Abstract
Deep brain stimulation (DBS) has long been established as a safe and effective treatment for the management of advanced Parkinson’s disease (PD). Due to the success of DBS in the treatment of motor symptoms of advanced PD, this therapy is also considered an effective treatment in earlier stages of PD. This chapter provides an overview on the use of DBS surgery in PD, including patient selection criteria, surgical approaches, surgical target selection, side-effects of neuromodulation, and future applications.
Keywords
Deep brain stimulation, Outcomes, Parkinson’s disease, Patient selection, Stimulation programing, Technique
Introduction
Parkinson’s disease (PD) is a progressive neurodegenerative disorder characterized by the cardinal motor features: rest tremor, bradykinesia, rigidity, and loss of postural reflexes. The pathological hallmark of PD is degeneration of dopaminergic neurons in the substantia nigra pars compacta and the presence of alpha synuclein positive neuronal inclusions known as Lewy bodies. It is the second most common neurodegenerative disorder, following Alzheimer’s disease. In the United States over 60,000 individuals are diagnosed with PD every year and there are over 1 million individuals living with the disease ( ). As the size of our elderly population continues to increase, it is expected that the economic burden of chronic diseases such as PD will continue to rise. Given that we have no current treatments to prevent, cure, or slow the progression of PD, it is imperative that we provide treatments to help improve the quality of life of sufferers ( ).
The mainstay of medical therapy is aimed at increasing the availability of striatal dopamine, resulting in symptomatic improvement in most patients with PD. However, the response to dopamine therapy becomes less reliable and predictable over time. After approximately 5 years of therapy, medication-related motor complications develop in most patients with PD, including dyskinesia, wearing off, and “on–off” motor fluctuations characterized by a sudden, unpredictable loss of treatment effect ( ).
Deep Brain Stimulation in Parkinson’s Disease
Deep brain stimulation (DBS) is an established safe and effective surgical alternative for patients with PD who either develop medication-induced side-effects or motor fluctuations. Traditionally DBS has been considered a therapy for advanced PD and has been limited to patients no longer receiving adequate relief of their symptoms; in published studies the mean age of patients who underwent DBS was 58.6 years with mean duration of disease of 12 years ( ). Many clinical trials have demonstrated the effectiveness of DBS therapy in advanced PD ( ). Collectively these trials showed the benefit of neurostimulation in reducing the duration and severity of motor symptoms during the “off” state, providing more consistent and longer “on” time as well as reducing the severity of dyskinesias during the “on” state. Given its effectiveness in advanced PD, DBS has also been considered for the treatment of patients in the earlier stages of the disease. The EARLYSTIM trial assessed the benefit of subthalamic DBS compared to medical treatment in 251 PD patients with mean disease duration of 7.5 years and mean age of 52.4 years. After 2 years subthalamic DBS was found to be superior to medical therapy, with improvements observed in quality of life and motor disability ( ). In 2016, based on the results of the EARLYSTIM trial, the United States Food and Drug Administration expanded the indication for DBS therapy to include patients with PD duration of at least 4 years and at least 4 months of motor complications. It has further been hypothesized that early treatment with DBS might slow the progression of PD, as animal models have shown upregulation of brain-derived neurotrophic growth factor (BDNF) in the substania nigra when the subthalamic nucleus is stimulated ( ). This increased production of BDNF may be neuroprotective and therapeutic.
Selection Criteria for DBS in PD
Patients being considered for DBS should be evaluated for surgical candidacy based on their medical history and PD symptoms, including tremor, dyskinesias, gait, and postural instability. Neurosurgery consistently benefits only patients with idiopathic PD. Atypical PD or “Parkinson plus” syndromes (supranuclear palsy, striatonigral degeneration, etc.) or other disorders with Parkinsonian features have not responded favorably to surgery ( ). The following are presurgical selection criteria for PD patients being considered for DBS.
- 1.
Idiopathic PD with a historically good response to antiParkinsonian medications.
- 2.
Surgery is most likely to benefit tremor, rigidity, bradykinesia, dystonia, motor fluctuations, and drug-induced dyskinesia.
- 3.
Midline symptoms that improve with antiParkinsonian medication also improve with DBS, but those symptoms that do not respond to antiParkinsonian medication generally do not respond to DBS, i.e., postural instability, gait, and speech.
- 4.
The best indicator of the potential benefit achieved with DBS is the levodopa response: patients who demonstrate a significant improvement in the motor subscore (III) of the Unified Parkinson’s Disease Rating Scale (UPDRS) during “off” versus “on” levodopa medication state are most likely to benefit from surgery.
- 5.
The cutoff number is arbitrary, and is in the range of 25%–30% improvement in the on-UPDRS score as compared to off-UPDRS score. Patients with severe medication refractory tremor or asymmetric disease may be exceptions to this rule. Decisions must be made on a case-by-case basis.
