Despite challenges, electrophysiologic studies can be performed readily in the intensive care unit (ICU) in a sensitive, reliable, and quantifiable manner. They assess nervous system functions that are clinically inaccessible. For example, most cases of septic encephalopathy or critical illness polyneuropathy cannot be confirmed without electrophysiologic studies. , Treatable conditions such as nonconvulsive status epilepticus and myasthenia gravis may be disclosed. The prognosis of anoxic-ischemic encephalopathy or neuromuscular conditions causing difficulty in weaning from the ventilator is determined more readily. Various tests provide objective methods of monitoring the course of disease and the effects of therapy. Newer techniques, notably those testing the control of respiration, will enhance the value of neuroelectrophysiology in the future and offer new avenues of research. These studies can be applied in adult, pediatric, neonatal, and neurologic-neurosurgical ICUs.
Role of electrophysiologic tests
Diagnosis
Electroencephalography (EEG) assists in the diagnosis of certain specific conditions such as nonconvulsive status epilepticus, herpes simplex encephalitis, and barbiturate intoxication. A normal EEG with reactivity to passive eye opening in an apparently obtunded patient raises the possibility of psychogenic unresponsiveness or locked-in syndrome from a brainstem lesion or neuromuscular paralysis. More often, disease categories can be classified only broadly. The EEG patterns can differentiate cortical gray matter involvement (mild slowing and spikes) from white matter (delta activity) and combined cortical and subcortical nuclear involvement (periodic discharges, as in some cases of anoxic encephalopathy). Typical triphasic waves or frontally predominant, generalized rhythmic delta activity on a slowed background is strongly suggestive of a metabolic encephalopathy but not specific to one disease process.
Electrophysiologic studies of the limbs and respiratory structures are indicated when a neuromuscular cause of respiratory insufficiency is suspected or when focal or generalized limb weakness and reduced deep tendon reflexes are present. Even in the absence of these signs, if the patient has established sepsis for more than 3 weeks, the tests should be performed. Localization within the peripheral nervous system is usually possible. Polyneuropathies, mononeuropathies, neuromuscular transmission defects, and myopathies usually can be distinguished, and neuropathies can be subclassified into axonal and demyelinating varieties. Specific patterns in certain disorders allow for more precise diagnoses. When electrophysiologic features are combined with clinical information and (in selected cases) neuroimaging, serum creatine kinase determination, and muscle biopsy, the differential diagnosis can be narrowed further.
Assessment of Disease Course and Effects of Therapy
Continuous EEG (CEEG) monitoring is of great value in the ongoing management of patients with unstable but potentially reversible conditions such as status epilepticus. In conditions associated with decreased perfusion (e.g., stroke, vasospasm, and microvascular compression from mass effect), the EEG reveals frequency and amplitude changes caused by ischemia before irreversible tissue damage occurs. The benefit of therapeutic maneuvers such as induced hypertension in cases of vasospasm can be monitored. In routine management, CEEG can be used for monitoring the depth of sedation with, for example, midazolam or other sedative drugs. This application of CEEG is especially useful for paralyzed patients. Because excessive sedation prolongs length of stay in the ICU, CEEG may be useful for ensuring optimal utilization of resources.
Electrophysiologic studies of the limbs and respiration should be performed early and then repeated. Such studies often establish the diagnosis and indicate the course of the disorder, thereby allowing a prognostic estimate of recovery and expected length of stay in the ICU.
Prognosis
With central nervous system (CNS) disorders, the neurologic or electrophysiologic determination of prognosis is valid only for conditions that are capable of causing neuronal death. (Some systemic disorders, such as sepsis, multiple organ failure, or intoxication, may compromise CNS function in a reversible manner.) Determination of the prognosis of comatose patients with intact brainstem function requires months of clinical evaluation. Electrophysiologic tests can, in some circumstances, establish a reliable prognosis at an early stage. In anoxic-ischemic encephalopathy, a “flat” or diffusely suppressed EEG is strongly suggestive of a prognosis no better than a permanent vegetative state. Invariant or deteriorating patterns, provided that no confounding elements such as sedatives and sepsis are present, are also strongly supportive of a poor prognosis. , Somatosensory evoked potentials (SEPs) are objective and quantified. The early components from activation of the primary somatosensory cortex, especially the N20 response to median nerve stimulation, are very robust. If peripheral nerve and spinal cord components (as well as subcortical waves) are present, but the cortical component is absent, either severe cortical damage or disconnection from subcortical sites is likely.
Research
The ICU provides opportunities for clinical research that have been neglected by clinical neurophysiologists. Valuable contributions in patient care guidelines should result from research in the ICU. Much future effort will be spent in the execution of controlled studies that examine the usefulness of various electrophysiologic investigations or monitoring systems in different conditions and with varying severity of illness. Guidelines should be formulated through an evidence-based approach. In clinical research, the classification of electroclinical phenomena should use strategies with high intrarater and interrater reliability.
