall, supraspinatus, or cervical or thoracic paraspinal muscles. One patient is reported to have developed an acute compartment syndrome of the leg following needle EMG, probably because of puncture of a small blood vessel by the examining electrode; this necessitated a surgical release procedure. The needle examination carries a small but definite risk of hemorrhagic complications in patients with acquired or inherited coagulopathies and in those patients taking anticoagulants or even antiplatelet agents. When it is required, nevertheless, small electrodes should be used; the advice of a hematologist should be obtained in severe cases. Certain over-the-counter herbal remedies, such as ginkgo biloba and ginseng, may also increase the risk of bleeding. Rare reports of needle electrodes breaking off within a muscle and requiring minor surgery for retrieval have been published.
Electrical activity of normal muscle
In clinical EMG, electrical activity is recorded extracellularly from muscle fibers embedded in tissue, which is itself a conducting medium. The action potentials that are recorded in this way have a tri- or biphasic configuration. The basis for this is illustrated schematically in Figure 11-1 , where the active electrode is shown on the surface of a fiber and the reference electrode is placed at a remote point in the conducting medium. Because there is a flow of current into the fiber (i.e., a current “sink”) at the point of excitation, and an outward flow of current in adjacent regions, the propagated impulse can be considered a moving sink of current, preceded and followed by current sources. Accordingly, when an impulse travels toward the active electrode, this electrode becomes relatively more positive as it comes to overlie the current source preceding the action potential ( Fig. 11-1, A ). A short time later, as the impulse itself arrives, the active electrode registers a negative potential in relation to the distant electrode ( Fig. 11-1, B ), and then a relatively positive potential as the impulse passes on and is followed by the current source behind it ( Fig. 11-1, C ). As this too passes on, the electrode comes again to be on a resting portion of the fiber and the potential between the two electrodes returns to the baseline ( Fig. 11-1, D ). Clearly, the recorded action potential will be biphasic with a negative onset (rather than triphasic with a positive onset) if the active electrode is placed over the region of the fiber where the impulse is initiated.

EMG Activity at Rest
EMG activity usually cannot be recorded outside of the endplate region of healthy muscle at rest, except immediately after insertion or movement of the needle recording electrode. The activity related to electrode movement (i.e., insertion activity) is caused by mechanical stimulation or injury of the muscle fibers and usually stops within about 2 seconds of the movement ( Fig. 11-2 ). After cessation of this activity, spontaneous activity may be found in the endplate region but not elsewhere. This endplate noise, as it is called, consists of monophasic negative potentials that have an irregular, high-frequency discharge pattern; a duration of between 0.5 and 2 msec; and an amplitude that is usually less than 100 μV. The potentials correspond to the miniature endplate potentials that can be recorded with microelectrodes in animals. Biphasic potentials with a negative onset are also a constituent of endplate noise and have a duration of 3 to 5 msec and an amplitude of 100 to 200 μV. They have been held to represent muscle fiber action potentials arising sporadically because of spontaneous activity at the neuromuscular junction or activity in intramuscular nerve fibers.

EMG Findings During Activity
Motor Unit Action Potentials
Excitation of a single lower motor neuron normally leads to the activation of all of the muscle fibers that it innervates (i.e., those that constitute the motor unit). The motor unit action potential is a compound potential representing the sum of the individual action potentials generated in the few muscle fibers of the unit that are within the pick-up range of the recording electrode. Its shape depends on the synchrony of firing of those few muscle fibers. When recorded with a needle electrode, motor unit action potentials are usually bi- or triphasic in shape ( Fig. 11-3 ), but in the limb muscles about 12 percent may have five or more phases and then are described as polyphasic; the actual percentage will vary with the muscle and the age of the patient. The number of phases is calculated by adding 1 to the number of times that the baseline is crossed. The total duration of the potentials (i.e., the time taken for the trace to return finally to the baseline after its initial deflection at the beginning of a potential) is normally between 2 and 15 msec, depending on the muscle being examined. The precise value relates to the anatomic scatter of endplates of the muscle fibers in the units under study that are within the pick-up zone of the recording electrode. This is because of the different distances along which the muscle fiber action potentials will have to be conducted from the individual endplates to the recording zone of the electrode. The amplitude of the individual motor unit action potentials is measured between the greatest positive and the greatest negative deflections of the potentials. When recorded by a concentric needle electrode, it is usually between 200 μV and 3 mV; this is determined largely by the distance between the recording electrode and the active fibers that are closest to it, and by the recruitment order. The number of active fibers lying close to the electrode and the temporal dispersion of their individual action potentials also affect the amplitude of the potentials but to a lesser extent. Computer simulations of motor unit action potentials have suggested that amplitude is determined by less than eight fibers situated within 0.5 mm of the electrode.

