The electroencephalogram (EEG) represents the electrical activity of the brain as recorded from electrodes placed on the scalp. Many clinical neurologists and neurosurgeons do not fully appreciate the potential value or the limitations of electroencephalography, and this lack of information is reflected in the manner in which they use this technique in clinical practice. On the one hand, patients are often referred indiscriminately for study with little, if any, information provided about their clinical background. On the other hand, patients for whom electroencephalography might be expected to provide clinically useful information are not investigated by this means at all.
Electroencephalography is most useful in the investigation and management of patients with epilepsy. The presence of “epileptiform” activity ( p. 49 ) in the EEG of a patient with suspected epilepsy does not establish the diagnosis beyond doubt because similar activity may occasionally be found in patients who have never had a seizure. It is, however, one more factor that must be taken into account when patients are evaluated clinically. In patients with behavioral or other disturbances that could be epileptic in nature, but about which there is some uncertainty, the presence of such activity increases considerably the likelihood that the attacks are indeed epileptic. In patients with an established seizure disorder, the EEG findings may help to classify the disorder, identify a focal or lateralized epileptogenic source, indicate the most appropriate medication that should be prescribed, provide a guide to prognosis, and follow the course of the disorder.
Electroencephalography also provides a noninvasive means of localizing structural abnormalities, such as brain tumors. Localization is generally by an indirect means, however, depending on the production of abnormalities by viable brain in the area of the lesion. Moreover, it is sometimes disappointingly inaccurate, and the findings themselves provide no reliable indication of the type of underlying pathology. In this context, it is hardly surprising that advances in neuroimaging techniques for the detection of structural abnormalities in the brain—in particular, by the development of computed tomography (CT) scanning and magnetic resonance imaging (MRI) —have led to a reduction in this use of electroencephalography as a screening procedure. Nevertheless, the EEG reflects the function of the brain and is therefore a complement, rather than an inconsequential alternative, to these newer procedures.
The third major use of electroencephalography is in the investigation of patients with certain neurologic disorders that produce characteristic EEG abnormalities which, although nonspecific, help to suggest, establish, or support the diagnosis. These abnormalities are exemplified well by the repetitive slow-wave complexes sometimes seen in herpes simplex encephalitis, which should suggest this diagnosis if the complexes are found in patients with an acute cerebral illness. The electrical findings are best regarded as one more physical sign, however, and as such should be evaluated in conjunction with the other clinical and laboratory data. A further use of electroencephalography—one that may increase in importance with the development of quantitative techniques for assessing the data that are obtained—is in the screening or monitoring of patients with metabolic disorders, because it provides an objective measure of the improvement or deterioration that may precede any change in the clinical state of the patient.
Electroencephalography is also an important means of evaluating patients with a change in mental status or an altered level of consciousness. Continuous EEG monitoring provides dynamic information that allows for the early detection of functional changes and may thereby improve clinical outcome. This is especially useful when the clinical examination is limited or in patients at particular risk of deterioration, as after head injury or subarachnoid hemorrhage. Technical advances have made long-term monitoring practical, but review of the raw EEG traces remains important in ensuring the validity of any conclusions. Finally, the EEG is used for studying natural sleep and its disorders, and as help in the determination of brain death. Further comment on these aspects is deferred to Chapters 33 and 35 , and the clinical utility of the EEG in the investigation of infants and children is considered separately in Chapter 4 .
The EEG is now recorded with simultaneous functional MRI (fMRI) in various research contexts. The approach was originally developed to improve the localization of the generators of certain EEG abnormalities in epilepsy by mapping their hemodynamic correlates, but has developed into a sophisticated investigative tool.
In describing the EEG findings in various seizure disorders, the revised terminology for the organization of seizures and epilepsies proposed by the International League Against Epilepsy has been adopted, but the older terms are retained in parentheses for the convenience of readers.
Practical considerations
Recording Arrangements
The EEG is recorded from metal electrodes placed on the scalp. The electrodes are coated with a conductive paste, then applied to the scalp and held in place by adhesives, suction, or pressure from caps or headbands. Alternatively, needle electrodes can be inserted directly into the scalp. The placement of recording electrodes is generally based on the international 10–20 system, in which the placement of electrodes is determined by measurements from four standard positions on the head: the nasion, inion, and right and left preauricular points ( Fig. 3-1 ). When this system is used, most electrodes are about 5 to 7 cm from the adjacent electrodes, in an adult. If closer spacing is required (e.g., to define the site of an epileptogenic focus), electrodes can be placed in intermediate positions. The potential differences between electrodes are amplified and then recorded on continuously moving paper by a number of pen-writers or displayed on an oscilloscope screen. Relatively inexpensive digital systems are now widely available commercially, and these have many advantages over the older analog systems. They permit reconstruction of the EEG with any desired derivation or format and also permit data manipulation for added analysis. They also facilitate access to any desired portion of the record and obviate storage problems. Further discussion of such systems is provided in Chapter 8 .

Electrodes are connected with amplifiers in predetermined patterns, or montages, to permit the electrical activity of various areas to be recorded in sequence. The recording arrangements can be varied, or the EEG can be reconstructed after digital recording, so that the potential difference is measured either between pairs of scalp electrodes (bipolar derivation) or between individual electrodes and a common reference point. In the latter arrangement, the reference point can be either a relatively inactive site on the scalp or elsewhere (e.g., the vertex or the linked ears) or a point connected to all the electrodes in use so that it reflects the average of the potentials at these electrodes. Each technique has its own advantages and drawbacks, but for routine purposes at least two of these methods for deriving the EEG are used. Montages are generally selected so that recordings are made from rows of equidistant electrodes running from the front to the back of the head or transversely across it. In most North American laboratories, traces from the left side of the head are displayed above those from the right, and those from anterior regions are displayed above those from more posterior areas.
The more detailed technical and practical aspects of EEG recording are beyond the scope of this chapter. There is, however, one general point that must be made clear. As already indicated, the potential difference between pairs of electrodes—or between an electrode and its reference point—is amplified before being displayed on moving paper or an oscilloscope screen. The input leads of the individual amplifiers are designated as black (input terminal 1, or G1) and white (input terminal 2, or G2). They are arranged so that when the electrode connected with the black lead is relatively more negative than that connected with the white one, an upward deflection of the trace occurs. The relationship between the two inputs, then, determines the direction in which the trace is deflected, not the absolute value of any discharge that is recorded. With bipolar derivations, the conventional American recording arrangement is for the most anterior electrode of each pair to be connected with the black lead when recording from the front to the back of the head, and for the left-hand electrode of any pair to be connected with the black lead when recording across the head. With the reference derivations, each of the active scalp electrodes is connected to the black lead of an amplifier, and all the white leads are connected with the common reference point.
Interpretation of specific events in the EEG derived with a common reference point may be confounded if the reference electrode itself lies within the active field, whereas a voltage peak involving two adjacent electrodes to the same extent may not be detected in the bipolar derivation because no potential difference will be recorded between these electrodes. Both the bipolar derivation and the use of a common reference permit abnormalities to be localized, but the former method is less satisfactory for localizing widespread changes or for demonstrating areas in which activity is suppressed. With linked bipolar derivations, the source of localized EEG abnormalities is determined by locating the common electrode at which the deflections of the traces show a reversal of phase or polarity when recordings are made simultaneously from at least two rows of electrodes at right angles to each other (i.e., from rows of electrodes in the anteroposterior and transverse axes of the head). Changes in amplitude may be misleading in bipolar recordings. For example, a greater amplitude in one channel does not necessarily reflect the origin of a particular discharge but signifies only that a larger potential difference exists between the two electrodes connected to that amplifier; a lower amplitude may reflect inactivity or equal activity at the two electrode sites. By contrast, with common reference derivations, localization of abnormalities is accomplished primarily on the basis of amplitude.
