Electrodiagnostic methods are well established in the evaluation of the sacral nervous system, which has a critical role in the control of bladder, bowel, and sexual functions. The application of electrodiagnostic techniques in this context closely followed their introduction into clinical neurology, the first technique being needle electromyography (EMG). Electrodiagnostic methods have been proposed for both research and diagnostic applications in patients with suspected neurogenic sacral dysfunction. The emphasis of this chapter is on established tests that are of diagnostic value in the assessment of individual patients. These include EMG, bulbocavernosus reflex studies, and pudendal somatosensory evoked potentials (SEPs). Other tests that are not of clinical diagnostic value are also presented briefly. Detailed descriptions of these investigational sacral electrodiagnostic tests are provided elsewhere.
Established electrodiagnostic tests
Electromyography
Kinesiologic EMG
The aim of kinesiologic EMG is to assess the time course and intensity of activity of individual muscles during various maneuvers. Such recordings reveal the behavior of pelvic floor and sphincter muscles during bladder or rectal filling and emptying (cystometry and anal manometry studies, respectively). It usually is referred to simply as EMG, which causes confusion with needle EMG performed for the diagnosis of motor unit (MU) pathology.
There is no commonly accepted standardized technique. Bioelectrical activity typically is sampled from a single muscle detection site. Figure 31-1 shows the most useful sphincter and pelvic floor muscle insertion sites. Various types of surface or intramuscular (needle or wire) electrodes can record the kinesiologic EMG signal; the former have problems with artifacts and contamination from other muscles, whereas the latter have problems with representation of the large pelvic floor muscles. The quality of the EMG recorded from the external urethral sphincter (EUS) muscle is improved by a catheter-mounted surface electrode device that applies mild suction. Although either standard EMG equipment or EMG facilities contained within urodynamic systems can be used, the better visual and audio control provided by standard EMG equipment facilitates optimal electrode placement and improves recordings. Generally, the EMG signal is described only qualitatively, but quantitative techniques are also available and increase the validity of the findings.

The normal sphincter EMG shows continuous activity of “low-threshold” MUs, which persists even when subjects are asleep. Such activity can also be recorded in many, but not all, sites of the levator ani and the deeper external anal sphincter (EAS) muscle. This continuous EAS activity is reduced in a subgroup of patients with idiopathic anal incontinence. EMG activity may be increased voluntarily or reflexly (e.g., by talking, deep breathing, or coughing) when “high-threshold” MUs are recruited. Strong voluntary activation of sphincter and pelvic floor muscles can be sustained only for less than 1 minute. In health, all sphincter and pelvic floor EMG activity disappears on voiding and defecation. However, in central nervous system (CNS) disorders, detrusor contraction and straining may be associated with increased sphincter EMG activity (“detrusor–sphincter dyssynergia” and “paradoxical puborectalis contraction,” respectively). Neurogenic, uncoordinated sphincter behavior is sometimes difficult to differentiate from voluntary contractions that may occur in poorly compliant patients and in patients with dysfunctional voiding.
Little is known about the normal activity patterns of different pelvic floor (i.e., parts of the levator ani) and sphincter muscles (i.e., sphincter urethrae, urethrovaginal sphincter, and the EAS). The assumption that they all act as one muscle may be incorrect because no relation is found between the ability to activate pelvic floor and the sphincter urethrae muscles. Coordinated behavior is often lost in disease states. A consistent contraction sequence of the superficial and deep pelvic floor muscles is found in continent but not in incontinent women. Apart from polygraph urodynamic recordings to assess detrusor–sphincter coordination, the diagnostic usefulness of kinesiologic EMG has not been established. Needle electrodes are more useful than perineal patch electrodes to demonstrate MU quiescence during voiding. In selected patients with neurogenic detrusor overactivity, EMG of the EUS muscle can also be used to demonstrate the onset of detrusor contractions.
Needle EMG
Although abnormalities on needle EMG may be caused both by muscle disease and by changes in muscle innervation, sacral myopathies are of no clinical importance. Thus, the aim of needle EMG of the sacral myotomes is to differentiate abnormally from normally innervated striated muscle.
For needle EMG, an advanced EMG system with quantitative template-based analysis of motor unit potentials (MUPs) is ideal. The commonly used amplifier filter setting for needle EMG is 5 Hz to 10 kHz; this needs to be identical to that set when reference values for MUP parameters were compiled.
Because of easy access, bulk of the muscle, and the relative ease of its examination, the EAS is the most practical muscle for needle EMG of the lower sacral myotomes. Examination of the subcutaneous EAS muscle is usually sufficient for most diagnostic purposes. To reach this muscle, the needle is inserted about 1 cm from the anal orifice to a depth of 3 to 6 mm (see Fig. 31-1 ). The left and right EAS muscles should be examined separately by systematic needle insertions into at least four muscle sites.
As with evaluation of other skeletal muscles, needle EMG of the sphincter and pelvic floor muscles is divided into observation of insertion activity, evaluation of any spontaneous activity, (quantitative) assessment of MUPs, and (qualitative) assessment of the interference pattern. In addition, counting of the number of continuously firing MUs during relaxation and observation of MU recruitment on reflex and voluntary activation are recommended.
