Chapter 5 Electroencephalographic Electrodes, Channels, and Montages and How They Are Chosen
In addition to scalp electrodes placed to record brain electrical activity, additional “monitoring” electrodes may be placed on nonscalp areas to record other physiologic activities including heartbeat, respirations, eye movements, contraction of certain muscles, including the respiratory muscles, and limb movements. The schema for the placement and naming of the EEG electrodes according to the international 10-20 system was described in Chapter 3. While considering the strategies used in different montage designs, the reader should consider how montage choice affects the reading process and also how new montages might be designed in special situations. Such situations could include a patient in whom part of the head is not accessible for one reason or another (e.g., a surgical bandage) or the occasional patient in whom accurate localization requires the placement of intervening electrodes.
CATEGORIES OF MONTAGES: REFERENTIAL AND BIPOLAR
Montages are generally divided into two large groups, referential and bipolar, denoting the technique by which EEG data are displayed. Because there are significant differences in the strategies used by these two montage families, each is discussed in its own section. Localization techniques for each of these montage types were discussed Chapter 4.
Recommended Montage Conventions
Although electrode channels can be presented in any order when creating a montage, certain conventions are encouraged (see the American Clinical Neurophysiology Society [ACNS] Guidelines in References): channels should be placed in a “left over right” configuration (electrode chains from the left side of the head are placed nearer the top of the page, and chains from the right side of the head are placed nearer the bottom of the page). More anterior electrodes should be placed before more posterior electrodes (front-to-back ordering of channels going down the page is encouraged). Interelectrode distances should be consistent (electrode positions should not be unpredictably skipped in electrode chains). Finally, the design should favor the simplest arrangement possible (see Figure 5-1).
In the days of paper EEG recording, it was up to the EEG technologist to choose the montage that would best demonstrate the patient’s findings at any particular point in the tracing. If an EEG event happened to be recorded in a montage that was disadvantageous for interpretation, the ink had already dried on the page, and it was not possible to change settings retrospectively. The newer digital EEG technology presents a new set of problems. Although the same EEG page can be viewed in a variety of montages during the course of interpretation, some readers may find that it takes less energy to leave the display in one montage for the whole tracing, usually choosing the montage with which they are most comfortable. Although scanning a whole EEG study in a single montage may allow for faster reading, occasionally an EEG finding may be seen well in one montage but only poorly seen, or perhaps not seen at all, in another. It is therefore best practice for the EEG technologist or the reader to cycle through a minimum set of montages during the course of each study. This will usually include some combination of longitudinal bipolar, transverse bipolar, and referential montages.
MONTAGE DESIGN
Referential Versus Bipolar Montages
The term bipolar derives from the fact that, as can be seen from this chain, each channel represents the voltage difference between two “poles” on the scalp. Bipolar montages are occasionally referred to as differential montages because they display the difference between adjacent electrodes. Although the bipolar technique is a powerful recording technique and a favorite among many readers, bipolar montages also have their drawbacks, as discussed later. Figures 5-2 and 5-3 show a schematic of the difference between the referential and bipolar recording strategies.
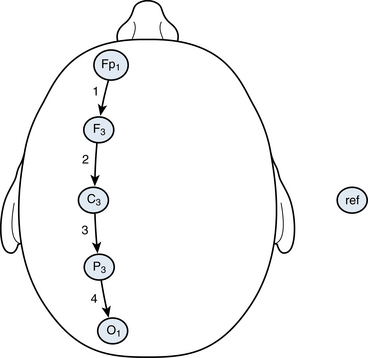
Figure 5-3 In this illustration, the same five parasagittal electrodes used in Figure 5-2 are connected to each other sequentially in a standard “bipolar chain.” Again, each arrow points from an Input 1 electrode to an Input 2 electrode creating the following montage: Fp1-F3, F3-F3, C3-P3, and P3-01. The reference position is not used. Each of the four channels generated with this technique represents the subtraction of an electrode from the previous electrode in the chain. Because the electrodes are paired to form each channel, five electrodes generate four channels of output.
Theoretical Strategies: How Can EEG Activity Best Be Recorded From a Single Point?