Other features of individual motor unit action potentials that merit consideration are the rise time, area, and stability. The rise time of the negative spike is the interval between the onset and the peak of this component of the motor unit action potential. It reflects the distance between the recording electrode and the muscle fibers that generate the spike, and becomes shorter as this distance is reduced. A rise time of approximately 200 μsec or less is necessary if the other characteristics of the potentials are to be evaluated usefully. The area of the negative spike depends on the number and diameter of muscle fibers closest to the recording electrode, and the temporal dispersion of their action potentials. The area of the entire potential provides similar information, but for all of the muscle fibers contributing to the potential. An individual motor unit action potential should show no change in its shape or amplitude in the absence of electrode movement. This stability is lost in certain contexts, however, and especially with disorders affecting neuromuscular transmission, when variability of the potentials is seen.
Physiologic Factors Influencing Motor Unit Action Potentials
The configuration and dimensions of individual motor unit action potentials are normally constant, provided that the recording electrode is not moved. They are, however, influenced by the characteristics of the recording electrode and apparatus in the electromyograph system; and by physiologic factors such as patient age, intramuscular temperature, the site of the recording electrode within the muscle, and the particular muscle under examination. The potentials recorded with concentric needle electrodes tend to have slightly lower amplitudes and shorter durations than those recorded with monopolar needle electrodes.
With increasing age from infancy to adulthood there is an increase in the mean duration of motor unit action potentials in limb muscles, probably because of growth in width of the territory over which endplates are scattered. Later increases in duration relate to increasing fiber density within motor units as a result of the reduction in muscle volume that occurs in older subjects. Mean duration of motor unit potentials and the number of polyphasic potentials also increase as temperature declines. Abnormalities in the parameters of motor unit action potentials occur in neuromuscular diseases, as discussed later.
Motor Unit Recruitment Pattern
When a muscle is contracted weakly, a few of its motor units begin firing at a low rate. As the force of contraction increases, the firing rate of these active units increases until it reaches a certain frequency, when additional units are recruited. The frequency at which a particular unit must fire before another is recruited (i.e., the recruitment frequency) depends on the muscle and motor unit being studied and on the number of units capable of firing and the tension that they can generate, but it is usually between 5 and 20 times per second. The ratio of the number of active motor units to the firing frequency of individual units is generally less than 5 and is relatively constant for individual muscles. Thus, with a firing frequency of 20, the number of active units will be 4 or more. In general, the units recruited first are smaller in amplitude than those recruited later.
With increasing muscle contraction, so many units are eventually active that the baseline is interrupted continuously by the potentials, and individual potentials cannot be distinguished from each other. The resulting appearance of the oscilloscope trace is referred to as the interference pattern ( Fig. 11-4 ).

Abnormalities of recruitment pattern are discussed on page 243 .
EMG activity in pathologic states
EMG Activity at Rest
Insertion Activity
Insertion activity is found only when some muscle tissue remains viable and is therefore absent in the advanced stages of various neuromuscular disorders when muscle has atrophied completely, to be replaced by adipose or connective tissue. It is also reduced or absent when the needle electrode is not situated in muscle, and in certain metabolic disorders (e.g., during the paralysis of hypokalemic periodic paralysis). Insertion activity is prolonged in denervated muscle and may be the first electrophysiologic sign of denervation, occurring within a few days of an acute neurogenic lesion and preceding the development of fibrillation potentials. It is also prolonged in polymyositis, the myotonic disorders, and some of the other myopathies; in these instances, it consists of repetitively firing fibrillation potentials and positive sharp waves. In myotonic disorders, waxing and waning myotonic discharges typically are found ( p. 240 ). Insertion activity consisting of an irregular discharge of variable duration that “snaps, crackles, and pops” and follows normal insertion activity has been described, but it has no particular pathologic significance.
After cessation of all insertion activity, various types of spontaneous activity may be recorded from fully relaxed muscle in patients with a neuromuscular disorder.
