CHAPTER 318 Diagnosis and Management of Thoracic Spine Fractures
The thoracic spine between T1 and T10 is unique in that considerable stiffness and stability are conferred by the connecting ribs, sternum, and chest wall musculature. The clinical notion that thoracic spinal trauma requires high-velocity impact and tremendous force has been buttressed by biomechanical studies demonstrating that the rib cage alone augments the spine’s load-bearing capacity by twofold to threefold.1 Nevertheless, this added osteoligamentous stabilization is offset by increased vulnerability to neurological deficits because the thoracic spine exhibits the narrowest canal-to-cord relationship and a relatively sparse blood supply to the spinal cord.2 Furthermore, the thoracic spinal cord, when compared with other spinal regions, has the poorest prognosis for functional recovery.
Historical Perspective
Surgical management of thoracic spine fractures before introduction of the Harrington internal fixation system in the 1960s was limited to laminectomy and often associated with neurological deterioration secondary to spinal cord manipulation or increased instability.3 However, the success of surgical treatment of thoracic fractures has grown in parallel with technologic advancements in spinal instrumentation. Krengel and colleagues were among the first to demonstrate that early stabilization plus decompression of incomplete thoracic spinal cord injuries was associated with improved neurological function.4 Further data have suggested that early internal fixation may decrease hospital stay, prevent bony deformity, and accelerate transfer to rehabilitation.5
The past 2 decades have witnessed a shift away from hook constructs as pedicle screw systems have been shown to be a safe, reliable, and biomechanically superior choice for internal fixation in the thoracic spine.6–8 The presumed clinical benefit of operative intervention continues to be the impetus behind surgical intervention despite a lack of class I evidence. As the emphasis on evidence-based clinical decision making continues, new methods of comparison have been championed to supplant the costly, lengthy, and often impractical randomized trial in the traumatic spine patient population. Recently, a novel parallel cohort study with blinded inclusion based on clinical equipoise was used to compare operative and nonoperative treatment strategies for traumatic lumbar and thoracic fractures; a trend toward better neurological recovery, although not reaching statistical significance, was found in the operative group.9
Epidemiology
Thoracic spine fractures are relatively rare in comparison to fractures of the cervical, lumbar, and thoracolumbar regions; in a large multicenter study, only 16% of spinal fractures occurred in the T1-10 region.10 A wedge compression fracture is the most common thoracic spine injury, and multiple noncontiguous fractures in the thoracic spine are not rare. Spinal cord injury accompanies about 10% of thoracic vertebral body injuries, as opposed to a 39% incidence of concurrent spinal cord injury seen with cervical injuries.11 Approximately 15% of all spinal cord injuries occur in the thoracic spinal cord, and 17% of upper thoracic spine injuries cause paraplegia.12–14
The midthoracic spine is the most rigid and consequently at increased risk for fracture; the upper thoracic and T10 levels are less frequently injured, as noted later. The high-energy impacts causing thoracic spine fractures can generate severe concomitant injuries, including dissection or rupture of the aorta, intra-abdominal solid organ injury, hemothorax, pulmonary contusion, esophageal perforation, thoracic duct injury with chylothorax, and tracheal injury (Fig. 318-1).15–17 Thoracic fracture-dislocations are very unstable and lead to complete paraplegia in 80% of patients; however, there have been several well-documented reports of neurologically intact patients in whom accompanying bilateral pedicular fractures caused autodecompression and spared the spinal cord.18
Anatomic and Biomechanical Considerations
The thoracic spine consists of 12 vertebral bodies aligned in a prominent kyphotic curvature. These load-bearing elements of the spinal column are 2 to 3 mm shorter in anterior body height than in posterior body height.19 This is the primary cause of the normal kyphotic curve. There is also a dorsal arch that consists of relatively small pedicles, transverse processes, articulating facets, broad laminae, and a downward-pointing spinous process. The center of gravity of an upright individual falls through the anterior body of T1, passes anterior to the thoracic spine, and falls through the midbody of T12 (Fig. 318-2).
