Traditionally, death was defined in medicine as the permanent cessation of heartbeat and respiration. When the loss of these functions is not reversed promptly by resuscitative measures, profound and irreversible pathologic alterations occur in the brain within minutes under normothermic conditions. The increasing effectiveness of resuscitative techniques and life-support systems has restored and artificially maintained cardiovascular and respiratory functions in countless individuals. However, the extreme vulnerability of the brain to anoxia, which exceeds that of other systems of the body, remains the major factor limiting a favorable outcome of resuscitation. In patients who have suffered a sufficiently prolonged arrest, restoring and maintaining pulmonary, cardiac, and other functions does not lead to recovery of conscious behaviors and higher mental faculties that represent the very essence of human life. Because preservation of other organs is inconsequential in the face of complete and permanent abolition of brain function, these states of coma dépassé (a state beyond coma) or brain death have been equated with death of the person by medical, legal, and religious authorities. It has been recognized that the use of extraordinary measures to support bodily functions in individuals with dead brains demands an unreasonable expenditure of human and financial resources, unnecessarily prolongs the sorrow and grief of relatives and friends, and is contrary to the individual’s right to die with dignity. The rapid progress of organ transplantation surgery provides further impetus to redefining death of the individual as death of the brain because the success of such surgery depends on the use of viable organs removed from a patient with permanently abolished brain function before the circulation fails.
Definition and neuropathology of brain death
Two opposing concepts of death by neurologic criteria exist. In the United States, death by neurologic criteria is defined as “irreversible loss of function of the brain, including the brainstem,” whereas in the United Kingdom it is characterized as an “irreversible cessation of brainstem function.” That irreversible cessation of brain function involves the whole brain is demonstrated irrefutably by electrophysiologic, i.e., electroencephalographic (EEG) and evoked potential tests, brain blood-flow studies, and pathologic findings. However, the opposing views of brain death expressed by British and American students of this condition are not irreconcilable; no fundamental contradiction appears to exist between the concept that brain death is characterized by the irreversible loss of function of the whole brain, including the brainstem, and the belief that permanent abolition of brainstem function “is the physiologic kernel of brain death, the anatomic substratum of its cardinal signs (apnoeic coma with absent brainstem function) and the main determinant of its invariable cardiac prognosis: asystole within hours or days.” This last observation has been challenged by Shewmon, although not without debate.
As the concept of brain death evolved, so did knowledge of the neuropathologic changes characterizing this condition. Early work suggested that marked edema, extensive softening, necrosis (particularly in the gray matter), and severe neuronal alterations without inflammatory reaction or vascular thrombosis characterized the brains of individuals who had been in coma dépassé and artificially ventilated. However, subsequent research revealed a greater diversity of findings in individuals with brain death maintained on a respirator for variable periods. Most commonly, swelling and softening as well as transtentorial herniation, hemorrhage, infarction, and necrosis were found in the cerebrum, cerebellum, and brainstem of individuals with brain death. These alterations varied considerably in extent, severity, and distribution. In addition, the brains of some patients who died within about 24 hours after the onset of coma and apnea displayed minimal or no pathologic abnormalities, thus indicating that some time must elapse after the onset of coma and apnea for the changes of the “respirator brain” to develop.
Brain death defined as irreversible loss of function of the whole brain must be distinguished from:
- 1.
Cortical death, characterized by acute failure of forebrain with variable preservation of brainstem function
- 2.
Primary brainstem death, defined as acute loss of brainstem function with substantial preservation of cortical function.
Clinical diagnosis of brain death in adults
Acceptance of the notion of brain death, and identification of this condition with death of the individual, required operational criteria for diagnosing this state in persons with artificially sustained cardiac and respiratory functions. The definition of these standards was intended to help physicians identify the circumstances under which continuation of extraordinary supportive measures was both unnecessary and inadvisable, as well as to protect patients against premature termination of these efforts. Following earlier formulations, guidelines for determining brain death in adults were published by the American Academy of Neurology.
Prerequisites for Applying the Criteria of Brain Death
Certain conditions must be satisfied before the diagnosis of brain death is considered, including:
- 1.