- 6.
Patients having had magnetic resonance imaging (MRI) within 2 years that is not suggestive of alternative causes for PD motor symptoms. Atrophy and white-matter disease are concerns, but do not rule out a patient with a good levodopa response.
Target Selection and Surgical Technique
The two most common targets for DBS are the subthalamic nucleus (STN) and globus pallidus (GPi). Rarely the ventral intermediate nucleus (VIM) is also targeted in tremor-dominant PD. Although both STN and GPi are equally efficacious, the choice of one over the other can be tailored based on patient characteristics and goals for surgical therapy. In general, STN is the target of choice due to ease of electrophysiological detection, easier programming, ability to improve tremor in addition to rigidity and bradykinesia, and medication reduction. GPi is reserved for patients with risk for cognitive decline, significant dyskinesias, axial symptoms, and gait difficulty. VIM is strictly reserved for patients with predominant tremor and mild rigidity and bradykinesia. It is important to note that VIM DBS does not improve motor fluctuations and dose failures, so patients at risk for development of these should be considered for STN DBS.
Treatment Planning: Indirect and Direct Targeting
The stereotactic targets (STN, GPi, and VIM) are not visible on routine structural imaging ( ). Thus high-resolution specialized sequences are required to improve visualization (e.g., T2 fast-spin echo and proton-density-weighted images) and aid DBS planning ( ). Susceptibility-weighted imaging is used to improve contrast of STN borders ( ). Other sequences have also been specifically developed to improve target visualization for stereotactic surgery ( ). These imaging sequences, especially high-field (3 T and higher) imaging, also have artifacts ( ) and therefore a combination of formulaic, atlas-based, and direct targeting methods is often employed for stereotactic targeting. Images are preprocessed (coregistered, aligned to AC–PC plane) using a stealth workstation and stereotactic planning software (Framelink Medtronic Inc., Minneapolis, USA).
Targeting methods differ across centers ( ). In general the coordinates used for initial target selection in STN are X = 10–12 mm, Y = 2–5 mm posterior to midcommissural point or corresponding with anterior border of red nucleus, Z = 3–5 mm below the intercommissural plane; in GPi they are X = 19–21 mm, Y = 0–3 mm anterior to midcommissural point, Z = superior border of optic tract; and in VIM they are X = 10–14 mm or 11 mm lateral to the wall of third ventricle, Y = 5–7 mm anterior to posterior commissure, Z = at the intercommissural plane. The targets are then refined based on direct visualization of the target nucleus borders. Electronic brain atlases are also available to guide stereotactic targeting ( ), including deformable brain atlases ( ). It is important to note that both formulaic ( ) and atlas-based ( ) targeting have limitations in capturing the variability in individual anatomy. Thus most surgeons use microelectrode recording (MER) and stimulation for intraoperative confirmation ( ). Novel functional stereotactic atlases are being developed to incorporate these intraoperative findings to create probabilistic maps of physiological and functional information ( ).
Surgical Procedure: Awake and Asleep DBS
The typical deep brain stimulation surgery is performed in awake patients with local anesthesia and sedation as needed for surgical exposure ( ). The MERs are performed with patients awake for intraoperative testing. The recording electrode is advanced with a microdrive to record neuronal activity and create a map of different cell populations. In general, the cells within stereotactic targets are large amplitude and high frequency ( Fig. 74.1 ). The cells in the motor subdivisions of these nuclei are also responsive to both active and passive movements. Once the borders of the nucleus are traced, macrostimulation testing is performed to detect efficacy and understand the therapeutic window for side-effects. The trajectory for final DBS implantation can be modified based on intraoperative findings.

With improved resolution of imaging and intraoperative imaging guidance ( ), several experienced centers perform image-guided DBS implantation with patients under general anesthesia (“asleep DBS”). The commercial MRI-compatible platform for DBS surgery provides a workflow for stereotactic planning and intraoperative guidance ( ). The clinical outcomes from centers with significant experience in image-guided DBS placement are comparable to the MER-based targeting approach ( ). Some patients report anxiety while staying awake during neurosurgery, and asleep DBS may improve patient comfort and satisfaction ( ). Overall the accuracy of targeting is critical for good clinical outcomes ( ). The following steps are typically taken to minimize targeting error.
- 1.
Head positioning to avoid significant brain sag and pneumocephalus.
- 2.
Minimizing the egress of cerebrospinal fluid.
- 3.
Minimum manipulation once the recording system is in situ.
- 4.
Intraoperative imaging with either two-dimensional X-rays ( ), O-Arm (Medtronic, Inc.) ( ), or intraoperative computed tomography ( ).
Postoperative Complications
The safety profile of DBS is excellent, with a 1%–2% risk of serious postoperative complications ( ). The risk of other adverse events, including infection and hardware malfunction, is reported to be less than 10%. The majority of these complications occur within 1–3 months after surgery ( ), and these risks maybe diminished with improvements in the engineering of the implant ( ).