Insertion Activity and Spontaneous Activity
As in other skeletal muscles, needle movement in sphincter muscles elicits a short burst of insertion activity (gain: 50 μV/division; sweep speed: 10 msec/division). Approximately 10 to 20 days after an axonal lesion, insertion activity becomes more prominent and prolonged, and abnormal spontaneous activity (i.e., fibrillation potentials and positive sharp waves) appears ( Fig. 31-2 ). The absence of insertion activity with appropriate placement of the needle electrode usually signifies an end-point state after complete muscle denervation.

In partially denervated muscle, some MUPs remain and mingle with spontaneous denervation activity. Because the MUPs in sphincter muscles are short and mostly biphasic or triphasic (as are fibrillation potentials), it takes considerable experience to differentiate one from the other. In this situation, examination of the bulbocavernosus muscle is particularly useful because, in contrast to sphincter muscles, it lacks ongoing activity of low-threshold MUs during relaxation (see Fig. 31-2 ). Needle insertion into the bulbocavernosus muscle is shown in Figure 31-1 .
In long-standing, partially denervated muscles, simple or complex repetitive discharges may also be provoked by needle movement or muscle contraction, or may occur spontaneously (e.g., rhythmically). This activity is sometimes found in the urethral sphincters of patients without any other evidence of neuromuscular disease and, in that situation, is regarded as a nonspecific sign without predictive value for the outcome of surgery. However, profuse repetitive discharges (called decelerating bursts and complex repetitive discharges), which have been described in the urethral sphincter muscles of some young women with voiding dysfunction ( Fig. 31-3 ), may be diagnostic of idiopathic urinary retention (Fowler syndrome). The spontaneous discharges are thought to cause involuntary muscle contraction and secondary detrusor dysfunction.

In partially denervated sphincter muscle, a loss of MUs occurs and can be quantified by MUP count during relaxation. The sensitivity of this approach for the diagnosis of neuropathic lesions in the EAS muscle is low; however, MU count during relaxation was reduced in most patients with idiopathic fecal incontinence. In limb muscles, qualitative assessment of the interference pattern (i.e., reduction in the number of activated MUs and increase in their firing rate) usually is performed for estimating MU loss. This is more difficult in the sphincter and pelvic floor muscles because concomitant assessment of the power of muscle contraction cannot be made.
MUP Analysis
With axonal reinnervation after complete denervation, short, low-amplitude, biphasic and triphasic nascent MUPs initially appear; these soon become polyphasic, serrated, and of prolonged duration. In small sphincter muscles with inefficient reinnervation after denervation, such a picture may persist, and on quantitative analysis, MUP area and duration may have values below the lower confidence limits (“pseudomyopathic” MUPs).
By contrast, changes caused by collateral reinnervation after partial axon-loss lesions are reflected in prolongation of the MUP waveform, which may have small, late components (“satellites”), as shown in Figure 31-4 . These have been defined as late spikes firing concomitantly with the main component of the MUP and starting at least 3 msec after the end of it. In newly formed axon sprouts and endplates, transmission is insecure, which may be reflected in MUPs as instability (i.e., jitter and blocking of individual components). Over time, in the absence of further denervation, the reinnervating axonal sprouts increase in diameter so that activation of all parts of the reinnervated MU becomes nearly synchronous; this increases the amplitude and reduces the duration of the MUPs (see Fig. 31-4 ). This phenomenon may be different in sphincter muscles affected by degenerative disorders such as multisystem atrophy, where long-duration MUPs seem to remain a prominent feature ( Fig. 31-5 ).


Three quantitative analysis techniques are available for the systematic examination of individual MUPs, all of which have a similar sensitivity for detecting neuropathic changes. Reference data for mean values and outliers for the EAS muscle have been published for each of these techniques. The first technique follows an algorithm similar to that used many years ago by Buchthal, but the MUPs are analyzed on the screen. This modified manual-MUP technique is time-consuming (2 to 3 minutes per muscle site); demanding on the operator (reproducible MUPs have to be identified, the one with the smoothest baseline selected, and the duration cursors set); and inevitably open to bias (especially in the determination of MUP duration). The second technique uses a trigger and delay unit, which is set on a steadily firing MUP during a constant level of EMG activity. The MUP is averaged until the baseline becomes smooth (usually about 1 minute). This single-MUP analysis is also time-consuming, provides fewer MUPs than the other two techniques, and is again prone to personal bias. In the template-operated techniques available only on advanced EMG systems (e.g., multi-MUP analysis), the operator indicates when the computer takes the previous (last) 5 to 10 seconds of the signal. MUPs are extracted automatically from that signal and sorted into several classes, each representing the average of consecutive discharges of MUPs (see Fig. 31-5 ). MUPs with an unsteady baseline (i.e., unclear beginning or end) or MUPs distorted by averaging need to be recognized and deleted. Multi-MUP analysis is the fastest and easiest to use of these three quantitative MUP analysis techniques.