Demonstration by clinical and neuroimaging studies that an acute central nervous system (CNS) catastrophe has occurred that is compatible with brain death. Brain death from primary neurologic disease is often the result of severe head injury, aneurysmal subarachnoid hemorrhage, orintracerebral hemorrhage. In intensive care units (ICUs), hypoxic-ischemic encephalopathy after prolonged cardiac resuscitation or asphyxia, large ischemic strokes associated with brain swelling and herniation, and massive brain edema in patients with fulminant hepatic failure are the most common causes of brain death.
- 2.
Exclusion of conditions that may reversibly produce or may contribute to cause clinical manifestations mimicking brain death, including:
- a.
Hypothermia . Prerequisite core temperature must be equal to or greater than 36 °C or 96.8 °F
- b.
Drug intoxication or poisoning , including the effects of sedatives, aminoglycoside antibiotics, tricyclic antidepressants, anticholinergics, antiepileptic drugs, and chemotherapeutic agents or neuromuscular blocking drugs causing total paralysis
- c.
Profound hypotension . Prerequisite systemic blood pressure must be equal to or greater than 100 mm Hg
- d.
Severe metabolic (electrolyte and acid-base) derangements and endocrine crises .
- a.
Clinical Observations that Establish Brain Death
Provided all prerequisites are satisfied, the following clinical findings establish the diagnosis of brain death :
- 1.
Unresponsiveness, defined as the absence of motor responses of the limbs and lack of facial grimacing to noxious stimuli such as pressure on the supraorbital ridge, the nail bed, or the temporomandibular joint.
- 2.
Absent brainstem reflexes, including pupillary, oculocephalic, oculovestibular, corneal, jaw, pharyngeal, and tracheal reflexes.
- 3.
Apnea demonstrated by a strictly standardized test that entails interrupting artificial ventilation long enough to allow the PaCO 2 to rise to levels producing maximal stimulation of the brainstem respiratory center while providing adequate oxygenation. This level usually is specified as 60 mm Hg in North America and 50 mm Hg in the United Kingdom. However, since the pH, not the PaCO 2 , is the major determinant of respiratory drive, the observer should ensure that the arterial pH declines to 7.3 or lower during the apnea test.
Some potentially misleading clinical observations that do not invalidate the diagnosis of brain death are detailed in the guidelines of the American Academy of Neurology.
Induced hypothermia for the treatment of patients in coma after cardiac arrest does not alter these criteria, but they can be applied only after the patient’s core temperature has been returned to normothermia. During the period of induced hypothermia, the usual prognostic significance of the clinical and electrophysiologic variables used to predict death or survival should not be used, with the possible exception of the median nerve somatosensory evoked response.
Confirmatory tests in adults
The guidelines of the American Academy of Neurology affirm that brain death is a clinical diagnosis. However, the guidelines recognize that in some circumstances specific components of the clinical examination cannot be tested or evaluated reliably. These conditions include severe facial trauma; pre-existing pupillary abnormalities; instability of the cervical spine; petrous bone fracture; and toxic blood levels of sedative drugs, aminoglycosides, tricyclic antidepressants, anticholinergics, antiepileptic drugs, chemotherapeutic agents, or neuromuscular blocking drugs. When these conditions exist, laboratory tests are desirable but are not required. Prolonged clinical observation, sometimes over a period of days, may be necessary to establish by clinical means that irreversible loss of brain function has occurred. This approach appears to be justified only when the instrumentation and human skills required for ancillary studies are not available.
In the author’s opinion, when the diagnosis of brain death is in doubt, no effort should be spared to decrease the chance of error, expedite the diagnosis, and provide objective proof of irreversible extinction of brain function by using appropriate confirmatory procedures. At present, these methods primarily include: (1) electrophysiologic methods; and (2) tests of cerebral blood flow. In most of the United States, the choice of the confirmatory test is left to the discretion of the physician, consideration being given to the laws of the individual states and to institutional directives. Some jurisdictions require specific tests by law, requiring that they be performed even when the history and clinical examination are conclusive. Because confounding conditions (e.g., the administration of high doses of sedative drugs, sometimes combined with neuromuscular junction blocking agents) are increasingly common in ICUs, the contribution of laboratory tests to the determination of brain death has acquired special significance. This chapter examines the role of electrophysiologic testing in the confirmation of suspected brain death.