Initial clinical evaluation
A careful clinical evaluation is essential to allow the clinician to pose suitable questions to be addressed by electrophysiologic testing. The clinical neurophysiologist must be aware of the key issues to plan appropriate investigations.
Clinical assessment of ICU patients is often difficult. Commonly, a history cannot be obtained because the patient has impaired consciousness or an endotracheal tube is in place. Relatives are often absent, and charts are voluminous. Sedating and paralyzing drugs and numerous lines and catheters further hamper clinical assessment. Nonetheless, as much clinical information as possible should be obtained. Relatives can be contacted by telephone; residents and bedside nurses can provide helpful information. Details of the admitting illness and past history (including drugs and previous illnesses) should be noted. In addition, details of current therapy, including procedures such as insertion of Swan–Ganz or aortic catheters, drugs (especially sedatives, antibiotics, aminophylline, vasopressor agents, corticosteroids, and neuromuscular blocking agents), and surgical procedures of the head, neck, chest, or abdomen are often relevant. One should look for evidence of sepsis and multiorgan failure and for episodes of significant hypotension or hypoxemia. Laboratory data, including neuroimaging, are then reviewed.
The physical examination is often limited. The patient should be observed for spontaneous movement and patterns of respiration before assessment of reactivity to increasing stimulation, beginning with auditory, then tactile, and then noxious stimuli to the limbs and face. Examination of most of the cranial nerves, tendon reflexes, and plantar responses is usually feasible unless the patient has received a neuromuscular blocking agent. Flaccidity, reduced tendon reflexes, and absent plantar responses may result from conditions varying as widely as acute encephalopathy; acute myelopathy with spinal shock; polyneuropathy; defects in neuromuscular transmission; and, occasionally, a myopathy.
In association with drug overdose, sustained pressure on various parts of the body by hard objects may induce local necrosis of skin, subcutaneous tissue, and occasionally the underlying muscle, and may also be associated with severe compressive focal neuropathy. The examiner should look for characteristic erythematous blisters of the skin at the site of compression and suspect injury to an underlying nerve if the latter lies nearby. Electrophysiologic techniques help to identify these mononeuropathies.
Rapid, shallow respirations and a rising PaCO 2 are supportive of neuromuscular respiratory insufficiency and should prompt electrophysiologic studies, including electromyography (EMG) of the diaphragm, which can localize and classify the problem and gauge its severity.
Electrophysiologic techniques
For many disorders, electrophysiologic tests enhance the clinical assessment in a sensitive and quantifiable manner. This extension of the clinical examination is particularly valuable when the aforementioned confounding factors compromise assessment. Electrophysiologic testing is a component in the care of the patient. Clinical interpretation is incorporated with other information to formulate a diagnostic and prognostic evaluation or to monitor the effects of treatment.
The choice of electrophysiologic test to be performed in the ICU depends on the nature of the clinical problem. For example, sedation monitoring requires an EEG of four or fewer channels. For seizures, long-term monitoring for at least 48 hours is optimal. Quantitative CEEG monitoring can be useful in detecting ischemia at a reversible stage when due to vasospasm from subarachnoid hemorrhage. The EEG can help to narrow the differential diagnoses, for example, by differentiating seizures from metabolic encephalopathies. “Trending” or recording serial EEGs or evoked responses may clarify the prognosis in certain circumstances. Thus, although a standard EEG may have a role in assessing comatose victims of cardiac arrest, there is more prognostic promise in trending the automated or raw EEG over 48 hours or more to determine whether there is variability (invariant EEGs carry a worse prognosis), improvement, or worsening. It is also important to consider which patients should be monitored. Some groups—such as those with recent convulsive seizures and with acute structural brain lesions—are at especially high risk for seizures.
In patients with suspected neuromuscular diseases, the clinical picture guides the electrophysiologic approach and—when results are equivocal, as in some cases of critical illness neuromyopathy—percutaneous muscle biopsy may be important in supplementing the electrophysiologic tests.
Specific Tests
The following electrophysiologic tests have proven or potential usefulness in the study of nervous system disorders of patients in the ICU.