In sphincter muscles, two MUP populations with different characteristics exist: (1) smaller, continuously firing, low-threshold MUPs; and (2) larger, recruited, high-threshold MUPs. To increase the accuracy of analysis it was proposed that MUPs should be sampled at an activity level permitting 3 to 5 MUPs to be evaluated by a template-operated multi-MUP technique. In contrast to the manual-MUP technique (biased toward low-threshold MUPs) and single MUP (biased toward high-amplitude MUPs), the multi-MUP technique samples all consistently firing MUPs during sphincter muscle relaxation and at slight activation.
In the small sphincter muscle, collecting ten different MUPs is held to be a minimal requirement for using single-MUP analysis. Using the manual-MUP and multi-MUP techniques, sampling of 20 MUPs (the standard number in limb muscles) from each EAS presents no problem in healthy controls and in most patients.
MUP Parameters
A number of MUP parameters are used in the quantitative EMG diagnosis of neuromuscular disease. Traditionally, amplitude and duration are measured and the number of phases is counted. In the EAS muscle, however, only the MUP area, duration, and number of turns are needed for optimal diagnostic power (sensitivity and specificity). Criteria for the diagnosis of neuropathic conditions have also been validated.
The MUP area reflects the sum of the total bioelectrical activity generated by the MU. It is the most sensitive MUP parameter for detecting neuropathic disorders in the EAS muscles.
The MUP duration is the interval between the first consistent deflection of the MUP waveform and the point when it finally returns to the baseline. The difficulty with duration measurement is in the exact definition of the beginning and end of the MUP. Using manual positioning of duration cursors depends on amplifier gain; at a higher gain, MUPs seem longer. Because of difficulty with automatic measurement, it has also been suggested that late (satellite) potentials should be excluded from the measurement. However, in the sphincter muscles of patients with suspected multisystem atrophy, the diagnostic power of MUP analysis depends critically on the inclusion of late potentials when MUP duration is measured.
The number-of-turns parameter is similar to the traditional MUP parameter of number of phases. A turn is defined as a change in direction of the MUP trace that is larger than a specified amount (e.g., 100 μV). The number of turns is an MUP parameter that is particularly sensitive to reinnervation changes in small muscles such as the EAS.
With computer analysis, other MUP parameters are available. Thus, by advanced EMG systems, MUP duration of the negative peak can be measured, and thickness (thickness = area/amplitude ) and size index (size index = 2 × log [amplitude] + area/amplitude ) can be calculated automatically. It has been suggested that the combination of MUP thickness and number of turns might be even more accurate than the previously suggested combination of MUP area, duration, and number of turns.
Interference Pattern Analysis
At high levels of activation of sphincter and pelvic floor muscle, a dense interference pattern can be seen and can be assessed quantitatively using a number of automatic quantitative techniques, the turn/amplitude analysis being the most popular. On applying turns/amplitude analysis in sacral electrodiagnosis, subjects contract muscles voluntarily or reflexly by coughing. The examiner selects 0.5-second time intervals of the crisp EMG signal to be analyzed using several interference pattern parameters measured automatically by the EMG system. Although interference pattern sampling using turns/amplitude analysis is even faster than MUP analysis using the multi-MUP technique, its sensitivity for detecting neuropathic EAS muscles is only about half that of MUP analysis techniques. By contrast, turns/amplitude, but not MUP analysis, may detect evidence of denervation and subsequent reinnervation in postpartum women with mild anal incontinence. Qualitative assessment of the interference pattern has been recommended in sphincter and pelvic floor muscles to assess MU loss.
Summary
Template-based multi-MUP analysis is sensitive (equal to traditional MUP analysis techniques), fast (5 to 10 minutes per muscle), easy to apply, and less prone to personal bias. In the EAS muscle, its use is supported by common normative data, which are unaffected by age, gender, number and characteristics of vaginal deliveries, or mild chronic constipation. All these factors make multi-MUP analysis the technique of choice for quantitative analysis of reinnervation of the EAS.
In practice, needle EMG examination can be extended in the same diagnostic session from the lumbar and upper sacral myotomes to the lower sacral myotomes, which commonly require study after a cauda equina lesion. A concentric needle electrode can also be employed at the same diagnostic session for recording evoked direct (M-wave) and reflex muscle responses (bulbocavernosus reflex).
Sacral Reflexes
Sacral reflexes are the electrophysiologically recordable responses of the pelvic floor and sphincter muscles to stimulation of sensory fibers in the uro-ano-genital region. Clinically, two reflexes commonly are elicited in the lower sacral segments: (1) the penilo- or clitoro-cavernosus (i.e., bulbocavernosus) reflex; and (2) the anal reflex. The bulbocavernosus reflex is elicited by squeezing the glans penis and observing or palpating the resulting pelvic floor contraction. The anal reflex is evoked by perianal pinprick and is detected by observation of the anal wink. In both, the afferent and efferent limbs of the reflex arcs are in the pudendal nerves and cauda equina, and integration occurs centrally in the spinal cord at levels S2 to S4 (conus medullaris).