Recalling that our basic tool for creating an EEG channel is to print the amplified output of a voltmeter for which the needle sweeps one way or the other depending on the voltage difference between two points, there must always be two separate amplifier inputs, Input 1 and Input 2, to make a comparison. We decide to attach the “electrode of interest,” Fp1, to one pole of our voltmeter (Input 1), but what would be the most advantageous choice of electrode(s) to attach to Input 2, the comparison point? This comparison electrode is called the reference electrode, and serves as the reference point against which the electrode of interest is measured. As described in Chapter 4, the voltage measured from the reference will be subtracted from the voltage measured at Fp1, and the resulting difference is the output for the channel that we will label as Fp1-ref.
It is clear that the choice of the reference electrode could have as big an impact on the appearance of the resulting output trace as the Fp1 electrode. At first glance, the channel label “Fp1-ref” may seem to imply that its output represents the voltage at Fp1. Note, however, that the “active electrode,” Fp1, does not enjoy any privileged position by being attached to Input 1 as opposed to Input 2; the two voltages will simply be subtracted one from the other and displayed. For that reason, if the chosen reference electrode were the earlobe and there happened to be more electrical activity present in the earlobe than in Fp1, the output of an Fp1-earlobe channel would be dominated by the electrical activity in the earlobe—not the desired result. Clearly, the choice of the reference electrode in referential montages is important.
Choice of a Reference Electrode
Finally, consider the extreme example of moving the reference electrode so close to Fp1 that it is nearly in the same position as Fp1. In such an example, the signal in Fp1 and the reference are so similar that the difference output will become nearly flat. Some generalizations can then be made regarding the distance between the electrodes attached to Input 1 and Input 2 of a channel’s amplifier. When two electrodes are moved closer to one another, there is greater commonality between what each detects in terms of both the noise signal and the brain wave signal. Therefore, as two electrodes become closer to one another, a channel comparing them will flatten. Conversely, as interelectrode distances increase, there is a bigger difference in both the noise signal and the brain wave signal and the channel”s output signal tend to increase.
All things being equal, larger interelectrode distances are associated with higher voltages, and smaller interelectrode distances are associated with lower voltages. This effect explains the recommendation that electrodes should not be skipped in bipolar montage chains (or stated another way, that interelectrode distances should be held constant). A channel pair with an inconsistently large interelectrode distance will tend to produce a higher voltage channel, possibly giving the erroneous impression that there is more electrical activity at that location than at adjacent locations. Variation in interelectrode distances may occur in two ways: a montage design that skips an electrode or a significant mismeasurement of electrode position during electrode application (see Figure 5-4).
What would the ideal reference electrode location be?
Each potential reference location is prone to certain problems and advantages—no single reference electrode position is ideal for all patients at all times. For instance, the earlobes often share a portion of the electrocerebral activity present in the adjacent midtemporal areas, causing a cancellation effect. This makes an earlobe reference a poor choice for a patient with a high voltage midtemporal discharge. Also, the left earlobe in particular is often contaminated with EKG artifact because of the left-sided position of the heart. In some, the nose reference may be contaminated by a surprising amount of muscle artifact, whereas in others it can be fairly clean. The cervical area is contaminated by muscle artifact or EKG signal in some patients and may also be disturbed by movement when patients are lying on their backs. The midline vertex electrode, Cz, is usually free of muscle artifact because of its location at the vertex of the scalp but contains a large amount of electrocerebral activity, especially during sleep. In different individuals, any of these reference locations could generate satisfactory or unsatisfactory recordings at different times.
The main difference between the two systems, one of which alternates left- and right-sided electrodes and the other of which groups left-sided electrodes in one area and right-sided electrodes in another, is the comparative ease of visual scanning for asymmetries. Choice of one of these arrangements over the other is essentially a matter of preference. Figures 5-5 and 5-6 show examples of dramatic left-sided slowing displayed with each of these two common strategies. The latter system, which groups electrodes together by location, is seen to have certain advantages. Asymmetries that occur between hemispheres are not usually localized to a single electrode but to a regional group of electrodes. For instance, if a temporal slow wave is present, it may be recorded in multiple electrodes such as F7, T7, and P7. When an abnormality such as a spike or a slow wave is present in multiple adjacent channels on a page, it tends to be easier to identify visually when the spatial clustering system is used. In the alternating left–right setup, the abnormality will only be seen in alternating channels. Another advantage of the clustering system is the ease of visualizing the topography of the discharge on the scalp; with this system a region of the page corresponds to a region of the brain starting with the fact that the left hemisphere is represented on the top of the page and the right hemisphere is on the bottom. Grouping the channels by region, especially when gaps are used between electrode groups as in the example illustrated here, makes it simple to know where a given channel is on the head at a glance, even without reading the channel labels. In contrast, when reading in a montage in which the left and right channels are alternated, it can be difficult to know the location of each electrode without consulting the channel labels. Proponents of the left–right alternating system like the fact that homologous channels are adjacent to one another, making individual interchannel comparisons easier. Considering these reasons together, the author prefers the clustering system (left over right and front to back), which should allow for easier and more accurate scanning and spatial visualization for most readers. However, the choice of either of these two arrangements is really a matter of laboratory or reader preference.