Fibrillation Potentials and Positive Waves
Fibrillation potentials are action potentials that arise spontaneously from single muscle fibers. When they occur rhythmically, their genesis may relate to oscillations of the resting membrane potential of denervated skeletal muscle fibers. Less commonly, they occur irregularly, perhaps relating to random, discrete, spontaneous depolarizations that originate in the transverse tubular system of the muscle fiber. They may occur as spikes or as positive waves. Their density provides a guide to the number of denervated muscle fibers and usually is determined subjectively on a scale of 1+ (a few fibrillations in most areas) to 4+ (profuse fibrillations in all areas, filling the oscilloscope screen)
Spike Form of Fibrillation Potentials
Fibrillation potentials usually have an amplitude of between 20 and 300 μV, a duration of less than 5 msec, and a firing rate of between 2 and 20 Hz ( Fig. 11-5 ). Their amplitude relates to the diameter of type 1 muscle fibers. They have a bi- or triphasic shape, the first phase being positive, except when the potentials are recorded in the endplate region. This positive onset facilitates their distinction from endplate noise. Over the loudspeaker, they give rise to a high-pitched repetitive click, which aids their detection. They are found in denervated muscle, provided that some tissue remains viable and the muscle is warm when examined; however, they may not appear for 3 to 5 weeks after an acute neuropathic lesion. Once present, they may persist for months or even years, until the muscle fibers have come to be reinnervated or have degenerated. They are not in themselves diagnostic of denervation, however, because they are also seen in primary muscle diseases such as polymyositis, inclusion body myositis, and muscular dystrophy, and in patients with botulism, trichinosis, muscle trauma, or metabolic disorders such as acid maltase deficiency or hyperkalemic periodic paralysis. The presence of fibrillation potentials in myopathic disorders probably relates to isolation of part of the muscle fibers from their endplates, so that they are denervated functionally. Scanty fibrillation potentials occasionally may be found in normal healthy muscle; pathologic significance therefore should not be attributed to them, unless they are detected in at least three separate sites within the muscle being examined.

Positive Waves as Fibrillation Potentials
Positive sharp waves usually are found in association with, and have the same clinical significance as, the spike form of fibrillation potentials. They occur both in denervated muscle and in certain primary disorders of muscle. Indeed, Nandedkar and colleagues have shown the transformation of a fibrillation potential to a positive sharp wave, and vice versa, reinforcing the concept that these potentials are two manifestations of the same phenomenon. They are thought to arise from single muscle fibers that have been injured. As viewed on the oscilloscope, they consist of an initial positive deflection, followed by a slow change of potential in a negative direction that may be extended into a small negative phase (see Fig. 11-5 ). Their amplitude is usually about the same as or slightly greater than that of the spike form of fibrillation potentials, their duration is often 10 msec or more, and their discharge rate is similar to fibrillation potentials but sometimes can reach values of up to 100 Hz. They may fire regularly or, less commonly, irregularly. Positive sharp waves may occur diffusely in the absence of the spike form of fibrillation, sometimes on a familial basis.
Fasciculation Potentials
Fasciculation potentials are similar to motor unit action potentials in their dimensions and have been attributed to spontaneous activation of the muscle fibers of individual motor units. They have an irregular firing pattern; an individual potential may occur at a rate that varies from several times per second to less than once per minute. Their detection is aided by the sudden dull “thump” that they produce over the loudspeaker. They may be found in normal persons or in patients with chronic partial denervation, particularly when this is caused by spinal cord pathology, and especially in amyotrophic lateral sclerosis. Benign fasciculations cannot be distinguished from those associated with neuromuscular disorders. Pathologic significance should therefore not be attributed to fasciculations, unless they are accompanied by other evidence of denervation (e.g., fibrillation potentials or changes in motor unit action potentials). Fasciculations also occur in hyperthyroidism, in the cramp-fasciculation syndrome, and with anticholinesterase agents.
In patients with anterior horn involvement, fasciculation potentials can arise at multiple sites along motor axons or the somas of diseased motor neurons; there can be an exclusively distal, exclusively proximal, or a mixed origin, but origin on the distal extremity of the axon seems to be most common. Analysis of the firing pattern of fasciculations in amyotrophic lateral sclerosis using a high-density surface recording technique has shown that potentials of axonal and neuronal origin can be distinguished and that they may coexist in the same muscle.
The basis of fasciculation potentials remains uncertain and may vary in different circumstances. Although widely regarded as representing the activity of muscle fibers in individual motor units, the evidence is conflicting in motor neuron disease and amyotrophic lateral sclerosis.