Standard EEG, CEEG, and quantitative EEG (QEEG)
Standard EEG consists of 20- to 30-minute single or serial recordings; CEEG and QEEG are discussed in Chapters 6 and 8 respectively. Digital EEG has paved the way for bedside monitoring, quantification of EEG signals, automated detection strategies, and the instantaneous transmission of EEG data from the bedside to the office of the electroencephalographer. The authors have developed an EEG classification system ( Table 34-1 ) for comatose patients that is relatively unambiguous, is easy to apply, and shows excellent interobserver reliability. Polysomnography has been underexplored in ICU patients, but holds promise in the approach to delirium and in assisting in prognostic determination after stroke, trauma, and other conditions. ,
Category | Subcategory |
---|---|
I. Delta/theta > 50% of the record (not theta coma) | A. Reactivity |
B. No reactivity | |
II. Triphasic waves | |
III. Burst-suppression | A. With epileptiform activity |
B. Without epileptiform activity | |
IV. Alpha/theta/spindle coma (unreactive) | |
V. Epileptiform activity (not in burst-suppression pattern) | A. Generalized |
B. Focal or multifocal | |
VI. Suppression | A. <20 μV but >10 μV |
B. ≤10 μV | |
GUIDELINES | |
1. Burst-suppression pattern should have generalized flattening at standard sensitivity for ≥1 second at least every 20 seconds | |
2. Suppression: for this category, voltage criteria should be met for the entire record; there should be no reactivity | |
3. When more than one category applies, the worst (most advanced) is selected |
EMG and Nerve Conduction Tests
All the tests routinely performed in the EMG laboratory can be done in the ICU ( Table 34-2 ). The choice of tests and sequence of testing will vary according to the circumstances. In the limbs, standard motor and sensory nerve conduction studies, needle EMG, and repetitive nerve stimulation are of great value. Phrenic nerve conduction studies and needle EMG of the diaphragm are performed if there is any possibility of a nervous system cause for respiratory insufficiency. The other respiratory electrophysiologic tests probably will prove valuable in the future. Preliminary studies indicate that stimulated single-fiber EMG and direct muscle stimulation may also be useful. Motor unit number estimates and muscle force and sound have yet to be tried, but are of great theoretical interest.
Conditions | Site of Dysfunction | Electrophysiologic Test |
---|---|---|
Neurologic respiratory insufficiency | Brain, spinal cord, peripheral nerves, neuromuscular junction, or diaphragm | Transcranial and cervical magnetic stimulation Phrenic nerve somatosensory evoked potentials Phrenic nerve conduction Repetitive phrenic nerve stimulation Needle EMG of the diaphragm Automated interference pattern analysis of the diaphragm Power spectral analysis of the diaphragm |
Polyneuropathies | Peripheral nerves | Motor and sensory nerve conduction, needle EMG |
Neuromuscular transmission defects | Neuromuscular junction | Repetitive nerve stimulation Stimulated single-fiber EMG |
Myopathies | Skeletal muscle | Motor and sensory nerve conduction, needle EMG Direct muscle stimulation Measurement of duration of compound muscle action potential |
Other tests | Measurement of muscle force and sound |
Evoked Potentials
SEPs are especially useful, inasmuch as they assess somatosensory pathways from the entry point of the sensory nerve to the cerebral cortex. Brainstem auditory evoked potential and blink reflex testing assess intrinsic brainstem circuitry and the integrity of extra-axial components of the relevant cranial nerves (see Chapters 19 , 24 and 25 ). Preliminary work has shown that later potentials (event-related or cognitive-evoked responses, discussed in Chapter 29 ) hold promise for detecting cognitive processing in patients who are not capable of showing behavioral responses. Such patients may have favorable outcomes, but this awaits further study.
Transcranial and Transcervical Magnetic Stimulation
Motor evoked potentials are discussed in Chapter 28 . Such techniques may help in assessing cerebral motor control, spinal cord dysfunction, and respiratory function, but their role in the ICU is still uncertain.
Technical and Patient-Related Considerations
When performing needle EMGs, it should be kept in mind that underlying nerve, muscle, and bone may be near the skin surface. The needle electrode should be advanced through the skin very carefully while constantly monitoring for the presence of insertion activity to ensure that the electrode is in muscle. This caution is particularly relevant to needle EMG of the diaphragm in young children, in whom the underlying muscle, including the diaphragm itself, is quite near the skin surface.
The relief of discomfort in conscious patients is worthy of comment. (Unconscious patients usually do not require analgesia or sedation for diagnostic tests.) Patients with Guillain–Barré syndrome find nerve conduction studies and EMG testing especially uncomfortable. Appropriate analgesia is therefore necessary for EMG procedures. Sedative drugs (especially midazolam) have profound effects on EEG recordings and should be used only when absolutely necessary.
Electrical interference is common in the ICU, and 60-Hz notch filters are therefore useful for both EMG and EEG machines. Adjacent machines or poorly grounded plugs may cause interference. The EMG machine must have adequately shielded cables. If other electrical devices are causing interference, these devices should be unplugged if safe to do so without harming the patient. Even then, 60-Hz artifact may be difficult to eliminate entirely.
Artifact from intravenous accurate control (IVAC) machines, movement artifact with ventilation, perspiration artifact, and current induction from proximity to other cables are common; static charge from drip chambers, fluid moving in ventilator tubing, and persons moving near or touching, moving, or percussing the patient are additional, commonly encountered sources of environmental and biologic artifact. With long-term EEG monitoring, problems with electrode impedance, especially ground and reference electrodes, may render the recording unreliable. The collodion technique of securing EEG electrodes is recommended. Wrapping the head in a bandage and taping the electrode wires together helps to preserve contacts and prevents traction and excessive movement artifact. Subdermal wire electrodes allow for artifact-free recording for days. The application of noncephalic leads or simultaneous video recording may help in detecting movement artifact. In applying EMG electrodes, it may be necessary, particularly in young children, to splint the limb to avoid excessive movement that may loosen the contact.