The thoracic spine is connected to the rib cage by body and transverse process articulations to individual ribs. The rib cage restricts the motion of the thoracic spinal column—especially in extension, which is reduced by 70%. The effects of the rib cage on flexion and lateral rotation are less prominent. The rib cage also adds stiffness to the spine, and experimental data have demonstrated a fourfold increase in tolerance of the spinal column to compression with an intact rib cage. Stabilization of the thoracic spine by the rib cage results in greater force being required to disrupt the spinal column at these levels than in other regions of the spinal column.20
The net result of the aforementioned anatomic considerations is that the mean of the range of flexion and extension at each segment in the upper thoracic spine is 4 degrees whereas that of the midthoracic spine is 6 degrees. The range of lateral bending is 6 degrees of motion per segment, whereas the range of axial rotation is 8 to 9 degrees.21 The rigidity of the midportion of the thoracic spine apparently places the midthoracic levels at increased risk for fracture. The upper thoracic and T10 levels are the most infrequently injured levels of the thoracic spine because they are protected by the adjacent, flexible cervical and thoracolumbar junctions (Fig. 318-3).22
Spinal Instability
Clinical instability is loss of the ability of the spine to maintain its pattern of displacement under physiologic loads so that there is no initial or additional neurological deficit, no major deformity, and no incapacitating pain.23 Although this definition is very inclusive, it has rather limited utility to a clinician faced with an acutely injured patient.
Various authors have tried to define stability and then recommend treatment based on the presumed injury mechanism. In 1949, Nicoll attempted to define stable versus unstable fractures by using an anatomic classification based on analysis of a series of 166 fractures or fracture-dislocations of the thoracolumbar spine. In his view, the major determinant of stability was the integrity of the interspinous ligament.24
Holdsworth introduced the first modern classification of fractures and attempted to use radiography to identify specific patterns of spinal fractures associated with the development of early as well as late instability.25 This classification scheme is based on the two-column theory of spinal column stability and is essentially a biomechanical interpretation of the injury mechanisms determined by a review of plain radiography. The integrity of the posterior bony elements and the posterior ligamentous complex, including the interspinous and supraspinous ligaments and the ligamentum flavum, is the major determinant of stability. A flexion-rotation injury is the most unstable of spinal column injuries in this classification scheme because both the bony and ligamentous elements of the posterior spinal column are compromised. Holdsworth acknowledged that this system was flawed and that it did not predict the presence of an unstable subset of compression fractures.25
Disruption of the posterior ligamentous structures alone, as posited by Nicoll and Holdsworth, is an insufficient view of instability of the spinal column. Denis modified the two-column theory of Holdsworth by dividing the anterior column into an anterior and a middle column, the latter consisting of the posterior longitudinal ligament and the posterior third of the vertebral body (Fig. 318-4).26 He used computed tomography (CT) of the spinal column injury to establish the integrity of the middle column. The more common anterior wedge compression fracture is distinguished by continuity of the posterior cortical rim from one pedicle medially over the posterior vertebral body to the opposite pedicle. If what appears to be a compression fracture also features disruption of the posterior vertebral body, it is actually a burst fracture (Fig. 318-5). The integrity of the middle column became the basis of a new classification of spinal stability, with injury to the anterior and the middle or the posterior and the middle columns resulting in an “unstable” fracture.
