Electrocorticography
Marc R. Nuwer
Electrocorticography (ECoG) is intraoperative electroencephalography (EEG) recorded directly from the exposed cerebral cortex. The technique is used for localizing epileptic discharges and regions of impaired cortex prior to resection. Together with direct cortical stimulation, the techniques are used to identify motor, language, and sensory cortex during tumor resection. The goal of ECoG is to separate regions suitable for resection from those that should be left intact.
ECoG was the first application of intraoperative neurophysiology, a field that has since evolved to include many other techniques (1). Even as far back as the 1940s, ECoG has been used to localize epileptogenic tissue prior in the neurosurgical treatment of partial epilepsy (2, 3, 4, 5, 6 and 7). The equipment for ECoG has evolved since then, but the clinical goal remains relatively unchanged.
The French group (8, 9 and 10) developed a method called stereoencephalography (SEEG) to record from deep structures and buried cortex that are not readily accessible by subdural or cortical methods of recording. In this way, ECoG can be obtained simultaneously from superficial and deep brain structures. A review (11) of the SEEG technique in relation to other methods of ECoG notes that SEEG exploration is well tolerated by the majority of patients and overall complication rates of SEEG are reported as being of the order of 5% (12,13). This is similar to complication rates of subdural strips (14) and is somewhat less than those of subdural grids (15). Although acute depth electrodes appear to be “more invasive” than subdural recordings, the complication rate is not higher.
ELECTRODES AND METHODS OF RECORDING DIRECTLY FROM CEREBRAL CORTEX
ECoG recording from the exposed cerebral cortex most often uses strip and grid electrodes. These electrodes are arrays of 3-mm metal discs embedded in a flexible silicon rubber matrix. Each disc is 1 cm apart measured center to center. Discs are stainless steel or platinum alloy. Fine flexible wires connect to each disc. These wires course through the silicon rubber matrix and are gathered together to exit at one end of the strip or grid. Together they form a ribbon cable or braided wire connector cable leading to a multipin connector. A matched multipin connector with grounded heavier wiring transmits to the standard EEG machine electrode junction box. Strips and grids come in many different sizes. Sizes used more often in surgery are 1 × 8 strips and 4 × 5 grids. For patients in whom these are left in place chronically in the subdural space for days or weeks to catch epileptic seizures in an epilepsy monitoring unit, grid sizes up to 8 × 8 are available.
When used in surgery, ECoG grids and strips tend to curl up or pull slightly away from the cortex especially in sulci. This results in poor electrical connection to some contacts. To prevent corners pulling up, the surgeon should place saline-wetted surgical Cottonoid over each strip or grid to weigh it down. These strips and grids can be moved around to record from various regions of exposed cortex, or even under the dura or skull edges, to record from as many regions as needed.
Montages are either bipolar among the adjacent contacts or referential to a distant site. A clip electrode on muscle or a disc scalp electrode can serve as a distant neutral reference. Some users prefer the mastoid site. Because the amplitude of ECoG is several-fold higher in amplitude than EEG picked up by electrodes on the muscle or scalp, referential recordings are often used without excessive channel contamination by an active reference.
A low-frequency filter of 0.5 Hz and a high-frequency filter of 70 Hz ensure adequate recording of epileptiform discharges and background activity. The amplitude of EEG recorded directly from the cortex is several-fold higher than EEG recorded from the scalp. This allows for a relatively low sensitivity of 30 to 50 µV/mm to be used for ECoG recordings. A collection of 20 to 40 cortical electrodes is recommended, which often are composed of signals from more than one grid or strip.
DIRECT CORTICAL STIMULATION TECHNIQUE
Direct cortical stimulation is used to localize functional cortex. This technique requires special instrumentation and precautions. Generally equipment specifically designed for this purpose is used. Contact with the cortical surface usually is made using a bipolar stimulation wand or probe held against the cortex. Alternately, the stimulation may be delivered through the grid or strip electrodes; the latter stimulation technique is used more often for the subacute subdural studies on epilepsy monitoring units. The wand stimulation technique is more convenient during surgery where the cortex is directly exposed. The typical stimulating wand or probe is 30 cm long, with two
round metal ball tips separated by a few millimeters. Such a wand can move among cortical locations quickly. This allows for testing many different locations in a short time.
round metal ball tips separated by a few millimeters. Such a wand can move among cortical locations quickly. This allows for testing many different locations in a short time.