The Electroencephalogram
Electrocerebral Inactivity: Definition
In a substantial proportion of patients with clinically diagnosed brain death (as many as 80 percent in a report by Grigg and associates ), EEG recording shows a pattern of “electrocerebral inactivity” (ECI) defined as “absence over all regions of the head of identifiable electrical activity of cerebral origin, whether spontaneous or induced by physiological stimuli and pharmacological agents,” and electrical activity of cerebral origin is identified when it exceeds an assumed instrumental noise of 2 μV ( Fig. 35-1 ). The use of nonphysiologic terms to describe ECI, such as “electrocerebral silence” (ECS), “isoelectric,” “flat,” and “null” EEG, is discouraged.

Early cortical and depth recordings in patients who satisfied all prerequisites and clinical criteria of brain death demonstrated that ECI in scalp EEGs was associated with absence of electrocerebral activity at all cortical and subcortical sites explored, indicating global loss of brain function.
As defined, ECI excludes the following:
- 1.
Records displaying a “burst-suppression” pattern that consists of “bursts of theta and/or delta waves, at times intermixed with faster waves, and intervening periods of low amplitude” that commonly are found in conditions such as severe hypoxic-ischemic encephalopathies, acute intoxication with CNS depressants, deep hypothermia, late-stage status epilepticus, and general anesthesia. In patients who are drug-intoxicated, the EEG activity may be reduced to isolated waves separated by apparently quiescent intervals lasting several minutes, but may subsequently recover ( Fig. 35-2 ).
Figure 35-2
Electroencephalogram (EEG) of a 30-year-old woman with secobarbital overdose. A , On the second day of hospitalization she was unresponsive to all stimuli and had apnea, no brainstem reflexes, constricted unreactive pupils, and a blood barbiturate level of 36 mg/100 ml. Her EEG showed pseudoperiodic sharp-and-slow waves more prominent on the right than left hemisphere, with intervening periods of “suppression” lasting up to 22 seconds. B , On the following day she was clinically unchanged, and demonstrated bilateral asymmetric sharp waves repeating at 1 to 2 Hz. C , Four days after admission, she withdrew to noxious stimuli and her pupils reacted to light stimulation. High-voltage 2- to 3-Hz waves occurred bilaterally in her EEG. D , Eight days later she was alert, followed commands, and had an EEG displaying a 9- to 10-Hz posterior rhythm attenuated by eye opening. The patient recovered without neurologic sequelae.
- 2.
Low-voltage, slow EEGs primarily consisting of delta and theta activity generally unreactive to stimuli such as are demonstrated in deeply comatose or brain-dead patients with widespread brain damage.
- 3.
Low-voltage waking EEGs that are “characterized by activity of amplitude not greater than 20 μV/mm over all head regions.” Using high instrumental sensitivities, these EEGs are shown to be composed of beta, theta, and (to a lesser degree) delta waves, with or without alpha activity over the posterior areas. These recordings “are susceptible to change under the influence of certain physiological stimuli, sleep, pharmacological agents and pathological processes.”
- 4.
EEGs showing no detectable electrocerebral activity, but recorded only over limited areas of the scalp.
Demonstration of Electrocerebral Inactivity
Demonstrating ECI in patients with suspected brain death requires rigorous recording technique. The EEG examination should begin with a brief recording using a montage and the instrumental settings utilized in ordinary EEG recording. If this preliminary sampling produces either no evidence or questionable evidence of activity of cerebral origin, the technique should be modified promptly to demonstrate ECI according to stringent standards. These technical requirements (summarized in Table 35-1 ) are in substantial harmony with the guidelines of the American Clinical Neurophysiology Society.
|
Artifacts (electrical potentials generated by extracerebral sources) commonly contaminate EEG records designed to demonstrate ECI. These spurious electrical events include potentials generated by instruments close to or connected with the patient, such as electrocardiographic (ECG) monitors, pacemakers, warming blankets, and dialysis units, as well as biopotentials of cardiac, muscular, and respiratory origin. These have been described and illustrated in detail elsewhere.
The most common and disturbing of all biologic artifacts is the ECG (see Fig. 35-1 ). ECG potentials are especially prominent in ear-reference montages. They occasionally resemble sharp-and-slow-wave complexes or triphasic waves. Premature ventricular depolarizations or ventricular tachycardia may produce potentials that appear on the scalp as sharp transients and theta or delta rhythms, respectively. In addition, rhythms of mostly alpha frequency occasionally result from head vibration caused by the systolic pulse wave (ballistocardiogram). Disconnecting existing ECG monitors, repositioning the patient’s head, and selecting montages less prone to ECG pickup may reduce, but generally do not eliminate, the ECG artifact. Thus, having attempted these and other maneuvers, the technologist can generally do little but acknowledge the presence of this artifact and try to prove its origin by monitoring an ECG lead simultaneously with the scalp activity (see Fig. 35-1 ).