Various special electrodes have been devised for recording the activity of inaccessible regions of the brain, because electrodes placed on the scalp may not detect such activity. A nasopharyngeal electrode, consisting of a flexible, insulated rod or wire with a small silver electrode at its tip, can be inserted into the nostril and advanced until the terminal electrode is in contact with the mucosa of the posterior nasopharynx. This permits recording of electrical activity from the anteromedial surface of the temporal lobe. Insertion of these electrodes is generally undertaken by technologists without the application of any local anesthetic, and not all patients tolerate the procedure. Furthermore, recordings from them may be contaminated by pulse, muscle, and respiratory movement artifact, and only rarely do they reveal abnormalities that are not seen with the usual scalp electrodes. Sphenoidal electrodes can record activity from the anteroinferior portion of the temporal lobe. These electrodes are less likely to lead to artifacts than are nasopharyngeal electrodes, but their application is more difficult. The electrode consists of a sterile needle or fine wire that is insulated, except at its tip, and a physician inserts it percutaneously under local anesthesia so that it lies adjacent to the sphenoid bone, a little in front of the foramen ovale. Other accessory electrodes that are commonly used in the electrophysiologic evaluation of patients with focal seizures and impaired consciousness (previously called complex partial seizures) are surface sphenoidal electrodes, minisphenoidal electrodes, and anterior temporal electrodes. There is no consensus of opinion regarding the relative merits of these different electrodes.
The EEG examination usually is undertaken in a quiet, relaxed environment, with the patient seated or lying comfortably with the eyes closed. Recordings initially are made for up to about 5 minutes from each of several different standard montages, for a total of about 30 minutes. Depending on the findings, the examination can then be continued by recording with less conventional montages. During the recording of activity from each montage, the patient should be asked to lie with the eyes open for about 20 seconds before closing them again, so that the responsiveness of the background activity can be assessed.
When this routine part of the examination has been completed, recording continues while activation procedures are undertaken in an attempt to provoke abnormalities.
Activation Procedures
Hyperventilation for 3 or 4 minutes is a generally well-tolerated method of provoking or accentuating EEG abnormalities, but it should not be performed in patients who have recently had a stroke, transient ischemic attack, or subarachnoid hemorrhage, or in those with moyamoya disease, significant cardiac or respiratory disease, hyperviscosity states, or sickle cell disease or trait. The patient is asked to take deep breaths at a normal respiratory rate until instructed to stop. The resultant fall in arterial PCO 2 leads to cerebral vasoconstriction and thus to mild cerebral anoxia. This anoxic state is held by many to bring out the EEG abnormalities, although cogent criticisms of this view exist and the precise mechanism involved remains unclear. Certain quantitative techniques have been suggested in an endeavor to establish a more uniform procedure, but these methods are not in routine use. The EEG is recorded during the period of hyperventilation and for the following 2 minutes, with the use of a montage that encompasses the area where it is suspected, based on clinical or other grounds, that abnormalities may be found; or, in the absence of any localizing clues, an area that covers as much of the scalp as possible. The eyes are generally kept closed during the procedure, apart from a brief period at its conclusion to evaluate the responsiveness of any induced activity. Hyperventilation usually causes more prominent EEG changes in children than in adults. There is considerable variation, however, in the response of individual subjects, and this variation makes it difficult to define the limits of normality. It may also produce or enhance various bioelectric artifacts.
Recording during sleep or after a 24-hour period of sleep deprivation may also provoke EEG abnormalities that might otherwise be missed, and this technique is similarly harmless. This approach has been used most widely in the investigation of patients with suspected epilepsy.
Abnormalities may also be elicited with an electronic stroboscope to cause rhythmic photic stimulation while the EEG is recorded with the use of a bipolar recording arrangement that covers particularly the occipital and parietal regions of the scalp. The flash stimulus is best monitored on one channel either with a photocell or directly from the stimulator. Abnormalities are more likely to be elicited when the patient is awake for the procedure. At any given flash rate, the EEG is recorded with the patient’s eyes open for about 5 seconds and then while the eyes are closed for a further 5 seconds. Flash rates of up to 30 Hz are generally used, but an even wider range of frequencies is employed in some laboratories. The manner by which abnormalities are produced is unknown.
Various auditory stimuli may also precipitate EEG abnormalities in patients with epilepsy, but they are not used routinely in the EEG laboratory, except in the evaluation of comatose patients ( p. 79 ). Other stimuli that may induce paroxysmal EEG abnormalities include tactile stimuli and reading.
A number of different pharmacologic activating procedures have been described. These procedures are not in general use, however, and may carry some risk to the patient; thus, they are not discussed in this chapter.
Artifacts
A variety of artifacts may arise from the electrodes, recording equipment, and recording environment. Examples are the so-called electrode “pop” resulting from a sudden change in impedance (seen as an abrupt vertical deflection of the traces derived from a particular electrode, superimposed on the EEG tracing, as shown in Fig. 3-2 ); distorted waveforms resulting from inappropriate sensitivity of the display; excessive “noise” from the amplifiers; and environmental artifacts generated by currents from external devices, electrostatic potentials (as from persons moving around the patient), and intravenous infusions (generating sharp transients coinciding with drops of the infusion, possibly caused by electrostatic charges). Bioelectric artifacts are noncerebral potentials that arise from the patient and include ocular, cardiac, swallowing, glossokinetic, muscle, and movement artifacts.

With reference derivations, artifacts may be introduced because of the location of the reference electrode or because the reference electrode is within the cerebral field under study. No single site is ideal as a reference point. The ear or mastoid placements are commonly used but may be contaminated by muscle, electrocardiogram (ECG), or temporal spike discharges. The vertex, which is also widely used, is very active during sleep and is sometimes contaminated by vertical eye movements during wakefulness.
In general, artifacts are recognized because of their temporal relationship to extracerebral monitors such as the ECG, because of their unusual appearance, or because the electrical field of the event is hard to interpret in a biologically plausible manner ( Fig. 3-3 ). They are discussed in detail in Chapter 5 , but brief comment concerning them is also made here.

Bioelectric Artifacts
Eye-movement artifacts are generated by the corneoretinal potential, which is in the order of 100 mV and has been likened to a dipole with the positive pole at the cornea and the negative pole at the retina. Eye movement leads to a positive potential recorded by the electrodes closest to the cornea. Thus, with upward movement of the eyes (such as occurs during a blink), a positive potential is recorded at the frontopolar (supraorbital) electrodes relative to more posteriorly placed electrodes, and thus a downward deflection occurs at these electrodes. Such eye movement is easily distinguished from frontal slow EEG activity by recording from an infraorbital electrode referenced to the mastoid process; the former leads to activity that is out of phase between the supra- and infraorbital electrodes, whereas frontal EEG activity is in phase. Similarly, horizontal eye movements lead to a positivity at the frontotemporal electrode on the side to which the eyes are moved and a corresponding negativity on the opposite side ( Fig. 3-4 ). Nystagmus or eyelid flutter, for example, produces a rhythmic discharge in the frontal electrodes (see Fig. 3-4 ). Oblique eye movements and asymmetric eye movements may be confusing, but are usually easily distinguished by experienced electroencephalographers.