Stimuli are bipolar and biphasic. Bipolar means that electric current runs between the two adjacent active contacts. Biphasic means that the current switches polarity halfway through each stimulus pulse. Biphasic stimulation is preferred in the ECoG setting so as not to leave a net electrical polarization at either metal-cortex contacts. Polarization could allow metal ions to move onto the brain, which is undesirable especially for iron ions that would serve as a source of future cortical irritation. Polarization alters the electrical sensitivity of the underlying cortex to subsequent stimulus pulses. Bipolar biphasic stimulation equipment is available, but most stimulation equipment for routine intraoperative monitoring is not biphasic. The latter routine stimulation equipment is unsuitable for direct cortical stimulation studies.
Typical direct cortical stimuli are 100 to 300 µsec (0.1 to 0.3 msec) pulses delivered in trains of 50 pulses per second for several seconds. A 5- to 7-second stimulation train is used for language testing, whereas shorter trains are used for motor testing. Stimulus pulses are delivered using currents up to 14 milliamperes (mA) for the usual wand or probe designs. This is sufficient to disrupt function at the cortex underlying the wand’s location. Often one starts with a lower intensity, for example, at 4 to 6 mA, increasing gradually to higher intensities if no desired or adverse effects are seen at the lower intensities at each site. Either constant current or constant voltage stimulation may be used. Duration longer than 8 seconds should be avoided.
During direct cortical stimulation, ECoG is recorded from nearby sites. This is carried out to check whether epileptic-like discharges occur with the stimulations. When epileptic-like discharges last longer than the stimulation, they are referred to as afterdischarges. Afterdischarges appear as rhythmic repetitive high-voltage spikes or polyspike-wave complexes lasting seconds or occasionally minutes. Such prolonged afterdischarges are usually subclinical or associated with subtle clinical behavioral manifestations (18). When afterdischarges occur, stimulation may have spread to other cortical regions. Therefore those stimulation trials should not be used to localize cortical functions because they include activation of areas away from the wand’s tip. Afterdischarges may herald epileptic seizures occurring with further stimulation at that site or intensity. This may prompt moving the stimulating wand to a different site or to reducing the stimulus intensity.
Cortical stimulation can provoke epileptic seizures. Cold Ringer’s lactate solution can treat seizures provoked by cortical stimulation. Such irrigation rapidly stops partial seizures (19).
INTERPRETATION OF ECOG AND DIRECT CORTICAL STIMULATION
The goal of ECoG is to determine locations of epileptic or otherwise impaired cortex. The recording usually shows EEG features similar to those seen with scalp EEG. Frontocentral fast activity, low-amplitude generalized slowing, and frontopolar triangular slow waves are seen during many types of anesthesia. Recordings above and below the sylvian fissure would show more fast activity above and less below. ECoG background activity is more sharply contoured than typically seen on scalp EEG recordings; this sharply contoured EEG is similar to the scalp EEG over a skull breach. This sharply contoured background needs to be distinguished from epileptic activity during interpretation. Some ECoG epileptiform spikes may not be apparent on concurrent scalp-recorded EEG (20). Areas of impaired cortex may show relatively decreased fast activity, increased slow activity, or epileptic spikes. A knowledgeable and experienced physician neurophysiologist identifies these changes and thereby identifies regions with impaired function.
ECoG is used for certain patients during epilepsy surgery. Most often this is when the epileptogenic zone was inadequately localized by other methods. ECoG is used to further define the resection limits. For patients with very frequent seizures during either scalp or invasive EEG monitoring, intraoperative ictal ECoG may be useful to demarcate the electrographic ictal onset zone more precisely. An interictal ECoG is much more commonly obtained. Interictal spike discharges usually arise from the epileptogenic zone but also extend to cortex beyond it. Those regions are defined and discussed with the surgeon to tailor the resection to the needs of the individual patient.
Sometimes ECoG is performed after resection. Epileptiform discharges observed at the resection margins may just represent “injury potentials” or “injury spikes,” not the location of additional epileptic tissue. Epileptic spikes distant from the resection margin are more concerning, and frontal lobe epilepsy patients with epileptiform discharges three or more gyri away from the resection site indicated likelihood of seizure recurrence.
To avoid anesthetic effects, the anesthesia is lightened or minimized during the ECoG. Many anesthetics can alter the presence of epileptiform discharges sought in ECoG. The effects of the anesthetic on the ECoG are clearly less important than the anesthetic’s clearance time. Propofol clears adequately to allow good ECoG in most patients. Nitrous oxide and narcotics may be used during the ECoG to avoid pain or consciousness during the procedure, although these may affect the epileptiform discharges seen on the recording.