Electrophysiologic measurement of the sacral reflexes is quantitative, more sensitive, and more reproducible than are clinical methods, which may also produce false-positive results. Changes in the threshold, amplitude, and latency of the bulbocavernosus reflex occur not only because of changes in conduction along peripheral and central neural pathways but also as a consequence of changes in transmission within the interneurons of the CNS. With a constant stimulus, they depend on the physiologic state of the pelvic viscera (e.g., bladder ) and are affected by pathologic conditions such as suprasacral spinal cord lesions. Thus, electrophysiologic testing of sacral reflexes reveals not only the integrity of the sacral spinal reflex arc but also the excitation level of motor neurons in the sacral spinal cord.
To elicit sacral reflexes, electrical, mechanical, or magnetic stimulation can be used. Whereas the latter two modalities have only been applied to the penis, clitoris, and the suprapubic area, electrical stimuli can be applied to various sites: to the dorsal penile or clitoral nerve; perianally (see Fig. 31-1 ); and, using a catheter-mounted ring electrode, to the bladder neck/proximal urethra. In clinical practice, electrical stimulation of the penis or clitoris is used most commonly ( Fig. 31-6 ).

For electrical stimulation, placement of surface electrodes on the dorsum of the penis/clitoris and application of 10 single, 0.2-msec supramaximal stimuli at time intervals of 2 seconds (0.5 Hz) has been recommended. In addition to single-pulse electrical stimulation, two identical electrical pulses separated by a 3-msec interval can be used (i.e., double-pulse electrical stimulation). Double-pulse electrical stimulation is more efficient in eliciting sacral reflexes. For mechanical stimulation, either a standard commercially available reflex hammer or a customized electromechanical hammer can be employed. Using a reflex hammer, the stimulus is applied to a wooden spatula placed on the glans penis or clitoris. Such stimulation is painless and is particularly suitable for testing children.
For reflex recordings, concentric needle or surface electrodes are placed into or over the bulbocavernosus or the EAS muscle. The use of filters (10 Hz to 10 kHz), a sweep speed of 10 msec/division, and a gain of 50 to 1000 μV/division are recommended. Traditionally, the onset latency of the sacral reflex has been the only measured parameter. Onset latency provides information on the fastest nerve fibers in the sacral reflex arc. Latency of mechanically elicited bulbocavernosus reflex is comparable to that elicited by single and double electrical pulses. However, using electrical stimulation, sensory threshold (lowest perceived stimulus intensity) and reflex threshold (minimum stimulus intensity eliciting sacral reflex) can also be measured. They evaluate lower sacral sensory pathways and excitation level of the sacral reflex pathway, respectively. Although, for men, confidence intervals for these two new parameters have been established, their clinical utility has not yet been determined.
The sacral reflex evoked by dorsal penile or clitoral nerve stimulation consists of two components. The first, with a mean latency of 29.9 ± 5.7 msec in one study in men, is stable, does not habituate, and is thought to be an oligosynaptic reflex. In adults, values of 40, 36, and 36 m/sec have been suggested as the upper limit of normal for the shortest latency obtained on eliciting a series of reflex responses using single, double, and mechanical stimulation, respectively. The second component, which is not always demonstrable as a discrete response, has a similar latency to the sacral reflexes evoked by stimulation perianally (anal reflex) or from the proximal urethra, and it is a polysynaptic reflex. In normal subjects it is usually the first component that has a lower threshold. However, in some patients the first component of the bulbocavernosus reflex cannot be obtained with single electrical stimuli but, on strong stimulation, the second component appears and can be misinterpreted as a delayed early response. The situation may be clarified by using double electrical or mechanical stimuli that facilitate the reflex and reveal the first component.
Two unilateral arcs for the bulbocavernosus reflex have been demonstrated. Thus, by recording of the bulbocavernosus reflex from the left and right bulbocavernosus or the EAS muscles, separate testing of both bulbocavernosus reflex arcs can be performed. This is important because in patients with unilateral disease (e.g., sacral plexopathy or pudendal neuropathy) or asymmetric lesions (e.g., cauda equina syndrome; see Fig. 31-6 ), a healthy bulbocavernosus reflex arc may obscure a pathologic one.
Sacral reflex responses on stimulation of the dorsal penile and clitoral nerve are of value in evaluating patients with cauda equina and other lower motor neuron lesions, although a response of normal latency does not exclude the possibility of a partial axonal lesion in its reflex arc. In men with chronic cauda equina lesions, the penilo-cavernosus reflex has a sensitivity of 81 to 83 percent (with different stimulation techniques), and higher specificity, predictive values, and likelihood ratios than clinical testing. The value of sacral reflex testing is less clear in women. An abnormally short reflex latency of the bulbocavernosus reflex suggests an abnormally low position of the conus medullaris.
Sacral reflex testing should be part of the diagnostic battery, of which needle EMG exploration of the sphincter and pelvic floor muscles is the most important part. Sensitivity of the combination of penilo-cavernosus reflex (using different stimulation techniques) and quantitative EMG was 94 to 96 percent in one study of men with chronic cauda equina or conus medullaris lesions. The results, however, should always be interpreted in the clinical context.