Cardiac artifacts are related to the ECG or ballistocardiogram and are especially conspicuous when monitoring for electrocerebral inactivity in brain-death suspects or in referential recordings involving the ears (see Fig. 3-2 ). The ECG can be monitored by electrodes placed on the chest and recorded on a separate channel, thereby facilitating recognition of such artifacts in the scalp recording, which may otherwise be misinterpreted as sharp waves or spike discharges. Conspicuous ECG artifact may obscure underlying low-voltage electrocerebral activity, a point of concern when comatose patients are being examined for possible brain death. Pulse artifact may occur at any site but typically is localized to a single electrode and appears as a recurrent slow wave that sometimes has a saw-toothed appearance and is time-locked to the ECG. It occurs when an electrode is placed over or close to an artery, and movement of the electrode will eliminate it. Pacemaker artifacts consist of spike discharges that precede the ECG.
Muscle artifact is composed of brief-duration spike discharges that are too rapid to be cerebral in origin. Chewing artifacts are electromyographic (EMG) artifacts produced by the temporalis muscles (see Fig. 3-2 ). Sucking artifact (in infants) is characterized by bitemporal sharp activity that may be difficult to identify without careful observation of the baby. Lateral rectus spikes are recorded from the anterior temporal electrodes and relate to horizontal eye movements; they are out of phase on the two sides of the head. Attempting to relax or quieten the patient should reduce muscle artifact. In most instances, high-frequency filters should not be used for this purpose, except as a last resort, because they simply alter the appearance of the artifact (sometimes so that it looks more like EEG fast activity) and also influence the background EEG.
Movement produces artifact in addition to muscle activity. Any movements may produce such artifacts, and these will vary in appearance depending on the nature and site of the movement. Movements arising at a consistent site may be monitored by a pair of electrodes and recorded on a separate channel. Hiccups produce a brief generalized movement artifact that may have a pseudo-periodic quality; this is not confined to the EEG but is seen also in, for example, the ECG channel. Glossokinetic artifact relates to the difference in potential between the tip of the tongue and its base; tongue movements generate slow waves that are recorded over one or both temporal regions ( Fig. 3-5 ). When suspected, tongue movements may be monitored by a submental electrode.

Sweat artifact is common and is characterized by high-amplitude potentials of very low frequency. Low-frequency filters can attenuate these slow waves.
Instrumental Artifacts
Background 60-Hz noise in a restricted number of channels is often related to mismatched electrical impedance of electrode pairs or to the poor application of electrodes so that slight movement alters transiently the impedance of an individual electrode. A 60-Hz interference signal normally is common to the pair of electrodes connected to an amplifier; the differential amplifier essentially discards this common signal. If the electrical impedance of one of the electrodes is altered, however, the current flowing across that electrode–skin interface will be altered, thereby leading to voltage differences between one electrode and the other of the pair. The differential amplifier will magnify these differences so that the 60-Hz artifact then becomes obtrusive. In addition, other artifacts related to movement arise at that electrode and are limited to the channel with which the faulty electrode is connected, causing “mirror-image” phase reversals when these channels are part of a linked bipolar montage. Widespread 60-Hz artifact is of concern because it may indicate a safety problem warranting attention as discussed in Chapter 2 , where the various artifacts that arise in digital equipment also are considered.
EEG Interpretation
Evaluation of the EEG for clinical purposes involves definition of the frequency, amplitude, and distribution of the electrical activity that is present, and of its response to external stimulation such as eye-opening. The degree of synchrony and symmetry over the two sides of the head is noted. The presence of any focal activity is determined and its nature characterized. The findings must be interpreted in relation to the patient’s age and level of arousal. For descriptive purposes, EEG activity is usually characterized on the basis of frequency.
Activity recorded in the EEG
The mechanisms responsible for the presence of widespread rhythmic activity in the brain are not known. Some means of generating rhythmic activity must be involved, as also must some method of synchronizing the activity of different cerebral regions. Experiments on animals have produced considerable evidence to suggest that the rhythmic activity normally recorded from the scalp has a cortical origin, being derived from the graded postsynaptic potentials of cortical neurons. It is the pyramidal neurons—cells that are vertically oriented with regard to the cortex, have a large apical dendrite extending toward the surface, and are located in cortical layers III, V, and VI—that are important in this respect. The origin of the scalp-recorded EEG primarily from postsynaptic potentials, rather than action potentials, is in keeping with the former’s longer duration and synchronous occurrence over a large area of cell membrane. Potentials arising from neuronal activity in subcortical structures or from horizontally oriented cortical cells contribute little, if anything, to the normal scalp-recorded EEG. The factors that determine whether a cortical potential is recorded over the scalp include its voltage, the extent to which the generator cells are discharging synchronously, the area of cortex involved, and the site of cortical involvement with respect to the sulcal convolutions. Potentials arising in the sulcal depths are less likely to be recorded over the scalp by conventional EEG than are those arising at the surface. Spatial summation of cortical activity is important in producing the voltage fields that are recorded as the scalp EEG because of the attenuating properties of the skull and other interposed tissues.
The cortical activity has a regular rhythmicity that seems to depend on the functional integrity of subcortical mechanisms. It has been accepted generally that the thalamus serves as the pacemaker of certain of the cortical rhythms that are recorded during electroencephalography, but intracortical circuitries may also be involved significantly. The precise details are beyond the scope of this chapter. The physiologic basis of the abnormal rhythms that are encountered at electroencephalography is defined even less clearly. It has become apparent, however, that a cortical area of 10 to 20 cm 2 is often required to generate an interictal spike or ictal rhythm recognizable at the scalp. It also remains unclear whether it is possible to record at the scalp the EEG from sources deeper than the most superficial cortex, but source area is clearly important in this regard.
The EEG is a two-dimensional representation of three-dimensional activity that fails to provide sufficient information to allow for the unique localization of the neuronal generators of an intracranial current source (the “inverse problem”).
Alpha Activity
Alpha Rhythm
Alpha rhythm may have a frequency of between 8 and 13 Hz, but in most adults it is between 9 and 11 Hz. This rhythm is found most typically over the posterior portions of the head during wakefulness, but it may also be present in the central or temporal regions. Alpha rhythm is seen best when the patient is resting with the eyes closed. Immediately after eye closure, its frequency may be increased transiently (the “squeak” phenomenon). The alpha rhythm is not strictly monorhythmic but varies over a range of about 1 Hz, even under stable conditions. It is usually sinusoidal in configuration, may wax and wane spontaneously in amplitude, and sometimes has a spiky appearance; a spindle configuration denotes a beating phenomenon that results from the presence of two (or more) dominant frequencies.