The most comprehensive classification scheme was published in 1994 by Magerl and associates, whose initial report described 53 distinct fracture patterns.22 The classification system of Magerl is based on the mechanism of failure of the spinal column and is accepted, with modifications, as a research tool by members of the major spine societies. The main categories in the Magerl system are type A, compression injuries; type B, distraction injuries; and type C, multidirectional torque injuries with translation (Fig. 318-6).27 These types are ordered in terms of increasing severity of the resultant spinal column injury and subdivided into groups according to the injury mechanism. The division into three groups is based on the morphologic features of the injury and the severity of the injury as it progresses through both the types and the groups, the most stable being an A1 fracture and the least stable being C3. Although the original classification contains further subdivisions, the simplified system reported by Gertzbein stops at the 3-by-3 grid (Fig. 318-6).27
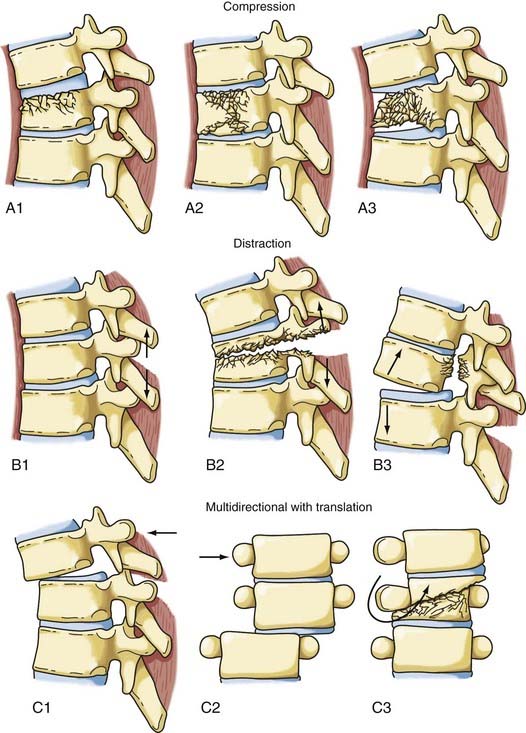
FIGURE 318-6 Fracture classification system as proposed by Magerl and coworkers and modified by Gertzbein. Fractures are divided into types according to major injury force: A1 to A3, compression; B1 to B3, distraction; and C1 to C3, multidirectional with translation (see text for details). The classification system is arranged in order of increasing severity: A1 is the most stable, whereas C3 is the least stable. The classification system can be used to determine the need for instrumentation and the optimal type of fixation. It represents a uniform method of classification of fractures for clinical research.22,27
Although perhaps useful in the research setting, the Magerl system has been criticized as being too complex for routine clinical use and has performed poorly in tests of interobserver agreement.28,29 Furthermore, although the Denis model remains popular, its original tenet—that instability ensues when two of the three columns are disrupted—has been recognized as an oversimplification that fails to incorporate the biomechanical importance of the spinal ligaments.
With spinal experts agreeing that most thoracolumbar fractures can be managed conservatively, the ideal classification system would be easy to apply, be highly reproducible, and assist in identifying the minority of patients who would benefit from operative intervention. This need for a practical thoracolumbar classification system directly linked to a clinical decision-making algorithm prompted the Spine Trauma Study Group to develop the thoracolumbar injury classification and severity score (TLICS).30,31 The TLICS scale is a point-based system founded on the triad of injury mechanism/morphology, integrity of the posterior ligamentous complex, and neurological status of the patient (Table 318-1).32 The total number of points is calculated for each thoracolumbar fracture based on these three major categories, and the final score is linked to an algorithm to help guide management: injuries with a total TLICS score of 3 or less should be considered for primary nonoperative management, fractures with a total TLICS score of 4 may be treated operatively or nonoperatively at the surgeon’s discretion, and injuries with a total TLICS score of 5 or higher should undergo primary operative management.30 Further studies have shown that TLICS exhibits excellent validity and interobserver reliability, unlike older classifications.33–35 By shifting emphasis away from the nebulous concept of spinal stability in favor of a quantitative assessment of operative necessity, the Spine Trauma Study Group has designed a seemingly robust classification that has thus far successfully met several stepwise validation challenges. Future studies will reveal whether the TLICS algorithm becomes widespread and whether the fracture score and its associated management recommendation are correlated with patient outcomes.
TABLE 318-1 Thoracolumbar Injury Classification and Severity Score
PARAMETER | POINTS |
---|---|
Morphology | |
Compression | 1 |
Burst | 2 |
Translational/rotational | 3 |
Distraction | 4 |
Neurological Status | |
Intact | 0 |
Nerve root injury | 2 |
Spinal Cord/Conus Medullaris Injury | |
Complete | 2 |
Incomplete | 3 |
Cauda equina | 3 |
Posterior Ligamentous Complex | |
Intact | 0 |
Indeterminate | 2 |
Disrupted | 3 |
The maximum score is 10. Treatment recommendation: total score of 3 or less, nonoperative treatment; total score of 4, surgeon discretion regarding nonoperative versus operative treatment; total score of 5 or higher, operative treatment.