Direct cortical stimulation testing is interpreted by the presence of movement or sensation or the disruption of language. Neuromuscular blockade needs to be avoided. For motor testing, anesthesia cannot be deep. Observations of responses can be made visually, or EMG electrodes can be placed in various muscles to record movements under the surgical drapes. Stimulations then can identify various face, arms, and leg regions. Finding stimulation effects at some cortical areas shows that the anesthetic depth is adequate and the technique is working properly. Considerable individual variability exists in location of eloquent cortex regions for language, motor, and sensory function (21, 22 and 23). Anatomic locations need to be determined for each eloquent function for each individual patient. Stimulation mapping allows for best resection of pathologic tissue while preserving function (24,25). Functional neuroimaging navigation methods are usually combined with stimulation mapping; such a dual approach is the current best guide for the limits of a resection (26,27).
The patient is awake during surgery for sensory and language testing. The conscious patient is extubated while restrained on the table (28). The primary sensory cortex can be mapped using such stimulation in the awake patient. For some awake epilepsy patients, stimulation near a seizure focus will cause the patient’s typical aura.
For language testing, a neuropsychologist or other trained language specialist administers language tasks for different aspects of auditory and visual language processing and short-term memory. The clinical neurophysiologist delivers the stimulations and observes for afterdischarges while the surgeon holds the wand and the language specialist administers oral and visual testing. Most adult patients tolerate this awake craniotomy language testing adequately. Stimulation at a series of cortical locations can identify functional language areas. Regions without noticeable disruption at 12 to 14 mA are considered candidates for resection.
The asleep-awake-asleep technique requires cooperative patients (28). Initial anesthesia is often propofol or sodium thiopental with endotracheal intubation. Local anesthetic is infiltrated around the operative site margins. The patient is awakened and extubated once the craniotomy exposure is achieved. Consistent intraoperative language performance baseline is needed before stimulation testing. Without baseline consistency, any observed language disruption might be due to inconsistent performance rather than specific anatomic disruption due to stimulation. After mapping is completed, the patient is reintubated and remains under general anesthesia for the remainder of the procedure.
Language mapping includes several types of tasks. Visual object naming involves the naming of objects presented as line drawings from the Boston Naming Test (29). Drawings are presented that the patient could name quickly in preoperative testing (21). Naming is a useful screening task for language during cortical stimulation mapping because naming deficits are frequently part of aphasia syndromes. Speech arrest or anomia may occur upon stimulating a cortical region important for language, although not all cortical language sites produce naming deficits (30,31). Different cortical sites may reveal stimulation deficits in sentence reading (32). Another easily accomplished stimulation language test is word generation in which patients generate lists of words that begin with a certain letter or are of a certain category (e.g., animals). In auditory responsive naming, the patient names objects, that is, the answer for “tall pink bird” would be “flamingo.” Auditory responsive naming and word generation tasks activate frontal language areas (33,34). Visual responsive naming differs from auditory responsive naming because the question is written on a flash card.
Stimulation mapping effects are well localized. Moving the stimulating electrodes 0.5 to 2 cm, even along the same gyrus, yields different functional effects (32,35). A site with consistent language function can be 2 cm from a site on the same gyrus without language disruption (21). Language function often is localized to small 1 to 2 cm2 regions (32,36). Postoperative language deficits occur when resection margins are within 1.5 to 2.0 cm of language function sites. No permanent language deficits were reported if the surgical margins remain >2.0 cm from language sites (32,36,37).
GENERAL ANESTHESIA AND CORTICAL EEG ACTIVITY
Anesthetic drugs tend to either excite or depress the EEG and follow a pattern summarized by Winters (38). The activating or exciting anesthetic agents are propofol and etomidate. They inhibit brainstem arousal mechanisms through GABAA receptor mechanisms (39,40). The resulting EEG patterns resemble slow-wave sleep including vertex sharp waves and spindles. Despite early reports, propofol does not produce EEG epileptiform activity (41, 42 and 43). Propofol is a popular agent for ECoG recording and before direct cortical stimulation. Because of its rapid metabolism, propofol can induce unconsciousness during portions of the craniotomy. Yet it is eliminated rapidly enough to allow intraoperative awakening, a major advantage for ECoG (44,45). Etomidate is another activating or exciting anesthetic agent. Like propofol, it enhances epileptic activity at low doses (0.1 mg/kg). It may produce seizures in patients with epilepsy (46). The paradoxical excitatory effect of low-dose propofol may result from a membrane-level interaction between the GABAA current and an intrinsic membrane slow potassium current (M-current) according to a proposal by McCarthy et al. (47). In that theory, a potential network mechanism underlying the generation of propofol-induced paradoxical excitation consists of an interneuron antisynchrony, one that also produces an EEG beta rhythm at low drug concentrations.