The alpha rhythm is attenuated or abolished by visual attention ( Fig. 3-6 ) and affected transiently by other sensory stimuli and by other mental alerting activities (e.g., mental arithmetic) or by anxiety. The term paradoxical alpha rhythm refers to the appearance of alpha rhythm on eye-opening in drowsy subjects; this represents an alerting response. Alpha activity is well formed and prominent in many normal subjects but is relatively inconspicuous or absent in about 10 percent of instances. Its precise frequency is usually of little diagnostic significance unless information is available about its frequency on earlier occasions. In children, the dominant, posterior responsive rhythm reaches about 8 Hz by the age of 3 years and reaches 10 Hz by approximately 10 years of age. Slowing occurs with advancing age; as a consequence of certain medication (e.g., anticonvulsant drugs); and in patients with clouding of consciousness, metabolic disorders, or virtually any type of cerebral pathology. The alpha activity may increase in frequency in children as they mature and in older subjects who are thyrotoxic. A slight asymmetry is often present between the two hemispheres with regard to the amplitude of alpha activity and the degree to which it extends anteriorly. In particular, alpha rhythm may normally be up to 50 percent greater in amplitude over the right hemisphere, possibly because this is the nondominant hemisphere or because of variation in skull thickness. A more marked asymmetry of its amplitude may have lateralizing significance but is difficult to interpret unless other EEG abnormalities are present, because either depression or enhancement may occur on the side of a hemispheric lesion. Similarly, a persistent difference in alpha frequency of more than 1 to 2 Hz between the two hemispheres is generally regarded as abnormal. The side with the slower rhythm is more likely to be the abnormal one, but it is usually difficult to be certain unless other abnormalities are also found.

Unilaterally attenuated or absent responsiveness of the alpha rhythm sometimes occurs with lesions of the parietal or temporal lobe (Bancaud phenomenon). Asymmetric attenuation of the alpha rhythm during mental alerting procedures with the eyes closed may also be helpful for lateralizing any impairment of cerebral function.
Some normal adults have an alpha rhythm that is more conspicuous centrally or temporally than posteriorly, or has a widespread distribution. Care must be taken not to misinterpret such findings as evidence of abnormality. The so-called slow alpha variant resembles normal alpha rhythm in distribution and reactivity but has a frequency of about 4 to 5 Hz, which approximates one-half that of any alpha rhythm in the same record. This variant is of no pathologic significance.
Other Rhythms of Alpha Frequency
Not all activity having a frequency of 8 to 13 Hz is necessarily an alpha rhythm. Alpha-frequency activity that is widespread in distribution and unresponsive to external stimulation is found in some comatose patients ( p. 79 ). Temporal alpha activity is sometimes found in elderly subjects and may be asynchronous, episodic, and persistent during drowsiness. Runs of activity in the alpha range of frequencies are occasionally found frontally in children immediately after arousal from sleep (frontal arousal rhythm) and are not of pathologic significance.
Mu rhythm has a frequency that usually is in the alpha range, is seen intermittently over the central region of one or both hemispheres, is unaffected by eye-opening, and is blocked unilaterally or bilaterally by movement or the thought of movement ( Fig. 3-7 ). It is also blocked by sensory stimulation. Bilateral mu rhythm is often asynchronous and may exhibit amplitude asymmetries between the two hemispheres. The negative portions of the waves are sharpened, and the positive portions are generally rounded. Mu rhythm is often associated with a centrally located beta rhythm that is also attenuated by contralateral movement. In most instances, mu rhythm has no diagnostic significance. It is found in about 20 percent of young adults. When recorded over a skull defect, it may be mistaken for a potential epileptogenic abnormality.

Beta Activity
Any rhythmic activity that has a frequency greater than 13 Hz is referred to as beta activity. Activity of this sort is present anteriorly in the EEG of normal adults. Beta activity, responsive to eye-opening, is sometimes found over the posterior portions of the hemispheres and is then best regarded as a fast variant of the alpha rhythm . Beta activity that fails to respond to eye-opening is a common finding and usually has a generalized distribution, but in some instances it is located centrally and is attenuated by tactile stimulation or contralateral movements. It usually has an amplitude of less than about 30 μV. The amount of such activity varies considerably among normal subjects. Activity having a frequency between 18 and 25 Hz is usually more conspicuous during drowsiness, light sleep, and rapid eye movement (REM) sleep than during wakefulness. It may also be augmented by cognitive tasks. Beta activity may be induced by a number of different drugs, particularly barbiturates and the benzodiazepine compounds, but also neuroleptics, antihistaminics, d -amphetamine, methylphenidate, and cocaine. Drug-induced fast activity is typically diffuse and symmetric over the two hemispheres. Focal or lateralized spontaneous beta activity, or asymmetric drug-induced fast activity, raises the possibility of localized cerebral pathology. In such circumstances, however, it must be borne in mind that the amplitude of beta activity may be increased either ipsilateral or contralateral to a lesion involving one cerebral hemisphere; and that an amplitude asymmetry is common in normal subjects, with beta activity being up to 30 percent lower on one side than the other. Beta activity is increased in amplitude over the area of a skull defect owing to the greater proximity of the recording electrodes to the surface of the brain and the low-impedance pathway. It is reduced in amplitude over a subdural collection of fluid or localized swelling or edema of the scalp, and transiently in either a localized or lateralized manner after a focal seizure.
Generalized paroxysmal fast activity is a rare finding, occurring in less than 1 percent of EEG recordings; it may be mistaken for muscle artifact, drug effect, or sleep spindles, depending on the circumstances. In one study of 20 patients with such activity, all had seizure disorders, usually with seizures of more than one type, and most were cognitively impaired. The paroxysmal EEG disturbance occurred almost always during sleep, often with associated clinical seizures that were commonly of the tonic variety.
Theta Activity
Activity with a frequency between 4 and 7 Hz is referred to as theta activity. Theta and slower activity is usually very conspicuous in children but becomes less prominent as they mature. Some theta activity is often found in young adults, particularly over the temporal regions and during hyperventilation, but in older subjects theta activity with amplitude greater than about 30 μV is seen less commonly, except during drowsiness. Focal or lateralized theta activity may be indicative of localized cerebral pathology. More diffusely distributed theta activity is a common finding in patients with a variety of neurologic disorders, but it also may be caused by nothing more than a change in the patient’s state of arousal.
Rhythmic trains of midline theta activity , occurring especially at the vertex and having an arciform, sinusoidal, or spiky configuration, have been described as a nonspecific finding in patients with many different disorders. Such activity may be persistent or intermittent; may be present during wakefulness or drowsiness; and shows variable reactivity to eye-opening, movement, alerting, and tactile stimulation. It is not present during sleep. Its origin is uncertain.
Delta Activity
Activity that is slower than 4 Hz is designated delta activity. Activity of this sort is the predominant one in infants and is a normal finding during the deep stages of sleep in older subjects. When present in the EEG of awake adults, delta activity is an abnormal finding.
Delta activity, responsive to eye opening, is commonly seen posteriorly (intermixed with alpha activity) in children and sometimes in young adults; it is then designated posterior slow waves of youth . The spontaneous occurrence interictally of posterior, rhythmic slow waves is well described in patients with absence seizures. The slow activity has a frequency of about 3 Hz, is present during wakefulness, is responsive to eye-opening, and may be enhanced by hyperventilation. The symmetric or asymmetric occurrence of rhythmic delta activity over the posterior regions of the head after eye closure is rare. Such activity usually lasts for no more than 2 or 3 seconds and is a nonspecific finding that has been described in a number of different neurologic disorders.