Mechanism of Injury
Surgical therapy for an injured thoracic spine must take into account the injury force or forces that acted on the bony column and the pattern of instability associated with the specific injury. The forces responsible for a spinal column injury are generally accepted as compression, flexion, extension, rotation, shear, distraction, or a combination thereof.36 Magerl and colleagues formulated a classification system by dividing the pathomorphologic characteristics of the action of injury moments into three patterns: compressive, tensile/distractive, and axial torque, which correspond to the three injury types in the system—A, B, and C, respectively (Fig. 318-6).22
Compression or Axial Loading Fractures
The normal kyphosis of the upper thoracic spine results in an axial compression load being converted into an anterior flexion load on the vertebral body. The resultant fracture of the vertebral body is a wedge compression fracture; the same injury is produced by a flexion vector (Fig. 318-7).36 Magerl classified these fractures as types A1 to A3, depending on the injury pattern.
Flexion
Flexion and compression or axial loads produce an anterior force (flexion-compression)—the most common load—on the anterior vertebral body and a simultaneous tensile force on the posterior column. The injury produced is an anterior wedge fracture with an intact posterior vertebral body and therefore an intact middle column. This fracture is stable and not usually associated with spinal cord injury. If the anterior vertebral body is compressed in excess of 50%, posterior ligamentous disruption or facet joint failure often occurs and renders the injury unstable even in the absence of a fracture of the bony elements of the posterior column. A facet fracture or capsule injury can allow the superior body to sublux on the inferior body and thereby result in a fracture-dislocation. The posterior elements are injured more frequently than generally appreciated, and disruption of the posterior ligaments often occurs before failure of the vertebral body.37 Severe injuries that compromise the posterior vertebral body in addition to the anterior vertebral body result in a distinctive pattern of fracture in which the superomedial portion of the posterior vertebral body is fragmented from the remainder of the body; the fragment then rotates into the spinal canal and compromises the intracanalicular neural elements (Fig. 318-7, A3). Although the resultant burst fracture is a type of compression fracture, the Magerl system differentiates between a stable burst fracture, type A3, which is similar to the more common wedge compression fracture, and an unstable burst fracture, type B1 or C1. The latter types of injuries are often associated with a spinal cord injury and are prone to long-term instability if not surgically reconstructed.
Shear
Shear forces can be directed in any direction along the longitudinal axis of the spine (Fig. 318-8). Roaf demonstrated that shear force can produce severe ligamentous disruption similar to that generated by the combination of flexion and rotation considered earlier.38 Shear forces acting in a posterolateral direction cause the superior vertebral body to sublux anteriorly on the inferior vertebral body, with a complete spinal cord injury resulting from movement of the vertebral bodies. There have been occasions when anterior movement of the superior body results in disruption of the pars interarticularis or the pedicles, thereby “decompressing” the spinal cord and protecting it from serious injury.39 The more common result of this load is an anterior spondylolisthesis; however, the anterior body can be displaced posteriorly or laterally on the inferior body. The Magerl classification of this fracture pattern is type C1.