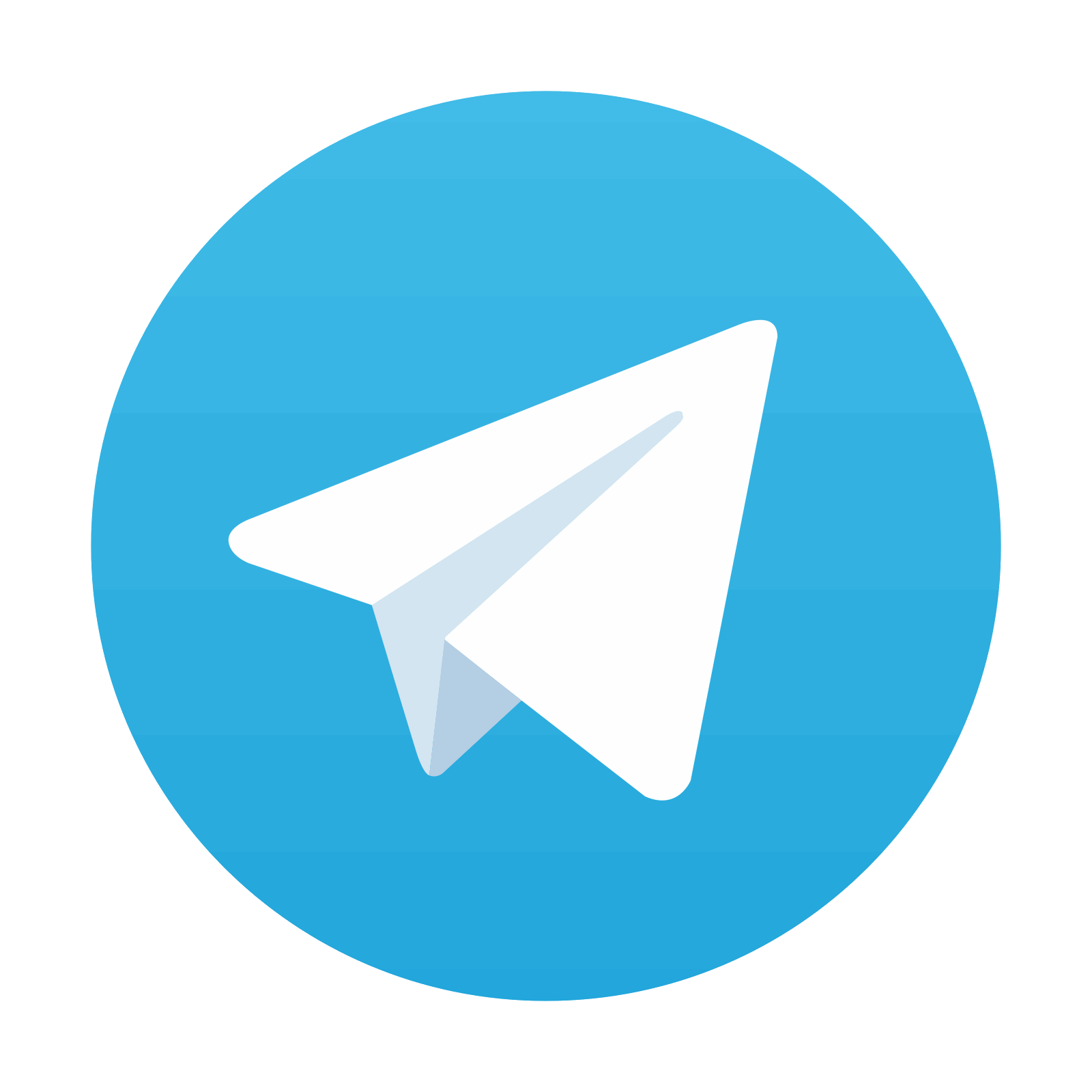
Stay updated, free articles. Join our Telegram channel

Full access? Get Clinical Tree
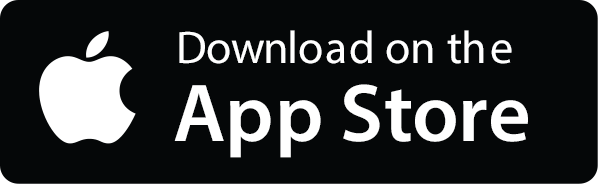
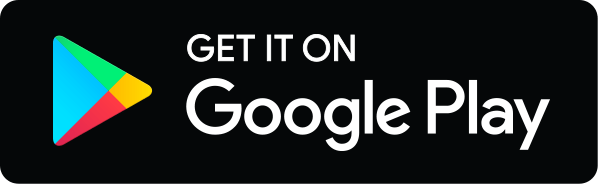