Polymorphic Delta Activity
Polymorphic delta activity is continuous, irregular, slow activity that varies considerably in duration and amplitude with time; it persists during sleep, and shows little variation with change in the physiologic state of the patient ( Fig. 3-8 ). It has been related to deafferentation of the involved area of the cortex and to metabolic factors. Such activity may be found postictally and in patients with metabolic disorders. It is commonly seen, with a localized distribution, over destructive cerebral lesions involving subcortical white matter ( Fig. 3-9 ), but it generally is not found with lesions restricted to the cerebral cortex itself. It may be found either unilaterally or bilaterally in patients with thalamic tumors or lesions of the midbrain reticular formation, but its distribution in such circumstances is somewhat variable. Thus, although diffuse irregular slow activity is found over one hemisphere in some cases, in others it has a more restricted distribution.


The EEG finding of focal polymorphic delta activity correlates with the presence of focal abnormalities on neuroimaging, but nonfocal abnormalities (e.g., diffuse atrophy or cerebral edema) are sometimes found. The maximal delta focus may not always overlie the structural abnormality, even though it is in the same hemisphere. The amplitude, frequency, and distribution of focal slow waves do not relate to lesion size or to mass effect. In some patients with focal polymorphic slow-wave activity, no abnormalities whatsoever are present on CT scans; such patients usually have seizure disorders, cerebral trauma, or cerebral ischemia.
Diffuse polymorphic delta activity occurs in patients with white matter encephalopathies and following acute or extensive lesions of the upper brainstem.
Intermittent Rhythmic Delta Activity
Intermittent rhythmic delta activity is paroxysmal, has a relatively constant frequency, and is usually synchronous over the two hemispheres. It is often more prominent occipitally in children or frontally in adults ( Fig. 3-10 ), may be enhanced by hyperventilation or drowsiness, and usually is attenuated by attention. Its origin is unclear, but it probably relates to dysfunction of subcortical centers influencing the activity of cortical neurons. Its significance is considered on page 51 .

Breach Rhythm
Breach rhythm is a mu-like rhythm found in patients with skull defects after surgical operations. It has a frequency of between 6 and 11 Hz, usually with faster components, and the waves often have spike-like negative phases. The rhythm recorded parasagittally is often responsive to fist-clenching and other stimuli, whereas that recorded more laterally (at the T3 or T4 electrode) is generally unresponsive to any stimuli. The presence of a breach rhythm has no predictive value for the development of seizures or the recurrence of the intracranial pathology that necessitated the original surgery.
Lambda Waves
Lambda waves are electropositive sharp waves that may occur in the occipital region in normal subjects who are looking at and scanning something (e.g., reading) in a well-illuminated field, particularly if their attention and interest are aroused. Morphologically similar activity is sometimes seen during non-REM sleep. The nature of these potentials is unclear, and they have no known diagnostic significance at present. They are sometimes asymmetric over the two hemispheres but can be distinguished from sharp transients of pathologic significance by their response to eye closure or reduction in the level of background illumination.
Triphasic Waves
Triphasic waves typically consist of a major positive potential preceded and followed by smaller negative waves. They are found most characteristically in metabolic encephalopathies, when they usually are generalized, bilaterally synchronous, and frontally predominant. They are sometimes reactive to external (painful) stimulation. Triphasic waves were originally thought to be specific for hepatic encephalopathy, but in fact are found in a variety of metabolic encephalopathies and suggest a poor prognosis for survival. They have also been described in hypothermia, myxedema coma, neuroleptic malignant syndrome, and a variety of other neurologic disorders, including dementia. Triphasic waves may occur in patients receiving pentobarbital, especially as the drug is being tapered after treatment of status epilepticus, during which time they should not be mistaken for epileptiform activity. Similarly, they have been noted in patients with primary generalized epilepsy at a time when they had a postictal depression in level of consciousness. The periodic complexes found in certain conditions ( p. 52 ), especially Creutzfeldt–Jakob disease, may take the form of triphasic waves.
Spike Discharges
One of the major uses of electroencephalography is in the investigation of patients with suspected epilepsy. In this regard, the presence in the EEG of interictal spike discharges or sharp waves is often held to be suggestive of an epileptic disturbance. Epileptiform activity is defined as abnormal paroxysmal activity consisting, at least in part, of spikes or sharp waves resembling those found in many patients with epilepsy. It is not synonymous with an electrographic seizure , which typically consists of rhythmic repetitive activity having a relatively abrupt onset and termination, a characteristic evolution, and lasting at least several seconds. A spike is defined arbitrarily as a potential having a sharp outline and a duration of 20 to 70 msec, whereas a sharp wave has a duration of between 70 and 200 msec. The distinction between epileptiform and nonepileptiform sharp transients usually is made intuitively but bearing in mind certain guidelines. Epileptiform sharp transients are usually asymmetric in appearance, are commonly followed by a slow wave, have a duration that differs from that of the ongoing background activity, may be biphasic or triphasic, and often occur on a background containing irregular slow elements ( Fig. 3-11 ). These criteria distinguish between epileptiform activity and background activity that is sharp and variable in amplitude (e.g., a spiky alpha rhythm).

Pathologic spike discharges have different clinical implications depending on their characteristics and location. Focal epileptiform spike discharges arise from a localized cerebral region. The likelihood of spikes arising from a particular area depends on the age of the patient, type of underlying lesion, and epileptogenicity of the involved region. Slowly progressive lesions are more likely to be associated with such activity than are rapidly progressive ones, and the frontal and temporal lobes are more epileptogenic than the parietal and occipital lobes. The benign epileptiform discharges that occur in drowsy subjects ( p. 54 ) have a different significance from that of the anterior or mesial temporal spike discharges found interictally in patients with focal (partial) seizures with impaired consciousness (complex partial seizures). Similarly, 3-Hz, 6-Hz, and 1- to 2-Hz spike-wave discharges differ in their clinical and prognostic relevance. Moreover, the significance of frankly epileptiform discharges depends on the clinical circumstances in which they are found.
Epileptiform spike discharges arising focally in the temporal region are associated most commonly with focal (partial) seizures accompanied by an impairment of consciousness and are activated during drowsiness or sleep. Intermittent delta activity may also be present. Frontal epileptiform spike discharges have been associated with seizures of different types, including focal seizures with impaired external awareness and focal motor seizures, posturing, and drop attacks. Occipital discharges of very short duration may occur in congenitally blind children without seizures. In general, occipital spikes tend to be benign, although they sometimes are related to structural abnormalities or occipital seizures, especially in older persons. One form of benign epilepsy of childhood is associated with spike-wave discharges that occur in one or both occipital regions after eye closure.
Focal epileptiform spike discharges relate to paroxysmal depolarizing shifts occurring synchronously (as a result of disinhibition and excitation) in a population of neurons. Such shifts consist of a 20- to 30-mV depolarization of the cell membrane for up to about 100 msec, with a superimposed train of action potentials and followed by an afterhyperpolarization that may last for approximately 2 seconds. The slow wave that is recorded from the scalp after many epileptiform spikes (“spike-wave discharge”) has been attributed to this afterhyperpolarization. Inhibitory activity is also triggered in the surrounding cortex as well as contralaterally, and this activity may be responsible for the focal slow activity that often occurs intermittently in association with an active spike focus.
Generalized spike-wave discharges are discussed in detail in the next section.