Flexion-Distraction
Although this type of injury was first described radiographically by Chance in 1948,40 the mechanism of injury was not defined until later.41 These injuries are commonly associated with the use of seat belts, especially lap belts, were once called seat belt injuries, but are more appropriately designated flexion-distraction injuries. Denis distinguished between “seat belt–type” injuries, of which the fracture described by Chance is only one, and fracture-distraction injury, which is a subtype of fracture-dislocation injury.26 Although the mechanism of injury is similar in both injuries, that is, a flexion movement produces a tensile force on the posterior and middle columns that acts on an axis of rotation and is translated anteriorly (by a seat belt holding the anterior abdominal wall to the seat), the latter injury is characterized by failure of the anterior column along with disruption of the anulus and stripping of the anterior longitudinal ligament from the inferior vertebral body. Loss of the anterior column allows anterior spondylolisthesis to develop. Although none of the fracture-dislocations of the flexion-distraction type occurred in the upper thoracic levels in the series reported by Denis, Eismont and associates stated that flexion-distraction can result in a bilateral facet dislocation in the thoracic spine.26,36 The fracture described by Chance is designated type B2 in the Magerl classification system, whereas fracture-distractions involving ligamentous disruption of the posterior column are designated type B1 (Fig. 318-9).22
Extension
The vector in this injury is the opposite of the flexion vector acting on the trunk, and it results in forced posterior movement. Although the majority of the injuries resulting from this force are isolated fractures of the posterior elements (facet, lamina, or spinous process), if a severe force is applied, the anterior longitudinal ligament and anulus will fail and result in a highly unstable shear fracture because retrolisthesis of the superior vertebral body on the inferior vertebral segment can occur. Denis and Burkus reported 12 of these types of fractures, 7 of which were in the upper thoracic spine, in a report after Denis’ original paper (Fig. 318-10).42 The Magerl classification of this fracture type is type B3.
Flexion-Rotation
This combination of injury vectors produces an extremely unstable injury of the vertebral column (Fig. 318-11). The flexion load causes an anterior vertebral body compression fracture, whereas the rotational load disrupts the facet joints by fracturing the superior facet on one side and tearing the facet capsule on the other. In addition, the anulus may fail, the posterior ligaments are disrupted, and the posterior vertebral body may fracture, with retropulsion of bone into the canal. The fracture-dislocation that results from this combination of load on the vertebral column was recognized by Holdsworth in his original paper as a highly unstable fracture that was “so constantly associated with paraplegia.”25 Magerl and colleagues classified this fracture as type C3, the most unstable fracture in their classification system.22
Initial Management of a Thoracic Spine Injury
After the patient is immobilized in the field with a collar and hard board and stabilized in accordance with the advanced trauma life support guidelines, the primary survey is conducted by the trauma team, including palpation of the entire posterior spinal axis to detect any bony step-off (and tenderness if the patient is responsive) during a logroll procedure to remove the hard board. In a modern trauma center, the initial thoracic spine imaging is typically generated as reconstructed images from thoracoabdominal CT scans in patients with a sufficient mechanism or clinical concern for spinal trauma. Any spinal fractures or bony displacement seen on such reconstructed CT images triggers neurosurgical consultation. Although trauma to the posterior ligamentous complex may be inferred from bony imaging findings such as splaying of the spinous processes on CT, MRI is performed for all thoracolumbar fractures as the preferred modality to assess the spinal ligaments.43 MRI is ordered on an emergency basis when the neurological examination reveals a focal motor or sensory deficit or if there is a history or signs of bowel/bladder dysfunction (e.g., elevated postvoid residual). The prevalence of wrong-level operations in the thoracic spine is significant,44 and we therefore insist on concomitant lumbar imaging so that one can count up from the sacrum to localize the thoracic pathology.