Electrical safety in the ICU is of special concern. Two-wire ungrounded devices that are not essential to patient survival should be unplugged with the agreement of the attending staff. If needed, these instruments must be grounded properly and plugged into an isolation transformer. The EEG or EMG machine must be plugged into a three-wire grounded outlet located in the same circuit branch that is used to power the other instruments connected to the patient. Extension cords must not be used. The reference or common line from the patient must be isolated electrically from the power line ground or include an appropriate type of current-limiting device. No additional ground electrode should be used. Switching the machine on and calibrating the EEG machine before the patient is connected, and disconnecting the patient before switching off the instrument, are recommended. Electrical interference should be investigated promptly because this may indicate current leakage through the patient.
When indwelling catheters or pacemaker electrodes are already connected to the patient, special precautions must be taken to ensure that these connections are isolated properly or current-limited. Application of an electrical stimulus near an intravascular line that terminates close to the heart is of theoretical concern in regard to the induction of arrhythmias or asystole. However, one of the authors (CFB) often stimulates at these sites in adults and children and has never had such a complication. This has been borne out in the study by Schoeck and colleagues. Such stimuli should, however, be avoided in patients with temporary transvenous pacemakers.
Central nervous system disorders
The role of the EEG in the evaluations of different neurologic disorders is considered in detail in Chapters 3 and 4 . Attention here is confined to disorders encountered in the ICU.
Seizures
Overt convulsive seizures, either generalized or focal, can be seen and managed clinically. However, the EEG allows the physician to look for nonconvulsive seizures, which may be clinically inapparent ( Fig. 34-1 ). Some evidence indicates that varieties of nonconvulsive status epilepticus other than absence status epilepticus can damage the brain, but this is controversial. , Prompt treatment of such patients should improve outcome and will shorten ICU and hospital stays. Continuous EEG monitoring is the optimal method of gauging the effects of antiepileptic drug therapy for status epilepticus. Problems of undertreatment or overtreatment can be minimized.

Impairment of consciousness may be attributed mistakenly to the cumulative effects of sedative drugs, intercurrent systemic (especially inflammatory) illness, or organ failure when, in fact, it is caused by nonconvulsive status epilepticus. The occurrence of nonconvulsive status is suggested by several clinical circumstances , :
- 1.
An apparently prolonged “postictal state” following generalized convulsive seizures or a prolonged reduction of alertness after an operative procedure or neurologic insult
- 2.
Acute onset of impaired consciousness or a fluctuating disturbance of consciousness with episodes of normal mentation
- 3.
Impaired mentation or consciousness with myoclonus of the facial muscles or nystagmoid eye movements
- 4.
Episodic blank staring, aphasia, automatisms (e.g., lip smacking, fumbling with fingers), or perseverative activity
- 5.
Aphasia without an acute structural lesion
- 6.
Other acute behavioral disturbances or impaired consciousness without obvious or adequate cause.
In such circumstances, an EEG should be obtained. If seizures or abundant epileptiform activity, such as periodic lateralized epileptiform discharges (PLEDs) or generalized epileptiform activity, is seen, more intensive monitoring with CEEG or serial recordings is indicated. ,
Continuous EEG is valuable in the management of status epilepticus, especially for the diagnosis of nonconvulsive seizures and for monitoring the success of antiepileptic drug regimens. In refractory status epilepticus, operationally defined as status epilepticus that continues for 60 to 90 minutes or more from the onset of therapy, full anesthesia usually is required to arrest the seizures. Agents include intravenous barbiturate anesthetic agents (e.g., thiopental and pentobarbital), inhalational agents (e.g., isoflurane), and intravenous nonbarbiturate anesthetics (e.g., midazolam, propofol, and etomidate). These drugs usually produce a burst-suppression pattern on the EEG to achieve control of seizures. In the authors’ experience, generalized suppression for 2 to 30 seconds is effective. Greater periods of suppression, at least with barbiturate anesthetics, are associated with profound depression of the vasomotor center and hypotension. Monitoring allows optimal administration of the necessary agent and therefore avoidance of underdosing as well as excessive administration, which can be followed by complications or protracted recovery.
Continuous EEG can be helpful in estimating the prognosis in various conditions: normalization of the EEG is associated with a good outcome, whereas burst-suppression (provided that it is not caused by drugs), recurring ictal discharges, and (to a lesser extent) PLEDs are less favorable. The cause of the seizures undoubtedly contributes to the prognosis. The duration of status epilepticus is also an independent determinant of mortality, thus emphasizing the importance of detection and prompt control of such seizures in the ICU.