Pudendal Somatosensory Evoked Potentials
The pudendal SEP is recorded easily following electrical or mechanical stimulation of the dorsal penile or clitoral nerves (see Fig. 31-1 ). The first positive peak, at 41 ± 2.3 msec in one study (called P1 or P40), usually is defined clearly in healthy subjects after computer averaging of 100 responses on electrical stimulation that is 2 to 4 times stronger than the sensory threshold ( Fig. 31-7 ). The response is, as a rule, of highest amplitude (0.5 to 12 μV) at the central recording site (Cz′–Fz of the international 10–20 system of electrode placement ) and is highly reproducible. Later negative (at about 55 msec) and subsequent positive waves show intersubject variability and have little clinical relevance.

Recording of pudendal SEPs sometimes is indicated in patients with a normal bulbocavernosus reflex who complain of abnormal sensation in the lower sacral segments, pointing to the possibility of a central (i.e., spinal cord) lesion above the sacral segments. It has been shown in such patients, however, that the tibial SEPs are more often abnormal than is the pudendal SEP, as in multiple sclerosis. Only rarely is the pudendal SEP abnormal and the tibial SEPs normal, suggesting a localized conus medullaris lesion. Furthermore, in the investigation of urogenital symptoms, the pudendal SEP is reported to be of no greater value than a clinical examination focusing on signs of spinal cord disease in the lower limbs. Abnormal pudendal SEPs seem to predict a poor surgical outcome after resection of a tight filum terminale.
The method is valid and robust, but seems to be of minor clinical value. There may, however, be circumstances in which it is reassuring to be able to record a normal pudendal SEP (e.g., when a patient complains of loss of bladder, genital, or perianal sensation). Cerebral SEPs elicited by penile/clitoral stimulation have been reported as possibly valuable for intraoperative monitoring in patients in whom the cauda equina or conus medullaris is at risk during surgery.
Other electrodiagnostic tests
The Sacral Motor System
Single-Fiber EMG
The aim of single-fiber EMG (SF-EMG) testing is similar to that of needle EMG: to differentiate normal from abnormal striated muscle. SF-EMG is most suitable for recording “instability” of MUPs (specifically, the jitter or variability in time interval between consecutive firings of muscle fibers, as discussed in Chapter 17 . The jitter may be increased by recent reinnervation as well as by diseases affecting neuromuscular transmission. The SF-EMG parameter that reflects MU morphology is the fiber density, which is defined as the mean number of muscle fibers belonging to an individual MU per detection site (recorded from 20 intramuscular detection sites).
SF-EMG has been applied in research into sacral nervous system physiology and into pelvic organ dysfunction. The normal fiber density for the EAS is below 2.0, increases with age, and is affected by gender (greater in women). Quantified needle EMG provides the same information on reinnervation in muscle as fiber density on SF-EMG, but it also reveals the presence of spontaneous denervation activity. After severe partial denervation, areas of fibrosis in muscle are “silent” on EMG exploration, and the results of the examination are based only on the MUP activity in surviving muscle. Such muscle is easier to examine with conventional EMG, which records from a larger volume of tissue than a single-fiber electrode.
Surface EMG with Noninvasive Electrode Arrays
Recently, surface EMG recording using noninvasive electrode arrays and multichannel EMG amplifiers was introduced to the sphincter and pelvic floor muscles (e.g., the EAS and puborectalis). Using this approach, investigators can localize muscle innervation zones and asymmetry in muscle innervation, and analyze discharge patterns of MUPs and propagation velocities along the muscle fibers—some characteristics of MUPs. Although comparisons with normal have been made of the findings in pathologic conditions, the clinical value of these methods in diagnosing neurogenic sacral disorders has not been demonstrated.
Motor Nerve Conduction Studies
Recording of the compound muscle action potential (CMAP) elicited by electrical stimulation of a motor nerve is hindered with regard to the lower sacral segments by access to stimulation sites and by difficulty in recording a reproducible supramaximal response. As a consequence, the only electrophysiologic parameter that can be measured in the pelvic floor is the pudendal nerve terminal motor latency (PNTML), which measures conduction in the fastest-conducting motor nerve fibers.
PNTML can be elicited by bipolar perianal stimulation (see Fig. 31-1 ) and recorded with a concentric needle electrode from the bulbocavernosus, EAS, or urethral sphincter muscles. Using this perianal technique, the PNTML is approximately 5 msec. More often, a motor response is evoked by a bipolar stimulating electrode fixed onto a distal gloved index finger inserted into the rectum, and by the recording electrode pair placed 8 cm proximally on the base of the index finger (the “St. Mark’s electrode”). It is assumed that, using this approach, the pudendal nerve is stimulated close to the ischial spine, and that the response recorded is of the EAS muscle. In women, intravaginal stimulation and recording from the bulbocavernosus muscles has also been undertaken, with similar distal latencies. However, the latency of such a response is typically only around 2 msec, which seems unusually short compared with the perineal technique and with conduction in the much thicker motor fibers of peripheral nerves in the limbs. It seems unlikely that the PNTML using the St. Mark’s electrode really evaluates conduction along the last 8 cm of the pudendal nerve. Stimulation of the terminal pudendal branches or pelvic floor muscles near their motor points seems more likely, and this is supported by the much longer PNTML (3.7 ± 0.9 msec) obtained with a monopolar intrarectal stimulation electrode.