Electromyographic (EMG) potentials may obscure possible electrocerebral activity unless nondepolarizing neuromuscular blocking agents such as vecuronium or cisatracurium are given. However, caution is suggested by a report of cardiac arrest and death following administration of the depolarizing agent succinylcholine to reduce myogenic artifacts, as this agent rarely may produce severe hyperkalemia.
Respiration-related artifacts may pose special problems. Delivery of a bolus of gas to the patient through flexible tubes may cause vibration of the head, resulting in rhythmic activity of alpha or other frequencies. Other head movements associated with respiration may generate large transients resembling periodic paroxysmal discharges. Monitoring respiration with appropriate transducers helps to determine the origin of these artifacts. On occasion it may even be necessary to stop the respirator briefly to assess questionable activity. However, if this disconnection test must be carried out over minutes, adequate oxygenation must be provided to avoid additional anoxic damage.
Identifying beyond doubt and eliminating or reducing and monitoring all sources of artifact are time-consuming tasks that often seriously challenge the competence, ingenuity, and determination of the EEG technologist. Preparing with special prudence the patient with suspected brain death for EEG monitoring, and sometimes interrupting the EEG examination to allow the performance of essential therapeutic maneuvers, often cause additional delays. Thus, the production of at least 30 minutes of interpretable recording satisfying current technical requirements may take as long as 2 hours and sometimes longer.
Determination that Electrocerebral Inactivity is Irreversible
The EEG pattern of ECI indicates loss of cerebral function but does not imply that this loss is permanent. This pattern must be regarded as potentially reversible in the presence of those same conditions that also transiently cause clinical manifestations mimicking brain death (e.g., intoxication with CNS depressants, hypothermia, profound hypotension, and severe metabolic and endocrine disorders). When these potentially confounding conditions are excluded, ECI is highly likely to be irreversible and confirms the diagnosis of brain death.
CNS Depressant Drugs
Most reports of ECI persisting for 24 hours or longer in patients intoxicated with CNS depressants described EEGs that probably were not electrocerebrally inactive by current standards. The EEGs of these patients more commonly demonstrate prolonged periods of markedly diminished EEG activity rather than ECI (see Fig. 35-2, A ). Major reversible decrease in EEG activity also characterizes patients treated in the ICU with high doses of barbiturates, sometimes combined with neuromuscular blocking agents and hypothermia.
Hypothermia
ECI occurs at a mean core temperature of 24 °C ; however, this pattern does not occur at the body temperatures that usually are encountered in the ICU. EEG activity recovers in hypothermic individuals with viable brains who are warmed to normal temperature. Profound reversible depression, but not ECI as currently defined, has been reported in a patient with hyperthermia (rectal temperature of 42.5 °C).
Profound Hypotension
EEG activity may be abolished by cardiovascular shock with consequent low cerebral perfusion pressure and may be restored when blood pressure is raised above shock levels. However, because patients are treated routinely in ICUs with intravenous volume expansion and vasopressors, hypotension generally does not play a major role in causing ECI.
Severe Metabolic and Endocrine Disorders
Severe metabolic and endocrine disorders (e.g., profound abnormalities of serum electrolytes, acid–base balance, and blood gases) and illnesses caused by severe dysfunction of the liver, kidney, and pancreas may also contribute to the generation of ECI.
Persistence of Electroencephalographic Activity
A substantial proportion of individuals who satisfy all preconditions and clinical criteria of brain death still demonstrate persistent electrocerebral activity in their EEGs several hours to several days after death of their brains has been diagnosed clinically. Grigg and associates observed this activity in 12 of 56 patients examined 2 to 168 hours after the diagnosis of brain death was established clinically, and characterized it as follows:
- 1.
Low-voltage theta or beta activity that occurred in 9 patients (16 percent) whose brains demonstrated hypoxic alterations involving diffusely the cerebral cortex, basal ganglia, brainstem, and cerebellum ( Fig. 35-3 )
Figure 35-3
This electroencephalogram was recorded on a 35-year-old man 32 hours after the diagnosis of brain death was made. Low-voltage 5- to 7-Hz activity was intermixed with some beta potentials and scattered delta waves. DPR, “dummy patient” resistor.