The most common cause of myoclonic status epilepticus in coma is the postanoxic state after cardiac arrest. The EEG findings are variable. One of the most common patterns is generalized burst-suppression, often with epileptiform potentials occurring within the bursts. Myoclonus often accompanies the EEG bursts, but the temporal relationship may be inconsistent. Other patterns include periodic complexes against a suppressed background; again, the relationship of the myoclonus to the complexes is variable. Some EEGs show no cortical spiking or complexes; in these situations the myoclonus probably arises entirely from a subcortical structure such as the brainstem reticular formation. Thus, EEG monitoring allows insight into the site of seizure origin in the brain and is of great assistance in classification. The syndromic classification and clinical background are of great importance in guiding further management.
Trauma
Trauma may affect the brain by a number of mechanisms such as intraparenchymal or extraparenchymal hemorrhage, ischemia, axonal shear injury, and a variety of metabolic derangements. These conditions may occur in any combination, and a rich variety of EEG abnormalities thus may be encountered. Their prognostic significance rests not only on their severity but also on the reversibility of the responsible pathogenetic mechanisms.
The following EEG patterns have been described: slowing in the theta range; delta activity, including continuous focal arrhythmic, diffuse rhythmic or arrhythmic, or intermittent rhythmic patterns; faster frequencies; epileptiform activity, including focal spikes or seizures, either clinical or electrographic; synchronous periodic slow-wave bursts; triphasic waves; focal suppression; other asymmetries; and alpha, theta, or spindle coma patterns.
The timing of the EEG in head injury is important with respect to the types of abnormalities and their associated etiologic, prognostic, and management implications.
Localized abnormalities at any stage should raise the possibility of structural lesions. Changes in amplitude, especially localized attenuation of activity, should be considered to reflect an intracranial hematoma until proved otherwise, provided that local scalp swelling is excluded. Focal slowing sometimes is associated with such lesions but can occur as a transient phenomenon. Electrographic seizures usually reflect severe brain injury, are of reliable lateralizing value, and should prompt urgent brain imaging and treatment.
When the recording shows continuous rhythmic activity (including sleep phenomena), the presence of variability in frequencies and amplitude is more favorable for outcome than are recordings showing no such variability. Any reactivity is associated with a better prognosis than is no reactivity.
Sometimes trauma affects the brain indirectly. Fat embolism to the brain and lungs is a complication of fractures of long bones. The patient may be asymptomatic for 12 to 36 hours after the injury and then become obtunded in association with pulmonary symptoms and tachycardia. The EEG shows replacement of normal rhythms with diffuse polymorphic delta and theta activity accentuated over the frontal regions. Trauma to the neck may cause delayed occlusion of the carotid or vertebral arteries and clinical and EEG features of strokes in these territories.
Serial EEGs or continuous monitoring may be useful in determining prognosis and in detecting potentially treatable conditions such as seizure activity, brain herniation, hydrocephalus, and intracerebral or subdural hematomas. The latter should be suspected if the EEG shows increasing slow-wave activity, focal suppression, or chronically persisting focal abnormalities along with intermittent rhythmic delta activity. These EEG findings and their timing need to be considered along with the integrity of brainstem function and clinical responsiveness when formulating a prognosis for a particular patient.
With regard to traumatic brain injury, the conduction time of the median-derived SEP through the brain (central conduction time) determined at a single time-point in the first few days after injury does not predict the outcome accurately in individual cases. However, bilaterally absent, cortically generated N20 waveforms in the early stages after a head injury nearly always predict death or a persistent vegetative state, whereas normal cortical SEPs are associated with recovery of conscious awareness and lack of severe motor deficits in adult patients. Intermediate grades of SEP abnormality, in which the N20 responses are present but delayed or reduced in amplitude, have less precise or useful predictive value.
Anoxic-Ischemic Encephalopathy
Anoxic-ischemic encephalopathy shares many features with metabolic encephalopathies but also has distinct clinical and electrophysiologic features. Unlike most other metabolic disorders, it is often associated with neuronal death.
Anoxic-ischemic encephalopathy because of cardiac arrest is the third leading cause of coma in ICU patients, following trauma and drug overdose. Among comatose survivors of cardiopulmonary resuscitation, only about 1 in 4 survives to discharge; only 1 in 5 is able to return home; and fewer than 1 in 10 returns to former status, employability, and independence. It is desirable to have reliable, early predictors of both favorable and unfavorable outcomes for resource allocation, management, and counseling of family members. Clinical, EEG, SEP, and biochemical studies have correlated outcomes in patients after cardiac arrest, but few have examined combined variables prospectively in the same patients while ensuring that patients survived for at least several days, to prevent premature “self-fulfilling prophecies.” SEP results serve as a reliable predictor of poor outcome; bilateral abolition of N20 indicates with close to 100 percent specificity that the patient will not recover. However, preservation of the N20 response does not guarantee awakening; more than 40 percent of such patients do not recover conscious awareness. Long-latency SEPs might reliably provide early identification of patients with favorable outcome because they provide a better evaluation of cortical integrity than short-latency responses. In a preliminary study, patients whose N70 response was preserved consistently recovered awareness, with about half making a good recovery and the other half remaining disabled but still interactive and aware. Further studies on the role of long-latency responses, including cognitive event-related potentials, clearly are needed.