All patients with a neurological deficit from a spinal fracture undergo a physician-documented neurological examination based on the American Spinal Injury Association (ASIA) Standard Classification of Spinal Cord Injury Scale, which has very good interrater reliability.45,46 Although an ASIA score is documented on admission, many subsequent scores are obtained because evidence demonstrates that the first ASIA score with prognostic capability occurs at 72 hours after injury. The use of high-dose intravenous methylprednisolone for acute spinal cord injury is highly controversial, and many centers are moving away from the practice.47,48 An important consideration—which remains at the level of option—is that patients with an acute spinal cord injury should be monitored in the intensive care unit with a minimum mean arterial pressure goal of 85 to 90 mm Hg and adjunctive use of vasopressors to limit spinal cord hypoperfusion.49,50
Surgical versus Nonsurgical Management
Since the introduction of Harrington distraction rods, enthusiasm has been growing for surgical treatment of thoracic fractures. This is due in part to the ongoing introduction of effective internal fixation technology and the perceived clinical benefits of operative spinal stabilization, such as early mobilization of the patient. Another impetus for surgery is to prevent neurological deterioration, chronic pain, or the development of spinal deformity. Before the TLICS scale (see earlier), classification systems for thoracic and lumbar fractures provided minimal assistance in helping physicians decide between nonoperative and operative management. With surgeons clinging to the simple dictum that instability merits operative intervention, broad definitions of instability, such as that of White and Panjabi, have become popular ways to encompass the concept that surgery for thoracic and lumbar fractures not only immediately protects the neuraxis but also provides prophylactic protection from future deformity, pain, or deficit. As noted earlier, White and Panjabi defined clinical instability as loss of the ability of the spine to maintain its pattern of displacement under physiologic loads so that there is no initial or additional neurological deficit, no major deformity, and no incapacitating pain.23
The results reported by Frankel and associates remain the “gold standard” for nonoperative therapy to which alternative treatments and final outcomes are measured; in 371 patients with thoracic and lumbar fractures, there was only a 0.5% incidence of neurological worsening in those treated by postural reduction and recumbency.51 Similarly, excellent results with long-term follow-up are available for nonoperatively treated burst fractures of the thoracic and lumbar spine (primarily the thoracolumbar junction), with or without neurological deficit.52–57 However, other series have demonstrated a much higher rate of neurological worsening during nonoperative treatment, such as the 18% incidence reported by Denis and coauthors.58 Moreover, a thoracic or lumbar burst fracture—particularly one with a rotational component—has been associated with a higher risk for early neurological deterioration, even while patients are still hospitalized.59
Whether surgery for patients with a thoracic fracture and spinal cord injury can increase the likelihood of neurological improvement remains a matter of debate. Neurological improvement of incomplete spinal cord lesions after anterior or posterior surgical decompression has been reported.60–63 Krengel and colleagues favored early posterior decompression and instrumented fusion and demonstrated that surgery was safe and improved neurological recovery in comparison to historical controls managed nonoperatively or by late surgical intervention.4 With a focus on incomplete upper thoracic injuries, Bohlman and coauthors reported an improvement of 2.0 Frankel grades per patient with anterior surgical decompression.64
Although two randomized prospective studies have compared operative with nonoperative management of neurologically intact patients with thoracolumbar burst fractures, there are no comparable studies for thoracic fractures.65,66 The multiple hurdles encountered when performing randomized trials involving surgical intervention have prompted some to explore novel methods of cohort comparison in the trauma population. Most recently, Stadhouder and coworkers performed a parallel cohort study based on clinical equipoise in which operative and nonoperative treatment of traumatic thoracic and lumbar fractures were compared. With a mean follow-up of 6.2 years, pain scores, disability indices, and general health outcomes were comparable between the two groups; however, there was a nonstatistically significant trend toward better neurological recovery in the surgical group.9