Myotonic Discharges
Myotonic discharges consist of high-frequency trains of action potentials that are evoked by electrode movement or by percussion or contraction of the muscle; they are enhanced by cold and reduced by repeated contractions. The potentials resemble fibrillation potentials or positive sharp waves. Their frequency (20 to 100 Hz) and amplitude (10 μV to 1 mV) wax and wane ( Fig. 11-6 ), and in consequence the trains of potentials produce a sound like that of a dive-bomber on the loudspeaker. They are found in patients with one of the various myotonic disorders, most often in types 1 or 2 myotonic dystrophy (dystrophia myotonica, DM1 or DM2) or in myotonia congenita. They may also be found in hyperkalemic periodic paralysis, and occasionally are found in patients with polymyositis, the myopathy of acid maltase deficiency, chronic axonal polyneuropathies, or neurogenic muscle wasting from other causes, or who have received colchicine or diazocholesterol.

EMG evaluation of 51 patients with chloride, sodium, or calcium channel mutations known to cause myotonia or periodic paralysis has shown differences that generally matched the clinical symptoms. By combining the responses to different exercise tests, five electromyographic patterns were defined that correlated with subgroups of mutations. It is not yet clear whether such techniques can be refined to predict the causal gene reliably in all cases. There are also differences between DM1 and DM2 in the character of the myotonic discharges. In DM1, myotonic discharges wax and wane and their severity correlates with muscle weakness, whereas in DM2 they tend to be waning in character and there is no correlation with weakness. Again, it remains unclear whether such EMG differences can be used to distinguish reliably between these two types of myotonic dystrophy.
Myotonic discharges do not result simply from mechanical irritation because they can be recorded with surface electrodes, and they are not prevented by pharmacologic blockade of neuromuscular transmission. They apparently relate to a disorder of the muscle fiber membrane. Altered chloride channels have been incriminated in myotonia congenita, and altered regulation of sodium channels has been implicated in myotonic dystrophy. Disease of the sodium channels appears to be responsible in hyperkalemic periodic paralysis and paramyotonia congenita.
Complex Repetitive Discharges
Trains of high-frequency action potentials are sometimes found in the muscles of patients with muscular dystrophy, inflammatory myopathies, hypothyroid myopathy, or chronic partial denervation. They also occur in patients with metabolic disorders such as hyperkalemic periodic paralysis, hypothyroidism, or certain of the glycogen storage diseases, and are found occasionally in otherwise normal muscles, particularly the iliopsoas. They are often believed to indicate chronicity of the causal disorder, but this probably is not the case. The discharges occur spontaneously and after electrode movement or voluntary contraction. They have a regular firing pattern and an abrupt onset and termination, but unlike myotonic discharges, their amplitude and frequency remain constant (see Fig. 11-6 ). The individual action potentials are often polyphasic. Their origin is uncertain, but they probably are initiated by a fibrillating muscle fiber which, by ephaptic transmission, depolarizes one or more adjacent hyperexcitable muscle fibers, regardless of whether they are part of the same motor unit. The configuration of the discharges depends on the synchrony of firing of the fibers that contribute to the discharges.
Motor Unit Action Potentials
Motor unit activity may occur spontaneously and repetitively, despite full voluntary relaxation of the muscle, in patients with myokymia, muscle cramps, or tetany. Individual action potentials sometimes exhibit a rhythmic, grouped pattern of firing so that double, triple, or multiple discharges are seen ( Fig. 11-7 ). Double discharges also sometimes occur at the beginning of a voluntary contraction in normal subjects and patients with disorders of the anterior horn cells, roots, or peripheral nerves.

Myokymic Discharges
The term myokymic discharges is used to refer to spontaneously occurring, grouped action potentials, each group being followed by a period of silence, with subsequent repetition of a grouped discharge of identical action potentials in a semirhythmic manner. The multiplets or grouped motor unit action potentials of tetany may resemble myokymic discharges but are under voluntary control.
Myokymic discharges may occur in the limbs of patients with radiation-induced plexopathy or, less commonly, patients with radiation myelopathy, multiple sclerosis, acute inflammatory polyradiculoneuropathy, chronic radiculopathy or entrapment neuropathy, syringomyelia, or gold intoxication. They also occur in Isaacs syndrome and Morvan syndrome. Myokymic discharges in facial muscles occur in some patients with multiple sclerosis, brainstem neoplasms, or polyradiculoneuropathy, or who have received radiation to the head and neck. The discharges are consistently unilateral when a brainstem neoplasm is responsible, may occur unilaterally or on both sides of the face at different times in multiple sclerosis, and may be bilateral in polyradiculoneuropathy.