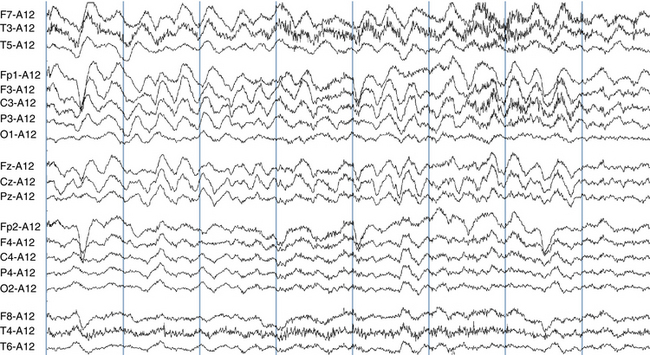
Figure 5-6 This EEG page shows the exact same left-sided slowing as was seen in the previous figure, however, the channels are ordered in chains so that the left hemisphere electrodes are shown on the top half of the page and the right hemisphere electrodes on the bottom. Starting at the top, channels are grouped on the page as the left temporal, left parasagittal, midline, right parasagittal, and right temporal chains (see channel labels). The same reference is used as in the previous figure, an average of the A1 and A2 electrodes. Note that, with nearby electrodes grouped together, it is visually much easier to appreciate the localization of the slowing over the left side of the brain (top half of the page). For this reason, this arrangement is favored by the author.
Categories of Bipolar Montages
There are two principal categories of bipolar montages, the anteroposterior (AP) bipolar montage and the transverse bipolar montage. The two types differ in that, with AP bipolar montages, electrode chains run from front to back (anteroposteriorly) down the head, and in transverse bipolar montages, the chains run from left to right (transversely) across the head. The fact that the reader has both of these montage families available for use raises the question of whether any particular discharge might only be visible in the AP bipolar but not in the transverse bipolar montage, or vice versa. Is it really necessary to use both AP and transverse bipolar montages for EEG interpretation, and, if so, what would be lost by not doing so?
Circumferential Montages
Some laboratories also use a third ordering for bipolar electrode chains, one that makes a complete circumference around the head (see Figure 5-7). This style of montage has been given a variety of names, such as a halo, circumferential, or hatband montage. Close examination of the electrode pairs used in the circumferential montages shows that almost every pairing is also present in the standard AP bipolar montage. The only electrode pairings that are unique to circumferential compared with AP bipolar montages are the Fp1-Fp2 and O1-O2 pairs. Because these two pairs are included in the transverse bipolar montages, circumferential montages are not a mandatory member of a laboratory montage set, although the decision to use such a montage is at the discretion of the electroencephalographer. For this reason, the use of a minimum of three montages—AP bipolar, transverse bipolar, and referential—is usually adequate to cover all necessary electrode pairings in standard EEG recording. Circumferential montages, when used, lend themselves to a particular technique for visual scanning. A visual axis is chosen and surrounding channel “layers” are compared (see Figures 5-8 and 5-9).