EEG can play a useful role in prognosis, especially when the N20 response of the median-derived SEP is preserved. Patients with diffuse but variable and reactive theta and delta patterns (not theta-pattern coma, in which there is little or no variability or reactivity) recover awareness. Similarly, those with more “malignant” EEG patterns, especially with generalized suppression of less than 20 μV or a burst-suppression pattern containing generalized epileptiform activity, do not recover awareness and either die in coma or remain in a vegetative state.
Metabolic Disorders
Anesthesia and metabolic encephalopathies share a number of features: EEG patterns show similarities, the graded EEG response to increasing anesthetic administration is similar to the evolutionary changes seen with increasing severity of metabolic or toxic derangement, and with some exceptions the changes are reversible.
In such a model the anesthetic dose, like the severity of metabolic encephalopathy, shows an inverse relationship with EEG frequency and a direct relationship with amplitude. Such slowing accompanies decreased unitary activity of cerebral cortical neurons. This linearity breaks down at high doses, with the appearance of a burst-suppression pattern or epileptiform activity. The following sequential EEG changes are common to increasing levels of anesthesia and to most metabolic encephalopathies of increasing severity:
- 1.
Desynchronization of background activity, followed by the appearance of increasing amounts of theta activity
- 2.
Increase in the rhythmicity and voltage of delta activity, initially mixed with faster frequencies
- 3.
Simplified delta activity (without faster frequencies)
- 4.
Burst-suppression pattern, with the duration of suppression becoming longer with deeper levels
- 5.
Suppression leading to an isoelectric EEG.
This progression applies to sedative drug intoxication and to most metabolic encephalopathies, including hepatic and renal failure, septic encephalopathy, carbon dioxide narcosis, and adrenal failure. This analogy has limitations, however, and is not perfect. First, with certain clinical conditions capable of causing neuronal death (e.g., anoxic-ischemic encephalopathy), the EEG changes are not reversible and may progress, if severe enough, to permanent suppression. Second, in some diseases, specific features are superimposed on the patterns of sinusoidal waves or suppression, such as triphasic waves in hepatic encephalopathy, renal failure, and septic encephalopathy, and spikes in anoxia and penicillin-induced encephalopathy. Third, the number of metabolic encephalopathies that progress to a burst-suppression or suppression pattern is limited. Examples of the latter include anoxic-ischemic encephalopathy, septic encephalopathy, drug-induced coma (especially from barbiturates and benzodiazepines), hypothermia, and extreme hypoglycemic encephalopathy. Most others do not progress to this degree of abnormality unless the encephalopathy is associated with raised intracranial pressure and resultant global ischemia, as in the cerebral edema accompanying acute hepatic encephalopathy or Reye syndrome.
Age has a bearing on EEG phenomena in coma. Triphasic waves are not found in children, whereas 14- and 6-Hz positive spikes have been described in comatose children with Reye syndrome or hepatic encephalopathy.
Table 34-3 lists a number of metabolic conditions, along with special caveats that depart from the anesthetic model, and the degree of reversibility of a suppression or burst-suppression pattern. Examples include drug intoxication, hepatic failure, Reye syndrome, uremia, dialysis encephalopathy, septic encephalopathy, porphyria, hypothyroidism, Wernicke encephalopathy, Addison disease, hypercalcemia, hypocalcemia, hypoglycemia, and hypothermia.