The preponderance of evidence points to the importance of restoring anatomic alignment. Whitesides was adamant that persistent or progressive kyphosis inhibited neurological recovery.20 In their studies of fractures of the thoracolumbar junction, Shuman, Jacobs, and colleagues found a direct correlation between progressive posttraumatic kyphosis and progression of neurological symptoms.67,68 Similarly, in a follow-up study of patients with unstable thoracic and lumbar fractures, Soreff and associates showed a significant correlation between spinal deformity and symptomatology, and Edwards and coworkers’ data on patients with posttraumatic paraplegia suggested that anatomic restoration was important for good long-term results.69,70 Nevertheless, Nicoll did not find any link between the degree of spinal deformity and symptoms, and McAfee and colleagues, in reviewing anterior decompression and fusion in patients with incomplete deficits, found that residual kyphosis did not preclude neurological improvement.24,71 In the absence of cystic degeneration of the spinal cord, neurological deterioration in the setting of spinal deformity has been associated with progressive kyphosis, stenosis, arachnoiditis, and spinal cord tethering.72 The potential morbidity of untreated deformity and the increased neurological risks encountered with delayed surgical management have caused some to argue that long-term outcomes may be more satisfactory when posttraumatic deformity is corrected with earlier surgical intervention.73
Nonoperative management of most thoracic and lumbar fractures has been fairly successful and almost as heterogeneous as surgical interventions—bed rest alone versus some combination of postural reduction, bracing, casting, early mobilization, and physical therapy.52,74–76 Most of the bracing literature focuses on treating thoracolumbar junction fractures with thoracolumbosacral orthoses (TLSOs). Some have argued that many thoracic fractures do not require bracing because an intact rib cage and sternum will splint the affected portion of the spinal column. Occasionally, fractures of the upper thoracic spine are braced by obtaining purchase from the cervical spine via mandible or occipital pads or with a halo ring; such braces are categorized as cervicothoracic orthoses or cervicothoracolumbosacral orthoses.2 Unstable, purely upper thoracic spine fractures have been successfully treated with orthotic bracing alone; in one series of 49 patients with upper thoracic fractures treated with bracing, no failure of arthrodesis was seen, yet complications included 1 case of neurological worsening, 8 cases of brace-related skin necrosis, and 12 cases of deep venous thrombosis.77
Clearly, the risk for complications must also weigh into the decision of whether to proceed to the operating room. Gertzbein and associates, in a large multicenter study of 1019 thoracolumbar fractures, found a complication rate of 3% in the nonoperative group and 25% in surgically managed patients.10 However, one must be wary of such comparison studies given the lack of randomization and the realization that surgery is usually offered to patients with more severe injuries and neurological deficits. Proponents of surgery argue that it facilitates mobilization and rehabilitation. There is evidence that hospitalization time can be shortened with surgical stabilization in patients with thoracic and lumbar fractures causing paralysis.78–80
Mirza and associates offer specific treatments for spinal fractures based on the classification of McAfee and colleagues and the presence or absence of a neurological injury. The specific recommendations are based on the authors’ collective experience and represent more traditional, principally orthopedic approaches to the treatment of spinal fractures. The recommendations have not been scientifically validated (Table 318-2).81,82
TABLE 318-2 Treatment Guidelines as Identified by Mirza and Associates
INJURY TYPE | SUGGESTED TREATMENT |
---|---|
Compression fracture | Observation with follow-up or prefabricated brace immobilization for 12 wk |
Burst fracture, stable | Custom-fitted orthosis or cast immobilization for 12 wk |
TLSO, L4 and above | |
TLSO or HTLSO, L5 and below | |
Hyperextension cast or brace if kyphosis >15 degrees | |
Burst fracture, unstable | Approach for surgical decompression and stabilization is controversial |
Emergency posterior short-segment decompression and fusion with immobilization in a custom TLSO for 12 wk | |
Delayed anterior decompression and fusion if the patient has residual cord compression in the setting of a neurological deficit | |
Flexion-distraction (Chance) | Hyperextension cast if osseous injury with no associated neurological deficit and no abdominal injury (Chance injury) |
Posterior short-segment stabilization and fusion if associated neurological injury or abdominal injury or when the spinal injury is primarily ligamentous | |
Fracture-dislocation | Posterior long-segment surgical stabilization with pedicle screw fixation of two to three levels above and below the injury and fusion with local bone graft |
HTLSO, hip thoracolumbosacral orthosis; TLSO, thoracolumbosacral orthosis.
Modified from Mirza SK, Mirza AJ, Chapman JR, et al. Classification of thoracic and lumbar fractures: rationale and supporting data. J Am Acad Orthop Surg. 2002;10:364.