Prolongation of the PNTML as measured by the St. Mark’s electrode has been found in several sacral disorders and has also been claimed to predict the outcome of therapeutic interventions by some investigators but not by others. This prolongation was taken as a sign of damage to the pudendal nerves, leading to the term pudendal neuropathy, which is used particularly by proctologists. A prolongation of PNTML has been equated mistakenly with pelvic floor denervation, but studies have failed to demonstrate any correlation between a prolonged PNTML and morphologic (EAS thickness ) or functional signs of denervation. The clinical significance of such prolongations in latency is unclear in the absence of relevant morphologic studies. The authors have found the PNTML unhelpful for diagnostic purposes in individual patients with pelvic floor disorders. In patients with fecal incontinence, conventional needle EMG is more useful than the PNTML. Eliciting a CMAP in pelvic floor muscles using perianal stimulation may be helpful in excluding a complete peripheral (axonal) lesion.
Conduction Studies with Root Stimulation
The sacral roots can be stimulated electrically or magnetically at their exit from the lower spinal canal. Typical mean latencies vary from 5 to 9 msec, depending on the stimulation site. Lumbosacral stimulation often evokes a large stimulus artifact that can be decreased by positioning the ground electrode between the stimulating and recording electrodes. Selective stimulation of individual sacral roots is possible by appropriate positioning of surface stimulating electrodes. However, nonselective stimulation is applied more commonly and results in activation of all muscles innervated by lumbosacral segments; responses from the large gluteal muscles may contaminate surface recordings from the sphincter muscle, and needle detection is necessary.
Recording the motor evoked potential (MEP) with lumbosacral magnetic stimulation has been less successful than with electrical stimulation. It also often evokes a large stimulus artifact that can be decreased by positioning of the ground electrode between the recording electrodes and the stimulating coil. Demonstration of a perineal MEP on stimulation over the lumbosacral spine and recording with a concentric needle electrode occasionally may be helpful, but an absent response has to be evaluated with caution.
Assessment of Central Motor Pathways
Magnetic or electrical stimulation can be used to stimulate the brain, the response being recorded from the pelvic floor and sphincter muscles. The aim of these techniques is to assess conduction, not only in peripheral but also in central motor pathways. In contrast to magnetic stimulation, cerebral electrical stimulation is painful and nowadays is used only on anesthetized patients during intraoperative monitoring.
In response to magnetic stimulation over the motor cortex of healthy subjects, MEPs in a variety of sphincter and pelvic floor muscles have been reported. As with measurements from limb muscles, only latencies are used in evaluating the response. When no facilitatory maneuver is used, mean latencies are 19 to 21 msec, but slight voluntary contraction of the sphincter and pelvic floor muscles shortens latencies by up to 3 msec. By subtracting the latency of the MEPs obtained by stimulation over the scalp and at the L1 level, a central conduction time of 15 to 16 msec without and 13 to 14 msec with facilitation is obtained for pelvic floor and sphincter muscles.
Although a substantially prolonged central conduction time usually is obtained in patients with multiple sclerosis and myelopathy, the diagnostic contribution of the technique is minor because these patients also have clinically evident spinal cord disease. However, MEPs may be useful in patients with unclear localization of spinal lesions. In patients with peripheral (e.g., cauda equina) lesions, a concomitant spinal cord lesion may be obscured clinically but revealed neurophysiologically by prolongation of the central motor conduction time calculated using both lumbar magnetic stimulation and the F-wave latency measurement; patients without an additional spinal cord lesion only have central prolongation obtained by lumbar magnetic stimulation. A well-formed sphincter MEP with a normal latency occasionally may be helpful in a patient with a possibly nonorganic disorder or in a medicolegal case.
The Sacral Sensory System
Electroneurography of Dorsal Penile Nerves
In healthy men, a low-amplitude (10-μV) sensory nerve action potential (SNAP) is recorded by placing a pair of stimulating electrodes across the penile glans and placing a pair of recording electrodes across the base of the penis. The sensory conduction velocity of the dorsal penile nerve is 27 to 33 m/sec. Measuring the conduction distance is difficult because penis length is readily changeable. The antidromic method of stimulating the pudendal nerve transrectally by the St. Mark’s electrode and recording SNAPs from the penis seems more reproducible. Nerve conduction studies of the dorsal penile nerve have been proposed as a means of assessing sensory function in the lower sacral segments. Theoretically, a normal-amplitude SNAP of the dorsal penile nerves in an insensitive penis distinguishes a sensory lesion proximal to the dorsal spinal ganglion (e.g., cauda equina, central pathways) from a lesion distal to the ganglion (e.g., sacral plexus, pudendal nerves). The test is not often used.