Paroxysmal Activity
Paroxysmal activity has an abrupt onset and termination, and it can be distinguished clearly from the background activity by its frequency and amplitude. It may occur as a normal phenomenon during hyperventilation in young adults and in response to arousal or sensory stimuli during sleep. The epileptiform transients just described are one example of paroxysmal activity. Abnormal paroxysmal activity that consists, at least in part, of epileptiform sharp transients ( epileptiform activity ) has a high correlation with the occurrence of epileptic seizures. It does not necessarily represent seizure (ictal) activity, however, and scalp-recorded electrographic seizures may not contain any epileptiform activity.
Spike-Wave Activity
Generalized, bilaterally symmetric and bisynchronous 2.5- to 3-Hz spike-wave activity is the expected finding in patients with primary or idiopathic generalized epilepsy; it is enhanced by hyperventilation or hypoglycemia. More is known about the fundamental mechanisms underlying the absence seizures that occur in primary generalized epilepsy than about other types of generalized seizure. Gloor and Fariello related the generalized spike-wave activity associated with absence seizures to abnormal oscillatory discharges between cortical and thalamic neurons. This oscillation involves the regular alternation of brief periods of neuronal excitation (associated with a markedly increased firing probability) during the spike phase with longer periods of neuronal silence during the slow-wave phase. Large populations of cortical and thalamic neurons are affected in near-synchrony, and the brainstem reticular formation also participates.
Generalized 2.5- to 3-Hz spike-wave activity may also be found in secondary generalized epilepsy (i.e., in patients with generalized seizures secondary to known pathology), but it is then superimposed on a diffusely abnormal background and is often accompanied by other EEG abnormalities. In the secondary generalized epilepsies, however, slow ( p. 62 ) or atypical ( p. 63 ) spike-wave activity is found more commonly. Generalized, bisynchronous spike-wave activity rarely may arise from a unilateral cortical focus, particularly on the medial surface of the hemisphere; this phenomenon is referred to as secondary bilateral synchrony . In such circumstances, the paroxysmal activity usually has a faster or slower frequency than that in primary generalized epilepsy, and the form and relationship of the spike to the wave component of the complex are less regular. Further, a consistent asymmetry of amplitude and waveform may exist between the hemispheres, the activity being either more or less conspicuous on the affected side. Recognition of the cortical origin of such activity is facilitated when isolated focal discharges arise from one side, particularly if they consistently precede the bursts of bilaterally synchronous activity; otherwise, recognition can be difficult unless the paroxysmal discharges have a focal or lateralized onset.
The mechanisms generating secondary bilateral synchrony of spike-wave discharges are not fully established but may involve either the propagation of discharges from one hemisphere to the other along the forebrain commissures or the activation by a cortical focus of diencephalic or other midline structures, which then elicit a synchronous discharge from both hemispheres. Studies have shown diminished but persistent bisynchronous epileptiform discharges after corpus callosotomy in patients with seizure disorders, suggesting that both mechanisms may be important.
It should be noted that bilaterally synchronous spike-wave activity may also be seen in rare instances in patients with structural subtentorial or midline lesions, as well as in unselected nonepileptic patients and in the clinically unaffected siblings of patients with primary generalized epilepsy.
Generalized paroxysmal EEG disturbances, consisting of either slow activity, spike-wave activity, or sharp transients, occur in patients with a diffuse encephalopathic process involving predominantly the cortical and subcortical gray matter, but not when the white matter alone is involved. If both gray and white matter are affected, generalized paroxysmal activity occurs on a background of continuous polymorphic slow activity. Bilaterally synchronous spike-wave activity is seen in diffuse gray matter encephalopathies and is usually more slow and irregular than that in patients with primary epilepsy.
Intermittent Rhythmic Delta Activity
Intermittent rhythmic delta activity (see Fig. 3-10 ) with a frontal predominance (often designated FIRDA) in adults and an occipital emphasis in children was referred to on page 48 . It may result from a destructive lesion or from pressure and concomitant distortion affecting midline subcortical structures, in particular the diencephalon and rostral midbrain; it also occurs with deep frontal lesions. However, such activity is nonspecific and of no particular diagnostic significance. There is no means of clearly distinguishing the EEG pattern in deep midline lesions from that in diffuse cortical or subcortical encephalopathies or in metabolic encephalopathies. In most patients with FIRDA a diffuse encephalopathic process is present rather than a lesion limited to deep midline structures. FIRDA is occasionally present in otherwise healthy subjects during hyperventilation.
Burst-Suppression Pattern
The so-called burst-suppression pattern is characterized by bursts of high-voltage, mixed-frequency activity separated by intervals of marked quiescence or apparent inactivity that may last for no more than a few seconds or as long as several minutes. It occurs with a generalized distribution during the deeper stages of anesthesia; in patients who are comatose following overdosage with central nervous system (CNS) depressant drugs; and in any severe diffuse encephalopathy, such as that following anoxia ( Fig. 3-12 ). The bursts may be asymmetric or bisynchronous. The prognostic significance of a burst-suppression pattern depends on the circumstances in which it is found. When it follows cerebral anoxia, it is associated with a poor outcome. Spontaneous eye-opening, nystagmoid movements, pupillary changes, facial movements, myoclonus, and limb movements have occasionally been associated with the EEG bursts, and these sometimes mimic volitional activity. In other instances, movements occur exclusively between EEG bursts. Experimental studies in animals indicate that approximately 95 percent of cortical neurons become hyperpolarized and then electrically silent during periods of EEG suppression; this results from increased inhibition at cortical synapses, which also leads to functional disconnection of the cortex from its thalamic input.

Periodic Complexes
Repetitive paroxysmal slow- or sharp-wave discharges, or both, may occur with a regular periodicity in a number of conditions. Such periodic complexes are seen most conspicuously and with a generalized distribution in subacute sclerosing panencephalitis and Creutzfeldt–Jakob disease, and are sometimes found in patients with liver failure. Periodic complexes that exhibit, to a greater or lesser extent, a regular rhythmicity in their occurrence may also be found in patients with certain lipidoses, progressive myoclonus epilepsy, drug toxicity, anoxic encephalopathy, head injury, subdural hematoma, occasionally after tonic-clonic seizures, and in rare instances in other circumstances. Their diagnostic value therefore depends on the clinical circumstances in which they are found.
Periodic Lateralized Epileptiform Discharges
Repetitive epileptiform discharges sometimes occur periodically as a lateralized phenomenon (designated periodic lateralized epileptiform discharges , or PLEDs), although they are often reflected to some extent over the homologous region of the opposite hemisphere as well. Their amplitude has ranged between 50 and 300 μV, and their periodicity between 0.3 and 4 seconds in different series. Structural cortical or subcortical lesions often—but not always—underlie the occurrence of these discharges. They typically are seen in patients with hemispheric lesions caused, most commonly, by cerebral infarction ( Fig. 3-13 ), hemorrhage, or tumors. In patients with acute hemispheric stroke, PLEDs are more likely when there are associated metabolic derangements, especially hyperglycemia and fever. Patients with herpes simplex encephalitis may have PLEDs, especially in the first 2 weeks of the illness, but their absence in no way excludes the diagnosis. Other infections occasionally associated with PLEDs include neurosyphilis, cysticercosis, bacterial meningitis, mononucleosis encephalitis, and early Creutzfeldt–Jakob disease. PLEDs may also be found in patients with chronic seizure disorders or long-standing static lesions (e.g., old infarcts), sometimes as a persistent phenomenon but more often for a brief period, especially when seizures or toxic metabolic disturbances have occurred recently. They or more generalized periodic epileptiform discharges are found occasionally with metabolic disorders that more typically produce diffuse EEG disturbances (e.g., anoxia, hepatic encephalopathy, and abnormal blood levels of glucose or calcium), and PLEDs also have been reported with head injury, lupus cerebritis, subdural hematoma, cerebral abscess or other cystic lesions, and sickle cell disease. In many cases, no specific cause can be found.