The pathophysiologic basis of myokymic discharges is unclear, but suggested mechanisms include abnormal excitability of lower motor neurons or peripheral nerves; ephaptic excitation; and the development of a rhythmic, oscillating, intra-axonal generator of action potentials. It has been suggested that membrane “bistability” of alpha motor neurons is sometimes responsible, the cell membrane having two equilibrium potentials, one at the resting potential and a higher one above threshold, generating maintained rhythmic firing.
Cramp Discharges
Cramp discharges consist of the involuntary repetitive firing at high rates (up to 150 Hz) of motor unit action potentials in a large area of muscles, usually associated with painful contraction of the muscle. The discharges typically have an abrupt onset. The number of motor unit action potentials and their discharge frequency increase gradually and then decline as the muscle contraction ceases. Cramps commonly occur in normal people but may also occur with chronic neurogenic disorders, various metabolic disturbances, and in the cramp-fasciculation syndrome.
Neuromyotonic Discharges
Neuromyotonic discharges consist of irregular bursts of high-frequency (up to 300 Hz) discharges of motor unit action potentials as doublets, triplets, or multiplets. The discharges often have an abrupt onset and termination and usually last for a few seconds. Some of the action potentials are very short in duration. The amplitude of the discharges typically wanes. The discharges occur spontaneously or with needle movement, activity, ischemia, or percussion over the nerve, and they do not disappear during sleep. They are abolished by curare. In many instances, the discharges also can be abolished by proximal nerve block, whereas the extent to which more distal nerve block stops the discharges is variable, suggesting that the hyperexcitability sometimes arises in the distal nerve trunk. Neuromyotonic discharges may occur in patients with peripheral neuropathy of the axonal or demyelinative type (e.g., in chronic acquired demyelinating polyneuropathy or multifocal motor neuropathy), and on either a sporadic or a hereditary basis in patients with muscle stiffness and other neuromuscular symptoms (see p. 254 ) but no evidence of neuropathy (Isaacs syndrome). They have also been described in patients with myasthenia gravis, raised titers of acetylcholine receptor antibodies, or thymoma, with anticholinesterase poisoning, and in those receiving penicillamine. At least in some instances, they appear to relate to the presence of autoantibodies that reduce the number of functional voltage-gated potassium channels and thus lead to hyperexcitability of motor nerves and increased release of acetylcholine; other antigenic targets may also be involved.
A form of these discharges, designated neurotonic discharges , occurs with mechanical irritation of nerves at operation. They consist of brief high-frequency discharges of motor unit action potentials. Their recognition is helpful in warning surgeons of possible nerve damage.
EMG Findings During Activity
Motor Unit Action Potentials
Any change in the number of functional muscle fibers contained in motor units will affect the parameters of motor unit action potentials. This is exemplified best by the character of the action potentials that are recorded from a muscle when the number of muscle fibers per unit is reduced. The mean duration of the action potentials is shortened because of the loss of some of the distant fibers that previously contributed to their initial and terminal portions, whereas their mean amplitude is reduced because of the loss of some of the fibers lying close to the electrode. If the spikes generated by the surviving muscle fibers of individual units are separated widely in time, there may also be an increased incidence of polyphasic potentials. As might be expected, therefore, the mean duration and amplitude of motor unit action potentials are reduced in patients with myopathic disorders (see Fig. 11-3 ), and there is an increased incidence of polyphasic potentials. This temporal dispersion of spikes may relate to scatter of endplate regions within surviving muscle fibers, variation in diameter of the muscle fibers (so that the conduction velocity in the fibers is more varied), longitudinal fiber splitting, regeneration of muscle fibers, or some combination of these factors.
Similar findings may also be encountered in patients with disorders of neuromuscular transmission (e.g., myasthenia gravis or Lambert–Eaton myasthenic syndrome) and during the reinnervation of muscle following severe peripheral nerve injury; however, the characteristic feature in such circumstances is the variability that the action potentials exhibit in their parameters, and especially in their amplitude, during continued activity ( Fig. 11-8 ). A similar variability in amplitude and morphology of motor unit action potentials may be encountered in amyotrophic lateral sclerosis and progressive muscular atrophy, when it signifies active disease and thus a poor prognosis.