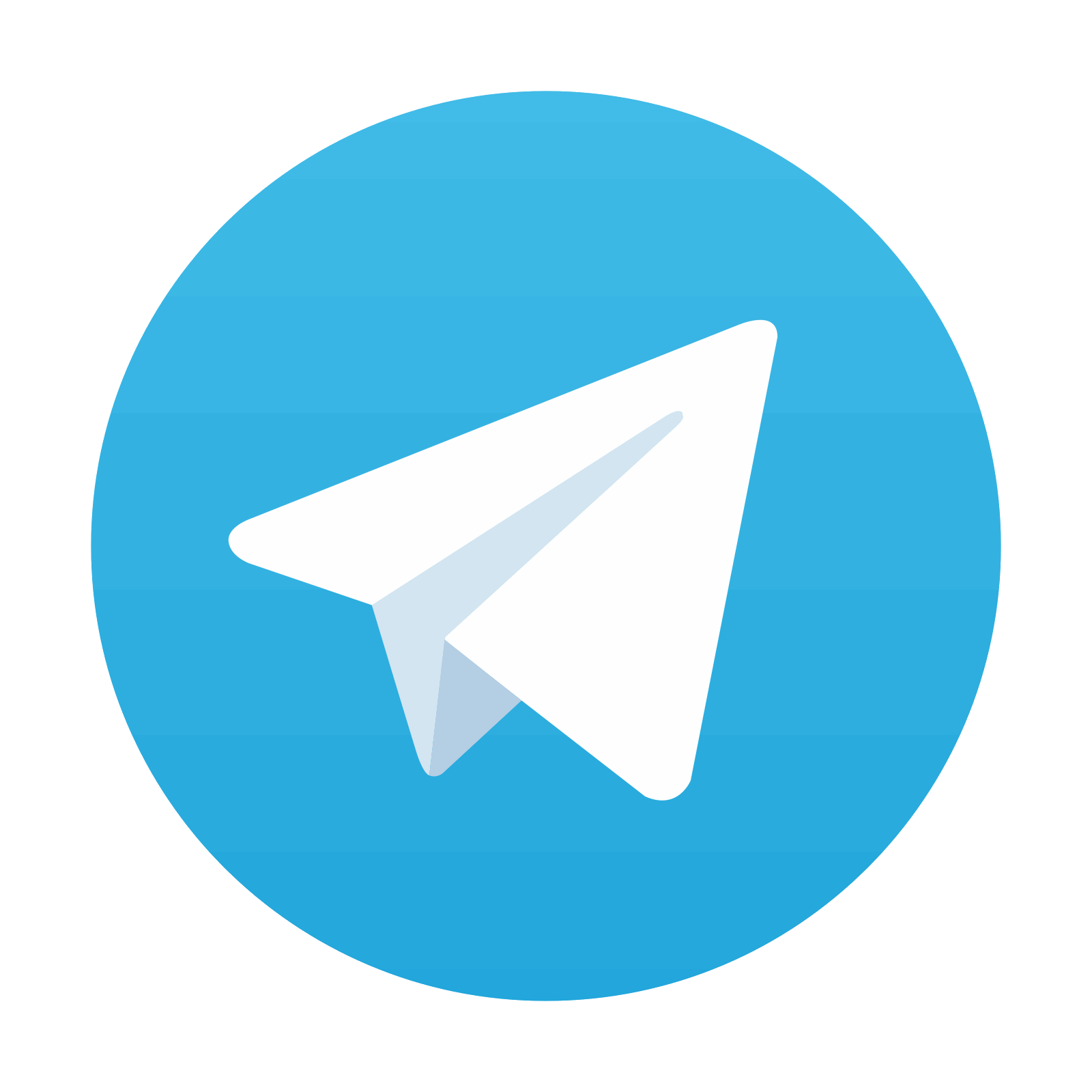
Stay updated, free articles. Join our Telegram channel

Full access? Get Clinical Tree
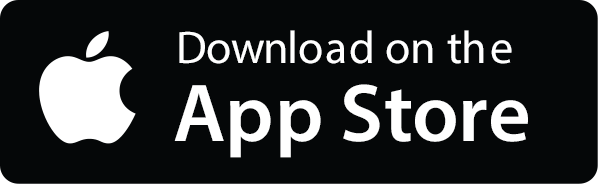
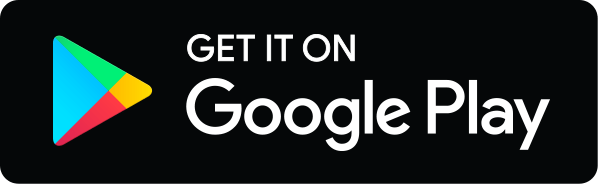