Electrical Responses of Dorsal Sensory Roots
Using surface electrodes at the level of the lower thoracic and upper lumbar vertebrae, particularly in slim men, a low-amplitude (less than 1 μV) monophasic negative potential with a mean peak latency of about 12.5 msec can be recorded on stimulation of the dorsal penile nerves. With epidural electrodes, sacral root potentials have been recorded in 59 percent, and postsynaptic cord potentials in 41 percent, of men.
Intraoperatively, with the sacral roots exposed, SNAPs can be recorded more readily on stimulation of the dorsal penile and clitoral nerves. This has been helpful in preserving roots important for perineal sensation in spastic children undergoing dorsal rhizotomy, perhaps thereby decreasing the incidence of postoperative voiding dysfunction. No use for such recordings has been established outside of the operating room.
Cerebral SEPs on Stimulation of Pelvic Viscera
Cerebral SEPs on stimulation of pelvic viscera are claimed to be more relevant to neurogenic sacral dysfunction than the pudendal SEP because the A-delta sensory afferents from pelvic viscera accompany the autonomic fibers in the pelvic nerves and also participate in visceral reflexes. To obviate depolarization of somatic afferents for such measurements, bipolar electrical stimulation applied by catheters and other special devices is required. These cerebral SEPs have been shown to have a maximum amplitude over the midline (Cz′–Fz). They are of low amplitude (1 μV or less) and of variable configuration, which makes their identification difficult, even in control subjects. On stimulation of the bladder, urethra, and sigmoid colon, the typical latency of the most prominent negative potential (N1) is about 100 msec, whereas, with stimulation of the anal canal, the response has a slightly longer latency. On electrical and mechanical stimulation of the rectum, the cerebral SEP is similar in shape and latency to the pudendal SEP. No clinical utility of such recordings has been established. Instead of recording the cortical response, the sympathetic skin response (SSR) can be recorded from the hand after bladder/urethral stimulation. A preserved palmar SSR then demonstrates continuity of the sensory pathways from the bladder neck/posterior urethra to the cervical spinal cord level.
Assessment of Sacral Sensation
Although not strictly a neurophysiologic test, measurement of electrical thresholds adds clinically unobtainable information on sensory function of the lower urinary tract. Electrical currents are applied to the bladder, urethra, or genital skin using catheter-mounted or surface electrodes. High-frequency stimulation (greater than 20 Hz), with a stimulus duration of 0.5 or 1 msec, is used because it is perceived more easily in the lower urinary tract. Measurement of sensory thresholds with such stimulation is reproducible, and normative data have been published. To date it has been used in only a few conditions (e.g., painful bladder syndrome ). In addition, palmar SSR and perineal surface EMG recordings can be used for more objectively demonstrating sensations during cystometry. The activity of both appears and increases in parallel with the first sensation of bladder filling, and with the first desire to void, respectively. Further studies using these methods are needed to establish their clinical utility.
Autonomic Nervous System Tests
The electrodiagnostic techniques just discussed assess only the somatic nervous system, whereas the autonomic nervous system is more relevant for sacral organ function. Although focal involvement of the sacral nervous system (trauma or compression) usually involves both somatic and autonomic fibers, in some circumstances the autonomic system is affected in isolation (e.g., after excision of mesorectal carcinoma and prostatectomy ). Furthermore, some systemic disorders affect predominantly the autonomic nervous system.
Sympathetic Skin Response
The SSR is a reflex subserved by myelinated sensory fibers (afferent limb), a complex central integrative mechanism, and sympathetic postganglionic nonmyelinated C fibers (efferent limb). The responses, which can be recorded from the perineum or penis, rapidly habituate and are critically dependent on a number of endogenous and exogenous factors, including skin temperature. The stimulus used in clinical practice is generally an electrical pulse delivered to a peripheral nerve in the limbs, but the genital organs can also be stimulated.
The SSR can be recorded on the penis following stimulation of the median nerve at the wrist in all control subjects; the response is variable in shape and has latencies of approximately 2 seconds. Similarly, the SSR can be recorded from the genital region in women. Only an absent SSR is considered abnormal. The SSR can also be recorded on bladder/urethral stimulation, which in addition to central sympathetic pathways also tests thin afferent fibers from the pelvic viscera. The SSR is reportedly useful in the assessment of neuropathies involving unmyelinated nerve fibers, and in patients with spinal cord injury, it may serve also as an indicator of bladder neck competence. In men it may serve as a predictor of preserved psychogenic erections.
Dartos Reflex
In men, another approach to test lumbosacral sympathetic function is by neurophysiologic measurement of the dartos reflex obtained by cutaneous stimulation of the thigh. The dartos muscle is a sympathetically innervated dermal layer within the scrotum, distinct from the somatically innervated cremasteric muscle. A reliable and reproducible dartos reflex (i.e., scrotal skin contraction) with a latency of about 5 seconds has been demonstrated in healthy men.