In neuropathic disorders , it is the number of functional motor units that is reduced, and the average number of muscle fibers per unit actually may be increased if denervated muscle fibers are reinnervated by collateral branches from the nerve fibers of surviving units. The motor unit action potentials recorded in such circumstances are of longer duration than normal and may be polyphasic (see Fig. 11-3 ). This is because the activity recorded by the electrode is temporally more dispersed than normal, caused primarily by the greater anatomic scatter of endplates in the units but also by the reduced conduction velocity at which immature collateral branches conduct impulses. The action potentials may also have a greater amplitude than normal if the number of muscle fibers lying close to the electrode is increased; such potentials are often particularly conspicuous in patients with involvement of anterior horn cells in the cord.
Polyphasic motor unit action potentials are found in limited numbers in normal muscle, and care must be exercised in attaching any pathologic significance to them, unless they are present in excessive numbers and have abnormal dimensions. Low-amplitude, short-duration polyphasic potentials are seen most characteristically in myopathies and myositis, but are also found during early reinnervation after axonal loss and in disorders of neuromuscular transmission. Similarly, large-amplitude, long-duration polyphasic potentials are typical of disorders characterized by chronic partial denervation with reinnervation (e.g., motor neuron diseases), but may also occur in polymyositis and muscular dystrophies.
Motor unit action potentials sometimes are followed by smaller potentials called satellite potentials . These can appear at any point after the termination of the motor unit action potential. Such potentials sometimes occur after an interval of about 15 to 25 msec or more (see Fig. 11-3 ). These late components may occur in both neurogenic disorders and muscle diseases, such as inflammatory myopathies and muscular dystrophy. They can be explained by the presence of an ectopic endplate or by delayed conduction along unmyelinated collateral nerve sprouts innervating previously denervated muscle fibers; however, in the latter case a change in the latency of the late component would be expected to occur with sprout myelination. In muscular dystrophy, the denervated fibers probably arise by segmentation of existing muscle fibers or by muscle regeneration.
Abnormalities of Recruitment Pattern
When the number of functional motor units is reduced, as in patients with neurogenic weakness , there may be a diminution in the density of electrical activity that can be recorded from affected muscles during a maximal voluntary contraction. In severe cases, it may be possible to recognize individual motor unit action potentials ( Fig. 11-9 ). In such circumstances, there is an increase in the rate at which individual units begin to fire and also in the rate at which they must fire before additional units are recruited (i.e., in the recruitment frequency), which, as indicated earlier, is influenced by a number of variables but is usually between 5 and 20 Hz. Firing rates as high as 50 Hz sometimes are encountered.

Reduced recruitment may be the sole EMG abnormality in patients with neurapraxia causing focal conduction block in a peripheral nerve and in patients with an acute axonal lesion if the examination is undertaken early, before fibrillation potentials have had time to develop.
In patients with myopathic disorders , the number of functional units remains unchanged until an advanced stage of the disorder, and therefore the interference pattern remains full. Indeed, it may be more complete than normal for a given degree of voluntary activity because there is an increase in the number of units activated to compensate for the reduced tension that individual units (with their reduced fiber content) are able to generate. Motor unit firing rates tend toward normal unless the myopathy is severe, when the frequency of firing at onset and at recruitment of other units may be increased. In advanced myopathies, however, recruitment is reduced when entire motor units have been lost because of involvement of all of their muscle fibers by the disease process.
When patients are unable to cooperate fully because of limited comprehension or pain, or for psychologic reasons, the interference pattern may be reduced during maximal voluntary effort because only a few units are activated and they fire at low rates (e.g., 10 Hz). The motor unit action potentials, however, are normal in configuration. The distinction of poor activation from the poor recruitment of neurogenic disorders is best made by the rate of firing of individual motor unit action potentials (slow in poor activation and rapid in poor recruitment) and by the ratio of the number of active motor units to the firing frequency of individual units (which is generally less than 5 and is relatively constant for individual muscles).