(From Grigg MM, Kelly MA, Celesia GG et al: Electroencephalographic activity after brain death. Arch Neurol, 44:948, 1987, with permission.)
- 2.
Theta and delta waves intermixed with 10- to 12-Hz spindle-like potentials that were found in 2 individuals with extensive ischemic necrosis of the entire brainstem and cerebellum, with relative sparing of the cerebrum
- 3.
Monotonous, unreactive, anterior-predominant activity of alpha frequency that was recorded in 1 patient in whom no autopsy was performed.
The presence of persistent electrocerebral activity and the pathologic alterations associated with this EEG finding indicate that relatively preserved areas of brain tissue in otherwise nonfunctional brains may still be capable of generating EEG potentials in individuals who are clinically brain dead. In harmony with this contention, pathologic studies have shown that residual EEG activity is most common among individuals whose brain shows only patchy swelling, edema, infarction, and necrosis, whereas more extensive necrosis, swollen brain, and cerebral herniations are especially common among patients with ECI. Individuals with EEGs classified as equivocal demonstrate pathologic changes intermediate between these two groups. The slow activity intermixed with spindle-like potentials sometimes found in the EEG of patients with extensive necrosis of the brainstem and cerebellum appears to be characteristic of “acute failure of brainstem function.”
In five of six brain-dead individuals with preserved EEG activity studied by Grigg and colleagues, radioisotope scintigraphy demonstrated absent cerebral perfusion. This finding adds substance to the belief that persistent electrocerebral activity in patients with clinically established brain death does not portend lasting survival and any form of residual sentience. However, how much residual EEG activity is compatible with irreversible loss of brain function has not been determined. Thus, hesitant interpretations are justifiably common when electrocerebral activity is detected in patients with suspected brain death.
Computer Analysis of the Electroencephalogram
Attempts have been made to use computer techniques to analyze the EEGs of patients with suspected brain death in the hope of facilitating and increasing the objectivity and reliability of their interpretation. The earliest and most ambitious of these undertakings was the design by Bickford and co-workers of a central computer facility capable of analyzing EEGs transmitted via telephone to the center from any of a number of hospitals and automatically generating estimates of “brain output” as well as verbal interpretations. Limitations and fallacies of this method were recognized, and telephone transmission of EEGs to determine ECI was discouraged. However, continuous monitoring and quantification of electrocerebral activity from limited areas of the scalp, pioneered by Prior and Maynard, can signal the onset of brain death and suggest the need for a more complete EEG or other study.
Suitability of the Electroencephalogram as a Confirmatory Test of Brain Death in Adults
The EEG has long enjoyed the status of a test of choice for the confirmation of clinically diagnosed brain death. Factors that account for this pre-eminent role included the noninvasive and safe nature of the test, its feasibility at the bedside in the ICU, its well-established technique and modest cost, the strong statistical association of ECI with brain death, and the high reproducibility of this pattern in successive recordings in the absence of the reversible conditions already alluded to in this chapter. However, the EEG suffers from major limitations and poses technical and interpretive problems that substantially diminish its utility as a confirmatory test of irreversible loss of brain function. These difficulties include the following:
- 1.
The EEG tests cortical but not brainstem function.
- 2.
EEG recording of patients suspected of having dead brains is not available around the clock in many hospitals in the United States.
- 3.
EEGs obtained in the ICU according to stringent technical standards, including high sensitivities (see Table 35-1 ), are difficult and time-consuming tests.
- 4.
Some EEGs obtained in these circumstances contain irreducible artifacts that hamper their interpretation.
- 5.
ECI can be caused by reversible conditions that hamper the clinical examination (i.e., in those same circumstances in which laboratory confirmation is most needed).
- 6.
EEG activity persists in a substantial proportion of clinically brain-dead patients even in the face of absent measurable brain perfusion.
- 7.
ECI does not differentiate between (whole) brain death and “cortical death” with substantially preserved brainstem function.
- 8.
Recordings obtained to confirm clinically suspected brain death must be interpreted by physicians with special training in clinical neurophysiology and experience with recordings in the ICU.
- 9.
Inter- and intra-interpreter disagreements occur even among qualified interpreters.