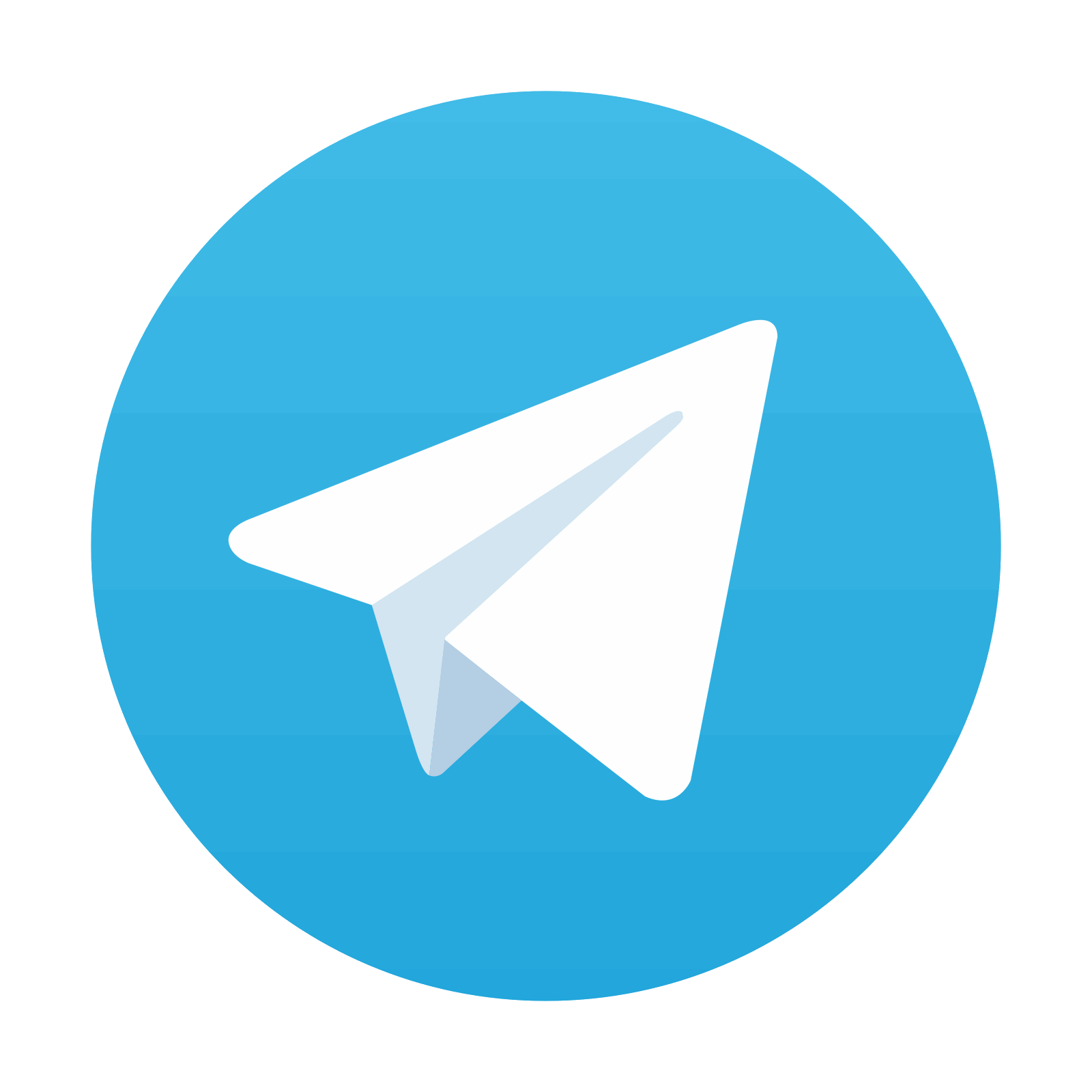
Stay updated, free articles. Join our Telegram channel

Full access? Get Clinical Tree
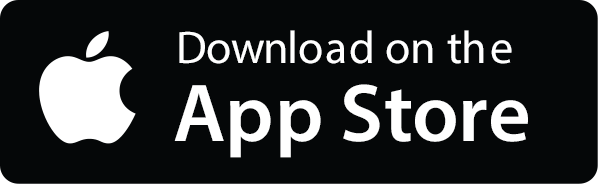
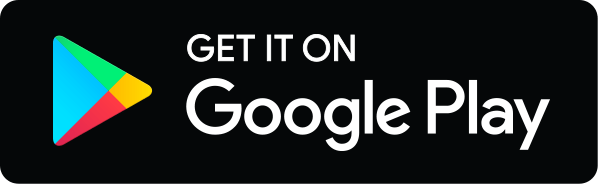