In one series, acute stroke, tumor, and CNS infection were the most common causes of PLEDs, accounting for 26, 12, and 12 percent of cases, respectively. Acute hemorrhage and traumatic brain injury combined were responsible for another 12 percent. Other, previously unreported, causes included posterior reversible encephalopathy syndrome, familial hemiplegic migraine, and cerebral amyloidosis. In 9 cases, chronic PLEDs were related to underlying cortical dysplasia or severe remote cerebral injury, all with an accompanying partial seizure disorder. Alcohol withdrawal was sometimes a precipitant, and in a few cases was seemingly the sole cause. Seizure activity occurred in 85 percent of patients. The overall mortality rate was 27 percent.
During the acute stage of illness, patients with PLEDs are usually obtunded and commonly have seizures (especially focal seizures) and a focal neurologic deficit. The PLEDs themselves are usually an interictal pattern, being replaced or obscured by other activity as a seizure develops. They may also occur ictally, however, particularly in patients with partial seizures, status epilepticus, or epilepsia partialis continua. The intense hypermetabolism and increased blood flow revealed by positron emission tomography (PET) and single-photon emission computed tomography (SPECT) scans provide some support for the belief that this EEG pattern is sometimes ictal in nature. Moreover, patients have been described with recurrent or prolonged confusional episodes during which PLEDs were present in the EEG; with clinical improvement, the EEG normalized.
PLEDs typically occur every 1 to 2 seconds; vary in morphology in different patients; and usually (but not always) disappear over the course of a few days or weeks, to be replaced by a focal or lateralized polymorphic slow-wave disturbance or by isolated spike discharges. The underlying disease determines the prognosis of patients with PLEDs. The absence of clinical seizures when PLEDs are detected is also associated with a poor prognosis for survival.
The pathophysiologic basis of PLEDs is not understood. Some have considered PLEDs to be equivalent to the terminal phase of status epilepticus; others have reported their association with rhythmic discharges that have a stereotyped distribution, frequency, configuration, and amplitude for individual patients, and that may be obscured by the development of frank electrographic seizure discharges.
PLEDs may occur independently over both hemispheres; they are then designated BiPLEDs. The complexes over the two hemispheres differ in their morphology and repetition rate. BiPLEDs are caused most commonly by anoxic encephalopathy, multiple vascular lesions, and CNS infection with either herpes simplex or other agents, but they may also be found in patients with chronic seizure disorders or with recent onset of seizures. BiPLEDs are usually found in comatose patients and are associated with a much higher mortality than are PLEDs.
Low-Voltage Records
A number of normal subjects have generally low-voltage EEGs, consisting of an irregular mixture of activity with a frequency ranging between 2 and 30 Hz and an amplitude of less than 20 μV. A little alpha activity may, however, be present at rest or during hyperventilation, and it is sometimes possible to enhance the amplitude of background rhythms by simple or pharmacologic activating procedures. This low-voltage EEG pattern may occur on a hereditary basis. Similar low-voltage records are occasionally encountered in patients with Huntington disease or myxedema, but they are of no diagnostic value. Such records should be distinguished from those consisting primarily of low-voltage delta activity.
Other EEG Patterns
Over the years, special pathologic significance has been attributed (without adequate justification) to a number of EEG patterns that are now known to occur as a normal phenomenon in some healthy subjects, particularly during drowsiness or sleep. They do not predict the occurrence of seizures. These patterns are therefore of dubious clinical relevance, but—although recent studies suggest that their prevalence is relatively low—they merit brief comment to prevent their misinterpretation and thus to avoid misdiagnosis and unnecessary investigations. Further details are provided in Chapter 5 .
14- and 6-Hz Positive Spikes
During drowsiness and light sleep, especially in adolescents, runs of either 14- or 6-Hz positive spikes, or both, may occur, superimposed on slower waves; generally they last for less than about 1 second. They are found especially in the posterior temporal or parietal regions on one or both sides and are best seen as surface-positive waveforms on referential recordings. They are of no pathologic relevance, although bursts of such activity have been described in comatose patients with Reye syndrome and in adults with hepatic or renal disease.
Small Sharp Spikes or Benign Epileptiform Transients of Sleep
Small sharp spikes or benign epileptiform transients of sleep are found during drowsiness or light sleep in as many as one-quarter of normal adults. Generally, they consist of monophasic or biphasic spikes; they are sometimes followed by a slow wave but are unaccompanied by sharp waves or rhythmic focal slowing of the background. They usually occur independently over the two hemispheres with sporadic shifting localization, but are best seen in the anteromesial temporal regions. Their appearance varies in different patients. They are distinguished from transients of pathologic significance by their bilateral occurrence, failure to occur in trains, and disappearance as the depth of sleep increases, and by the absence of abnormal background activity. Although commonly less than 50 μV, they are sometimes larger, so that size is not a reliable distinguishing feature. Such discharges are best regarded as normal and are of no diagnostic help in the evaluation of patients with suspected epilepsy.
6-Hz Spike-Wave Activity
Brief bursts of 6-Hz spike-wave activity, usually lasting for less than 1 second, may occasionally be seen in normal adolescents or young adults, especially during drowsiness, and are sometimes referred to as phantom spike-waves. They disappear during deeper levels of sleep, unlike pathologically significant spike-wave discharges. In some instances, the discharges are bilaterally symmetric and synchronous, but in others they are asymmetric. The spike is usually small compared with the slow wave and may be hard to recognize; when the spike is large, the discharges are more likely to be of pathologic significance. Discharges that are frontally predominant are also more likely to be associated with epilepsy than are discharges that are accentuated occipitally.
Wicket Spikes
A wicket spike pattern usually is found in adults, most commonly in drowsiness or light sleep but also during wakefulness. It may consist of intermittent trains of sharp activity resembling a mu rhythm, or of sporadic single spikes that are surface-negative and essentially monophasic. Its frequency is usually between 6 and 12 Hz. Wicket spikes are generally best seen over the temporal regions, either bilaterally or independently over the two sides, and sometimes have a shifting lateralized emphasis. The spikes are not associated with a subsequent slow wave or with any background abnormality. Wicket spikes have no diagnostic significance and, in particular, do not correlate with any particular symptom complex, including epilepsy. When these spikes occur singly, however, they may be mistaken for interictal temporal spike discharges.
Rhythmic Temporal Theta Bursts of Drowsiness (Psychomotor Variant)
During drowsiness or light sleep, especially in young adults, bursts of rhythmic sharpened theta waves with a notched appearance sometimes occur, predominantly in the midtemporal regions either unilaterally or bilaterally ( Fig. 3-14 ). If bilateral, they may occur synchronously or independently, with a shifting emphasis from one side to the other. Individual bursts commonly last for at least 10 seconds. Unlike electrographic seizure discharges, the bursts of activity do not show an evolution in frequency, amplitude, or configuration. They are of no pathologic significance.