Etiology | Additional Features | Reversibility and Implication of Burst-Suppression or Suppression |
---|---|---|
Anoxia | Epileptiform activity Alpha/theta coma * Generalized periodicity | Usually not reversible Usually unfavorable Usually unfavorable ‡ |
Drug intoxication | Some may produce epileptiform activity; a mixture of beta plus theta/delta suggests barbiturate or benzodiazepine overdose | Completely reversible for all patterns |
Hepatic failure | Triphasic waves † | Not reversible (older literature) ? Potentially reversible if liver function improves or with detoxification |
Reye syndrome | 14- and 6-Hz positive spikes in 50% (controversial significance) | Probably not reversible (dependent on damage from brain swelling and/or hypoglycemia) |
Uremic encephalopathy | Triphasic waves † or epileptiform activity in some; photic sensitivity | Probably reversible |
Septic encephalopathy | Triphasic waves † in some | Potentially reversible if the patient survives multiple organ failure |
Dialysis encephalopathy | Epileptiform activity (generalized spike-wave abnormality) | Not reversible in advanced cases |
Porphyria | Focal features (e.g., spikes, suppression) occasionally seen; usually transient | Largely reversible; EEG may be slow to improve |
Hypothyroidism | Low amplitude | Should resolve, except in cretinism |
Wernicke encephalopathy | May show sharp and slow-wave complexes, periods of suppression in advanced cases | EEG may improve; many patients have severe amnesia and ataxia |
Addison disease | Majority are normal; others show diffuse slowing | Resolves, especially with corticosteroids |
Hypercalcemia | May have triphasic waves or FIRDA | Rare, resolves |
Hypocalcemia | Bursts of generalized spikes | Interictal suppression reversible |
Hypoglycemia | May show epileptiform activity, periodic complexes | Partly reversible |
Hypothermia | No change until <30°C Burst-suppression at 20°C to 22°C Suppression at <18°C | Completely reversible Completely reversible Completely reversible |
* Alpha or theta coma patterns have been described in anoxic-ischemic encephalopathy, brainstem lesions, and drug intoxication, especially with barbiturates. In intoxication, the prognosis is favorable. In anoxic-ischemic encephalopathy or brainstem lesions, these patterns usually but not invariably are associated with a poor prognosis for recovery of full conscious awareness. The alpha-theta coma pattern is unstable and usually changes to a more definitive prognostic pattern in 5 to 6 days from coma onset.
† Triphasic waves were thought originally to be specific for hepatic encephalopathy but can occur in a variety of metabolic encephalopathies, septic encephalopathies, and neurodegenerative conditions. In the context of an acute alteration in alertness, however, they are highly suggestive of metabolic encephalopathy. Hepatic or renal failure and septic encephalopathy are the most common underlying causes in this situation.
‡ A burst-suppression pattern after cardiac arrest is associated with 96 percent mortality rate; recordings should be done more than 24 hours after arrest and repeated to help establish the prognosis.
Infections
Bacterial and tuberculous meningitis usually produce irregular, widespread delta activity as the predominant EEG abnormality. Background activity usually is preserved better than in encephalitis. The improvement in frequencies parallels clinical resolution. In patients with a good prognosis, the EEG shows rapid normalization within 6 to 9 days. Prolongation of EEG abnormalities beyond 2 weeks suggests a complication such as infarction from vasculitis, hydrocephalus, or subdural hygromas (the latter in young children).
Encephalitis also usually produces delta activity as the predominant EEG abnormality. Multifocal epileptiform activity commonly occurs and is a reflection of cortical gray matter disease. In herpes simplex encephalitis, the temporal regions are the site of maximal slowing; between the 2nd and 14th days of illness these may contain PLEDs with a repetition rate of 0.5 to 2 per second. This feature is important in suggesting herpes simplex encephalitis, which is treatable with the antiviral agent acyclovir. Multifocal delta activity may accompany acute disseminated encephalomyelitis, a postinfectious, immunologically mediated syndrome characterized by perivenous demyelination.
Septic encephalopathy, in which the brain appears to be affected in an indirect, reversible fashion (at least in the early, acute stage), behaves like most metabolic encephalopathies in that it closely follows the “anesthetic model” ( Figs. 34-2 and 34-3 ). Cerebrospinal fluid analysis and imaging studies are usually unremarkable.


Structural Lesions
Single structural lesions in the supratentorial compartment produce coma mainly by compression of deeper structures such as the diencephalon or midbrain tegmentum. The EEG manifestations may be diffuse by the time the patient is in coma, but a supratentorial lesion should be suspected if significant and consistent asymmetry in voltage is noted between the two hemispheres or if intermittent rhythmic delta activity is exclusively frontal. Another mechanism by which supratentorial lesions produce coma is by causing seizures. Epileptiform activity on EEG may suggest that an earlier seizure was missed clinically and that the comatose patient is in a postictal state. Alternatively, electrographic seizure activity may indicate a nonconvulsive seizure or status epilepticus.
Lesions limited to the brainstem often do not produce much EEG slowing; however, sleep potentials sometimes are found. Often the EEG shows an alpha pattern that may be more marked posteriorly. Care should be taken to ensure that the patient is in coma and not in a locked-in state, as discussed in Chapter 3 .
Cerebrovascular Diseases
Cerebrovascular disorders typically cause focal structural brain lesions. Sometimes, however, the lesions are not single. In subarachnoid hemorrhage, for example, multifocal vasospasm may cause multifocal delta or epileptiform activity. Watershed ischemia also shows bilateral abnormalities but with maximal localization over the posterior temporal-occipital-parietal regions. The background is slow and often accompanied by periodic epileptiform activity. Thrombosis of the superior sagittal sinus and draining veins would be expected to produce a somewhat similar picture of bilateral arrhythmic delta and epileptiform activity, but this activity may not consistently be maximal posteriorly in the head.