The author believes that, because of these shortcomings, limitations, and pitfalls, relying on the EEG as the confirmatory test of choice is not justified. This view is not accepted universally. In several countries in Europe, Central and South America, and Asia, the demonstration of an EEG showing ECI is mandated by law for the certification of brain death. In France, two EEGs showing ECI at least 4 hours apart or an angiogram showing absence of intracranial circulation similarly is required. Existing guidelines of the American Academy of Neurology suggest confirmatory tests only in those circumstances in which the diagnosis of brain death is suspected but cannot be established fully by the clinical examination. In Canada, the EEG is not recommended as a confirmatory test. Confirmation of brain death by EEG as well as other laboratory tests is neither required nor recommended in the United Kingdom.
Evoked Potentials
Computer-averaged evoked potentials (EPs) elicited by somatosensory, auditory, and visual stimuli offer an alternative to or may complement the EEG in the confirmation of clinically suspected brain death.
Somatosensory Evoked Potentials
Recording Methodology, Components, and Postulated Origin
In normal subjects, short-latency somatosensory evoked potentials (SEPs) to electrical stimulation of the median nerve at the wrist consist of a sequence of waves that are generated by the ascending volley at progressively higher levels of the somatosensory pathway from the sensory periphery to the cerebral cortex. Because appropriate technique is critically important for the recording of SEPs, the following minimal montage was recommended by the American Clinical Neurophysiology Society :
Channel 1: CPc–CPi (contralateral to ipsilateral centroparietal)
Channel 2: CPi–EPc (ipsilateral centroparietal to contralateral Erb’s point)
Channel 3: C5S–EPc or AN (C5 spine to contralateral Erb’s point or anterior neck)
Channel 4: EPi–EPc (ipsilateral to contralateral Erb’s point).
SEP components most relevant to the assessment of brain death are designated N9, N13, P14, N18, and N20 ( Fig. 35-4 ). Reviews published elsewhere and Chapters 26 and 27 in the present volume analyze the features, topography, and putative generators of individual response components. Although the specific identity of these generators is still controversial, the following broad notions provide a framework for interpreting SEPs in brain-dead patients. The N9 potential recorded at Erb’s point (see Fig. 35-4, A , channel 4) is generated by the brachial plexus afferent volley (“brachial plexus” or “peripheral” potential). The N13 peak detected over the fifth cervical spinous process (C5S; see Fig. 35-4, A , channel 3) reflects postsynaptic activity in central gray matter of the cervical cord (“spinal” or “cervical” potential). The P14 component ( Fig. 35-4 , channel 2) is widely distributed over the scalp with a frontal maximum, and is demonstrated by scalp–noncephalic reference derivations (e.g., CPi–EPc or Fz–EPc) but not by scalp-to-scalp recordings. The designation “P14” is used commonly in the literature to describe the “P13–P14 complex.” This complex consists of two potentials, P13 and P14, which cannot always be differentiated clearly. Observations in normal subjects and individuals with cervicomedullary or high cervical cord lesions indicate that P13 and P14 are distinct potentials with different generators; P13 is generated in high segments of the cervical cord, whereas P14 arises in the low brainstem close to the cervicomedullary junction. Because of its widespread acceptance, the designation P14 is used in this review to describe the P13–P14 complex, but any reference to P14 as a “brainstem” potential should be interpreted as applying solely to the P14 component of the P13–P14 complex. An earlier, variably identifiable P11 potential preceding P13 is believed to reflect the ascending volley in the fibers of the dorsal column at the cervical level. The long-lasting N18 potential has a wide distribution on the scalp similar to that of the P14 and is demonstrated best by scalp–noncephalic reference derivation (CPi–EPc or Fz–EPc) (see Fig. 35-4, A , channel 2). This potential is believed to reflect postsynaptic activity in brainstem gray matter structures. As opposed to P14 and N18, the N20 is localized to the parietal region contralateral to the limb stimulated and represents the first response of the primary somatosensory cortex to the ascending volley (“cortical” potential) (see Fig. 35-4, A , channel 1).