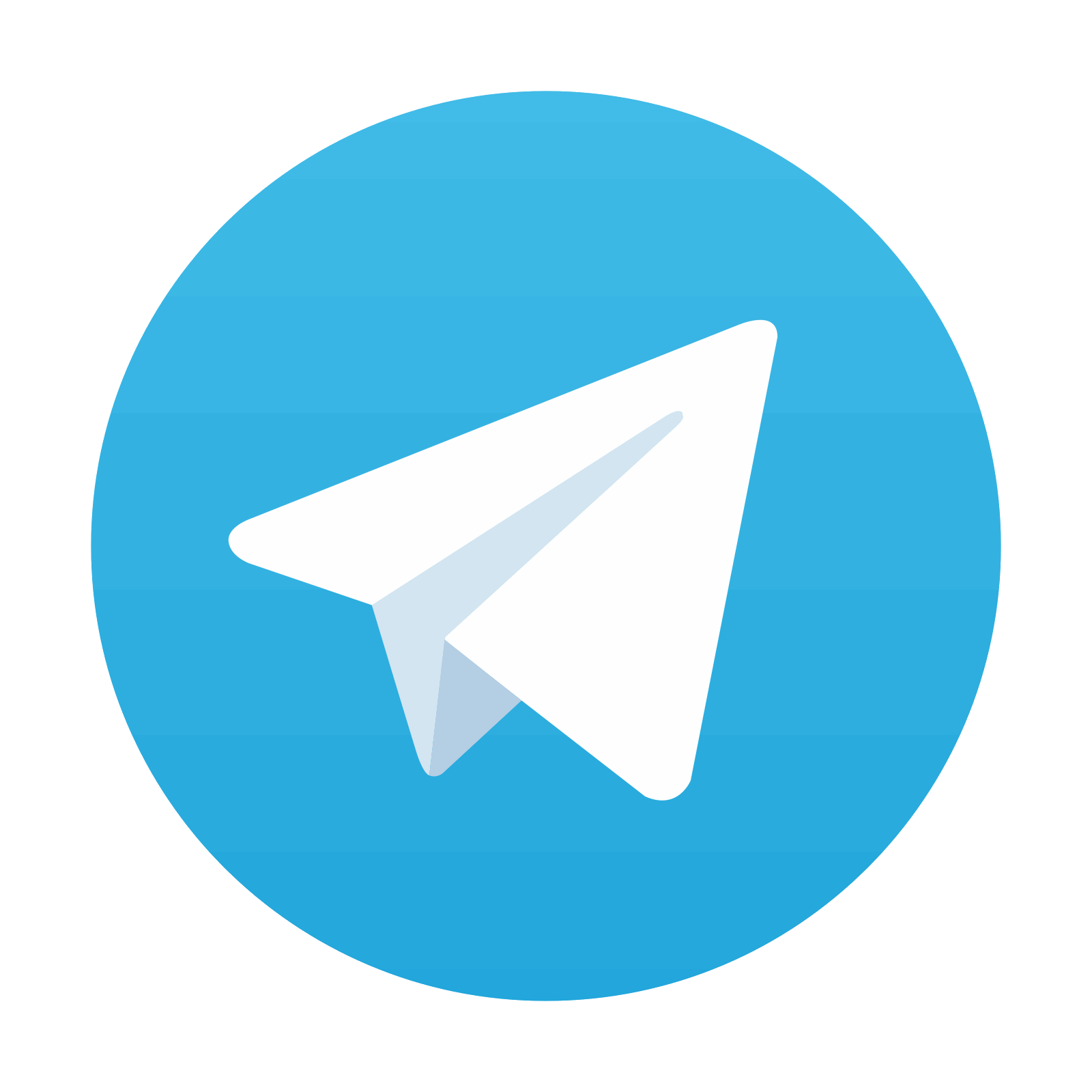
Stay updated, free articles. Join our Telegram channel

Full access? Get Clinical Tree
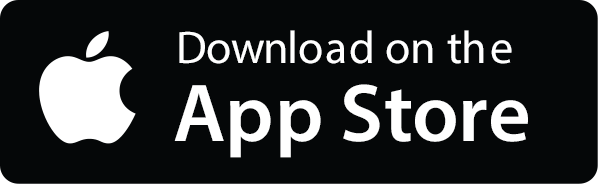
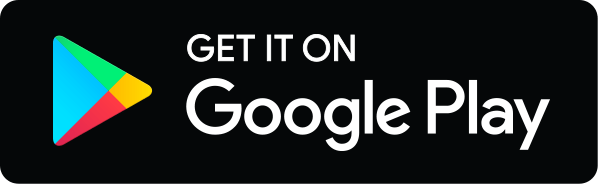