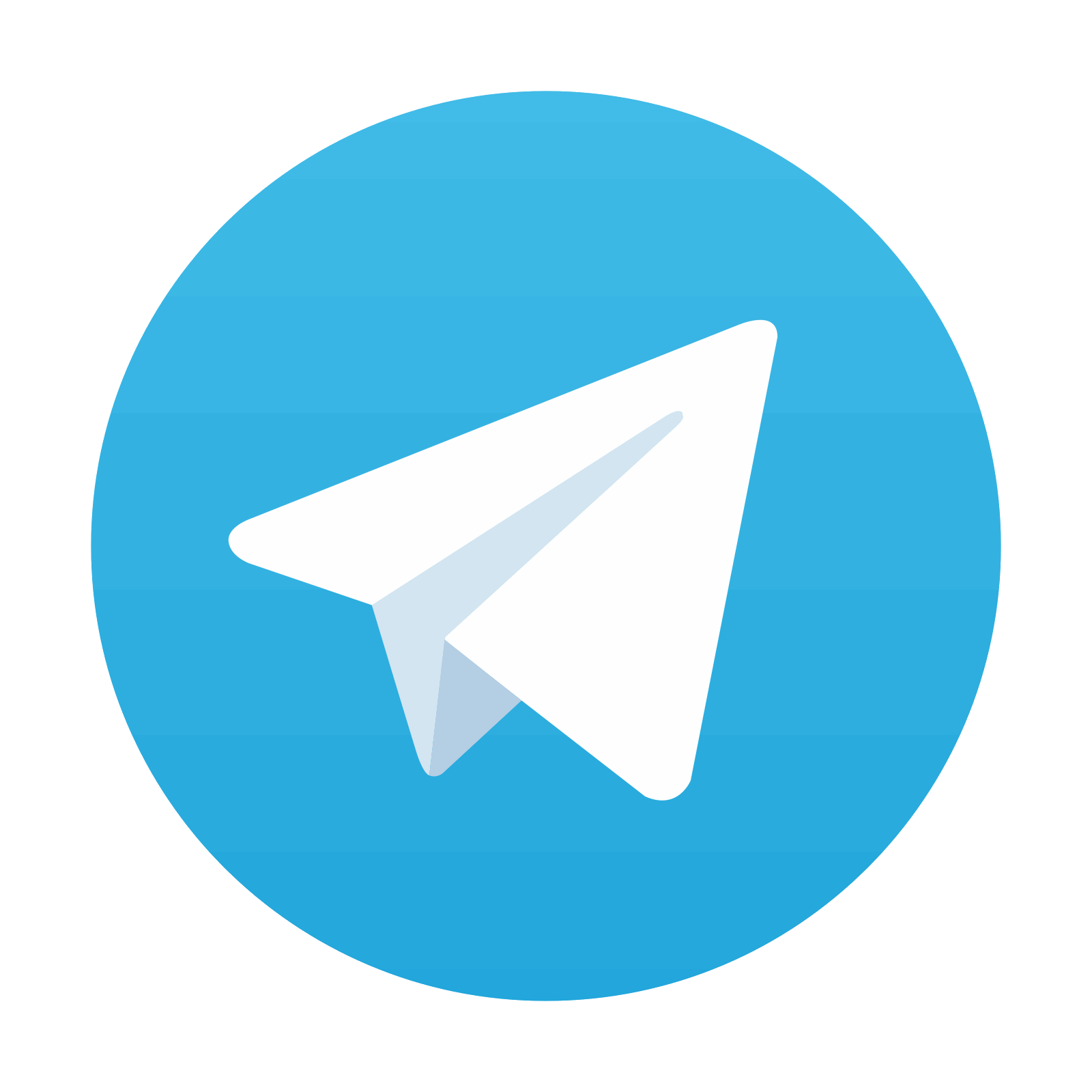
Stay updated, free articles. Join our Telegram channel

Full access? Get Clinical Tree
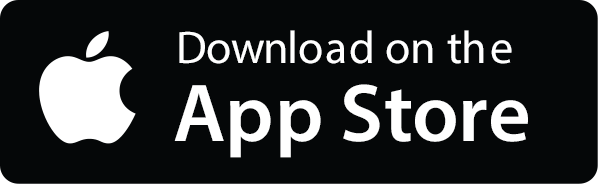
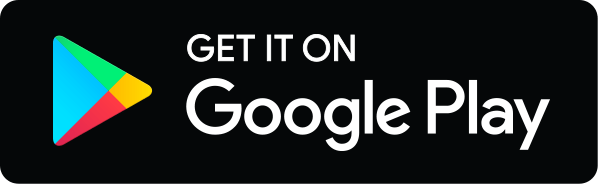