Smooth Muscle EMG
Information on parasympathetic bladder innervation can, to some extent, be obtained by cystometry, although this cannot demonstrate directly the neuropathic etiology of the dysfunction. Electrodiagnostic tests of sacral parasympathetic nerve function (e.g., corpus cavernosum EMG, clitoral EMG, and detrusor EMG) would, in principle, constitute the most definitive indicator of neurogenic sacral organ involvement. Further studies to validate these tests would be necessary to clarify their place in research and clinical practice. At present, clinical application of these tests is premature.
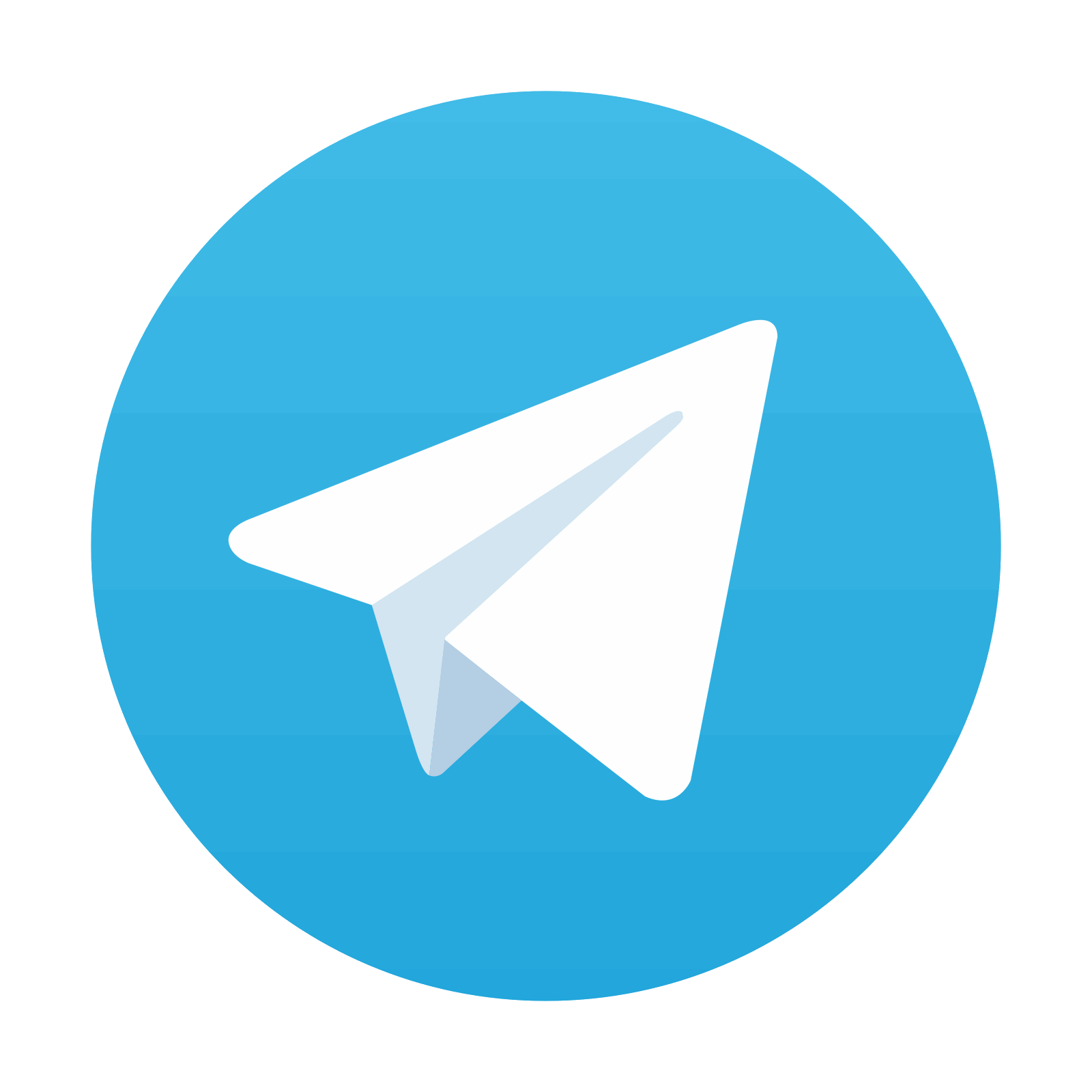
Stay updated, free articles. Join our Telegram channel

Full access? Get Clinical Tree
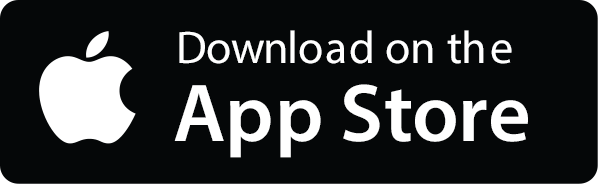
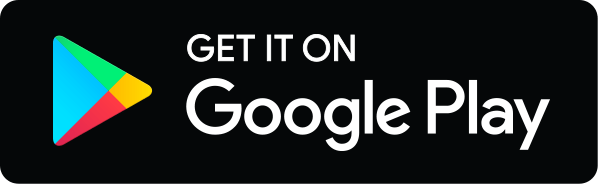