Rhythmic Theta Discharges
In patients older than about 40 years, bursts of rhythmic sharpened theta activity at about 5 to 7 Hz may occur, often with an abrupt onset and termination. In contrast to seizures, they typically show no evolution of their frequency, distribution, or configuration, and they are not followed by focal or diffuse slow activity. These subclinical rhythmic electrographic discharges in adults (known by the acronym SREDA) usually are distributed bilaterally and diffusely, but occasionally are focal or lateralized. When diffuse, the discharges are often most conspicuous over the parietal and posterior temporal regions. They can occur at rest, during hyperventilation, or with drowsiness. The bursts may last up to 1 or 2 minutes, or even longer, and may be mistaken for a subclinical seizure. Digital analysis has revealed that the bursts are maximal in the parietal or parietocentrotemporal region and that they consist of a complex mixture of rapidly shifting frequencies that show little spatial or temporal correlation. Such discharges are probably of no diagnostic relevance and do not correlate with epilepsy or any specific clinical complaints.
Unusual variants of SREDA include predominantly delta frequencies, notched waveforms, or discharges having a frontal or more focal distribution; bursts that are more prolonged in duration; and discharges that occur during sleep.
EEG Changes with Aging
A number of EEG changes occur with senescence, but the extent to which they occur varies widely in different subjects. It is widely believed that the mean alpha frequency slows in elderly subjects compared with young adults, but whether such slowing occurs in completely healthy and cognitively intact elderly subjects is unclear; if it does, it is to a minimal degree. In subjects older than 50 years, alpha-like activity is sometimes seen in one or both temporal regions and may be more conspicuous than the occipital alpha rhythm.
The effect of aging on beta activity is less clear, and review of the published accounts is confounded by differences in the methods of analysis and recording circumstances, differences in the types of patients studied, and a failure to consider medical and drug histories and the state of intellectual function. No consistent effects on beta rhythms have been found in elderly subjects, although various alterations have been described by different authors.
Diffuse theta and delta activity is significantly increased in elderly persons and is clearly related to intellectual deterioration and life expectancy. Polymorphic or rhythmic focal slow activity, encountered fairly commonly in the elderly, usually is localized to the left anterior temporal region. Such slow activity may be enhanced or brought out during drowsiness or hyperventilation. It is of little pathologic significance, showing no obvious correlation with life expectancy, neurologic disease, or intellectual changes. It should not be regarded as abnormal if it occurs infrequently in bursts on an otherwise normal background; however, the bounds of normality are unclear.
EEG sleep patterns also change with age, with a reduction in total sleep time and especially in the duration of stage 3 and 4 sleep.
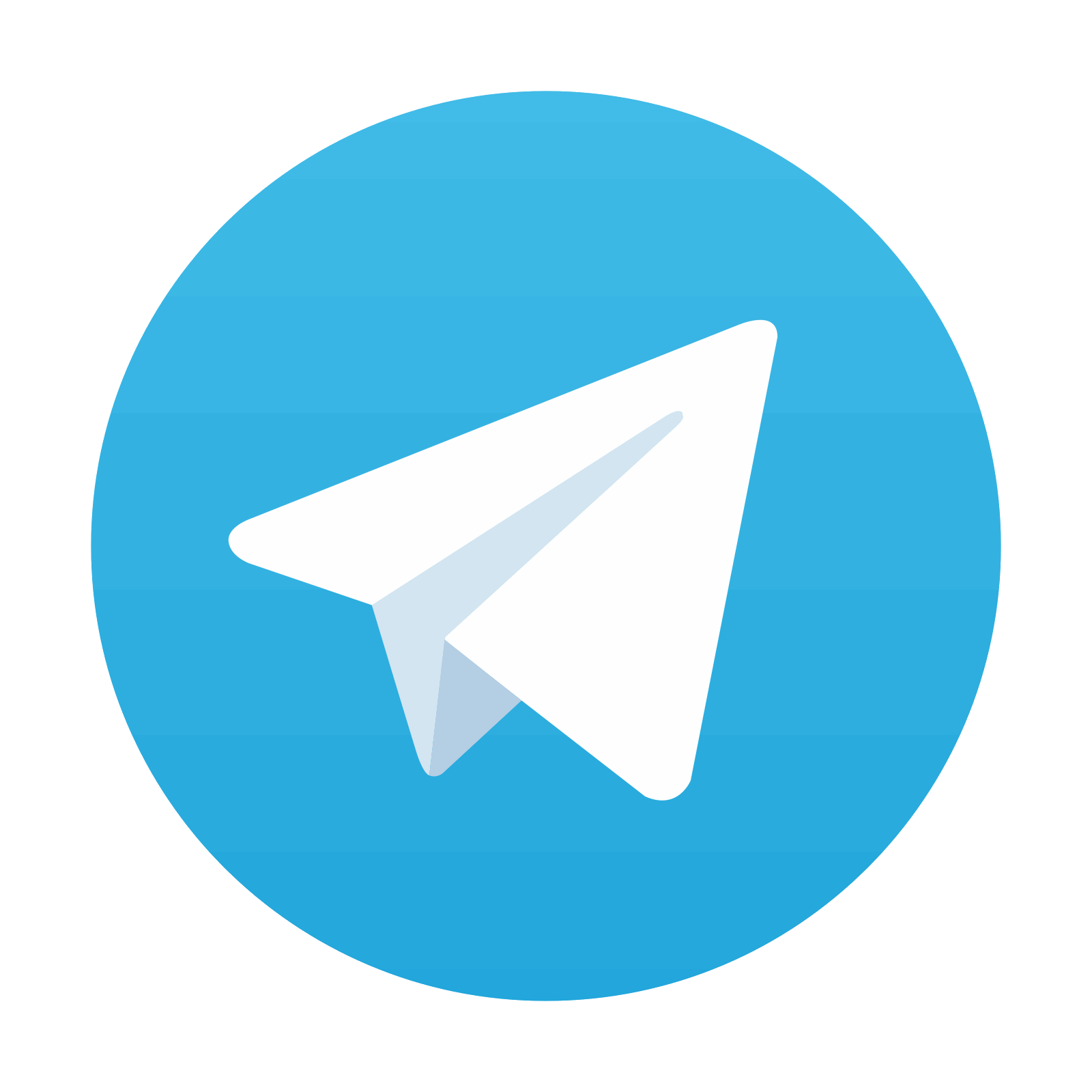
Stay updated, free articles. Join our Telegram channel

Full access? Get Clinical Tree
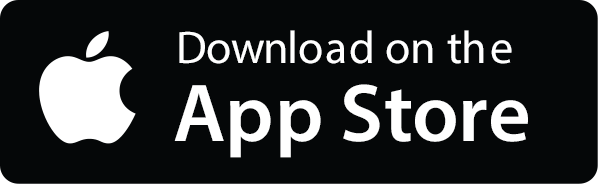
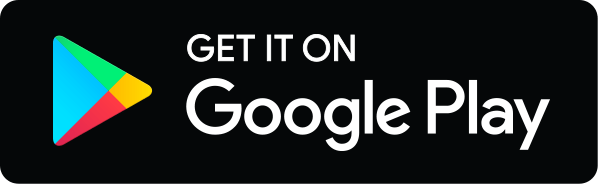