Metabolic consequences of cerebrovascular diseases (e.g., inappropriate antidiuretic hormone secretion and hyponatremia) may alter the EEG diffusely, as may hydrocephalus with coma.
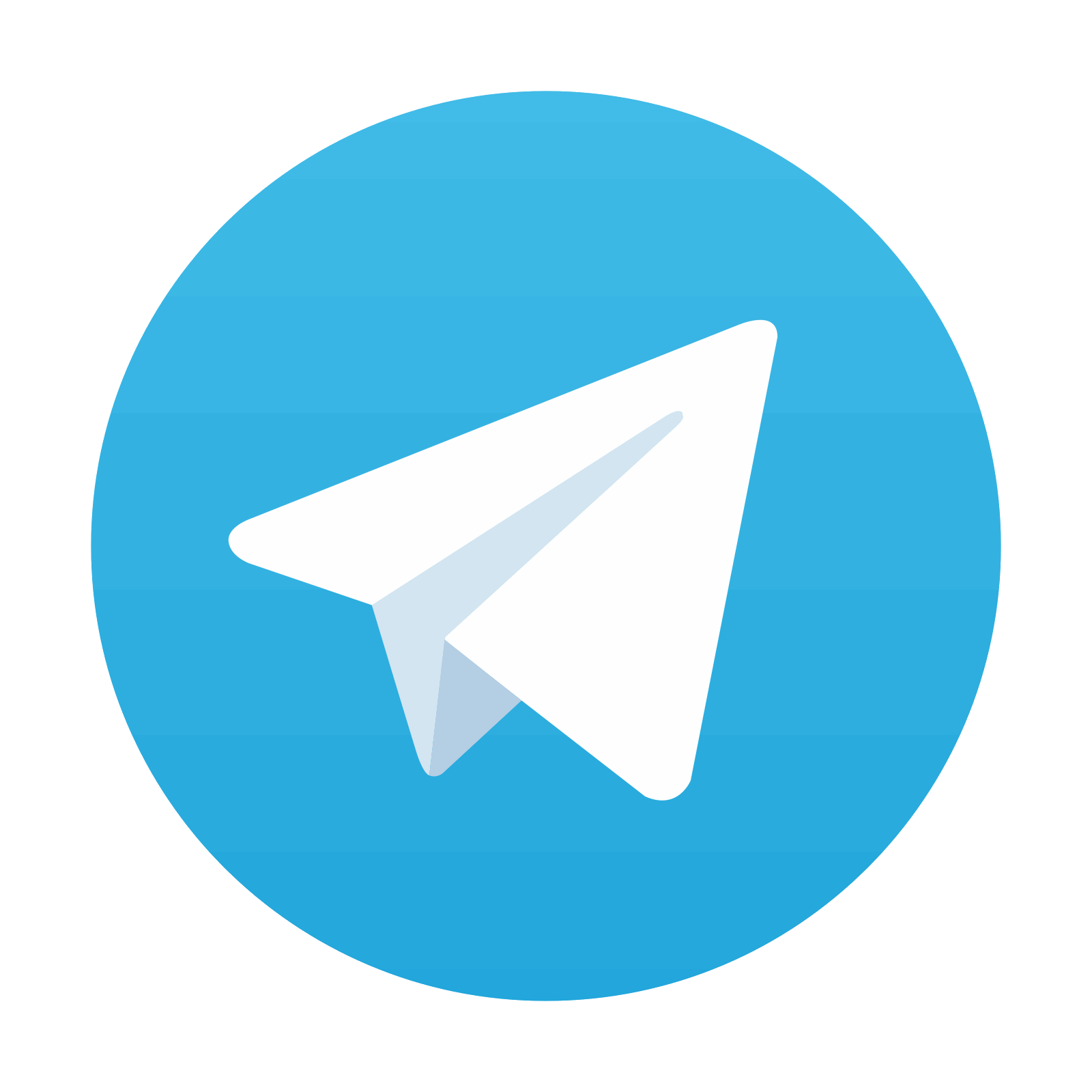
Stay updated, free articles. Join our Telegram channel

Full access? Get Clinical Tree
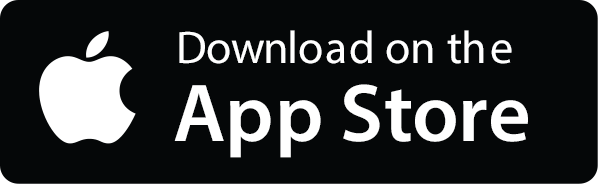
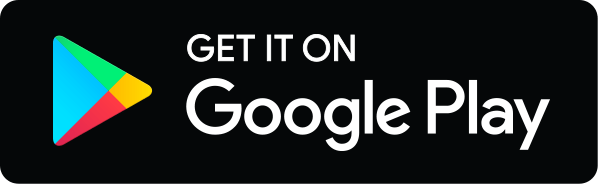