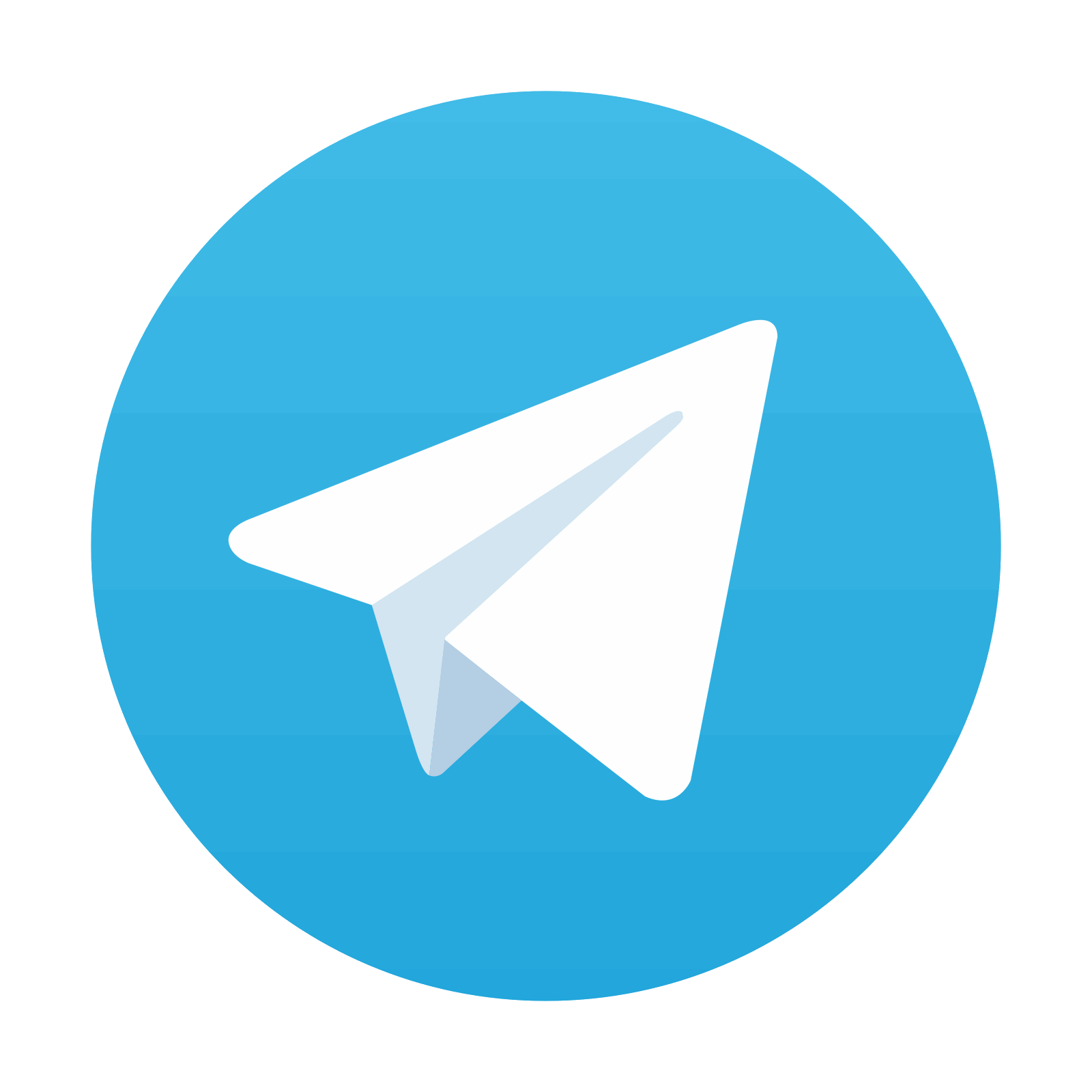
Stay updated, free articles. Join our Telegram channel

Full access? Get Clinical Tree
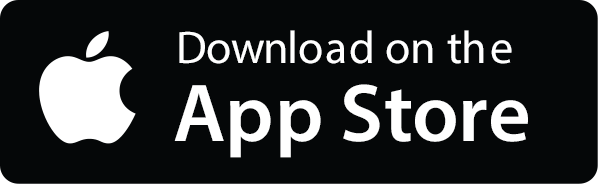
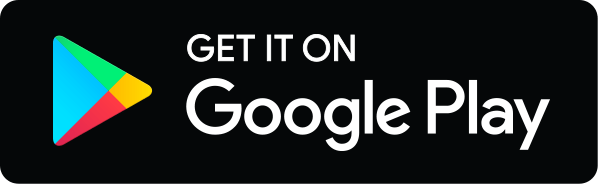
