CHAPTER 376 Endovascular Stenting of Intracranial Aneurysms
Overview: Conceptual Evolution and History of Intracranial Stenting for Aneurysms
The impetus for stent-supported aneurysm embolization was the recognition that many wide-necked aneurysms were not amenable to endovascular treatment simply because embolization coils could not be reliably retained within the aneurysm fundus. Although many of these lesions could be successfully treated using a balloon-remodeling technique,1,2 this approach was perceived to be technically demanding, risky, and without an adequate “bail-out” strategy should the coils begin to prolapse into the parent artery during treatment. Intracranial stenting can overcome many of these limitations. These devices can be placed into the parent arteries to provide a durable mesh barrier across the aneurysm neck, which prevents coils from prolapsing out of the aneurysm after detachment.
Initial Applications of Stents for Aneurysm Therapy
Early Experience with Balloon-Expandable Stents
Several years later, Higashida and associates6 described the first successful transstent coiling of a circumferential, fusiform basilar artery aneurysm that could not be coiled using conventional techniques. During the ensuing 5 years, several additional case reports and small case series appeared in the literature describing the off-label applications of balloon-mounted coronary stents (BMCSs) to support the coiling of wide-necked intracranial aneurysms.7–13 During this initial era, stent-supported aneurysm treatment was greatly limited by the rigid nature of the available balloon-expandable coronary stents and the subsequent challenges involved with the delivery and deployment of these devices within the tortuous cerebrovasculature.
Self-Expanding Intracranial Microstents
In 2003, Neuroform (Boston Scientific, Fremont, CA), the first intracranial, microcatheter-delivered, self-expanding nitinol microstent was released in the United States for use in treating wide-necked aneurysms under a Humanitarian Device Exemption. Neuroform and its delivery system are far more flexible than the predicated BMCSs. These characteristics greatly facilitated delivery and deployment within tortuous segments of the cerebrovasculature.14 In 2007, Enterprise (Cordis Neurovascular/Johnson and Johnson, Warren, NJ) was introduced in the United States, providing a second self-expanding intracranial microstent (SEIM) for the treatment of intracranial aneurysms. The Enterprise stent presents several benefits over Neuroform, including ability to be reconstrained, a lower-profile delivery system, and a technically less-complicated deployment mechanism. The introduction of these devices led to a marked increase in the number of stent-assisted aneurysm treatments performed and greatly broadened the scope of lesions amenable to endovascular therapy. As practitioners gained experience with SEIMs, novel approaches to more complex lesions were innovated and the sophistication of endovascular reconstruction increased.15–22 During the past decade, stenting has become a standard adjunctive technique used to facilitate the treatment of wide-necked and complex aneurysms.
Parent Vessel Remodeling
Experimental and Histopathologic Evidence
Given the relative flexibility and low metal surface area coverage (e.g., Neuroform, 6% to 9.5%) of the commercially available SEIMs, the ability of these devices to produce “physiologically significant” parent vessel remodeling could be questioned. Canton and associates23,24 performed a series of experiments to assess the impact of the Neuroform stent on intra-aneurysmal flow. These authors demonstrated that two stents placed in a Y configuration reduced the velocity of the inflow jet by 11% and reduced residual intra-aneurysmal vorticity and shear stress by more than 40%. With respect to biologic remodeling, only a single case is available in the literature describing the histopathologic appearance of an implanted Neuroform stent at autopsy.25 The aneurysm in this case was treated solely by stenting because subsequent attempts to place coils were unsuccessful. After this patient died of unrelated causes 4 months later, an evaluation of the explanted aneurysm demonstrated de novo fibroelastic tissue growing across the aneurysm neck and moderate intimal thickening along the stented segment of the parent vessel.
Clinical Evidence
The available data from clinical case series have provided some additional, albeit preliminary, evidence that stenting may improve the durability of endovascular aneurysm therapy. Aneurysms treated with stents are wide necked, typically larger, and often in a terminal location. These characteristics would predict a very high rate of recurrence with standard coiling techniques. Evaluation of mid- and long-term follow-up results from the collaborative Barrow Neurological Institute–Cleveland Clinic database demonstrated a surprising level of long-term durability in aneurysms treated with stent-support.14,26,26a At an average follow-up of 12.9 months, 72% of aneurysms demonstrated either stability or progressive thrombosis (Fig. 376-4). Of the 28% aneurysms that demonstrated recanalization, defined as any amount of increased filling in comparison to the immediate postcoiling result, many were either very large or giant aneurysms. Small (<10 mm) aneurysms actually recanalized at a very low rate (9.3%), with only 3.1% requiring retreatment.
Several investigators have reported success with stents alone for the treatment of small dissecting and blood blister–like aneurysms, providing additional evidence in support of “physiologically significant” stent-induced remodeling of the parent vessel–aneurysm complex after self-expanding stent implantation.27–33 In the largest series, 10 patients with these types of aneurysms were treated with the Neuroform.29 Nine patients demonstrated interval regression or complete resolution after stent monotherapy (Fig. 376-5), and one was stable after only 1 month of follow-up. No patient experienced aneurysm rerupture during the follow-up period.
The observation of in-stent stenosis (ISS) (Fig. 376-6) in about 5% of patients after Neuroform placement (either as the sole or an adjunctive therapy) further suggests that these devices have the capability to stimulate a significant biologic response after implantation.34 Although the nature of the material accounting for the stenosis observed in these patients remains unknown, the angiographic appearance and time of onset are compatible with the intimal hyperplasia that has been encountered in other vascular beds after stenting for atherostenosis.34
High Metal Surface Area Stents (Flow Diverters)
The concept of parent vessel reconstruction is quickly advancing with the recent development of dedicated flow-diverting endovascular constructs designed for intracranial use. These devices primarily target parent vessel reconstruction, rather than endosaccular occlusion, as the means by which to achieve definitive aneurysm treatment. Currently, these flow-diverting devices are high metal surface area coverage, stent-like constructs (Fig. 376-7) that are designed to provide enough flow redirection and endovascular remodeling to induce aneurysm thrombosis without the use of additional endosaccular occlusive devices (i.e., coils). At the same time, the pore size of the constructs is large enough to allow for the continued perfusion of branch vessels and perforators arising from the reconstructed segment of the parent vessel.35
Commercially Available Devices
Balloon-Expandable Stents
Device Delivery and Deployment
The BMCSs consist of an angioplasty balloon catheter on which a collapsed (but expandable) cylindrical metal cage is mounted. The balloon catheter on which the device is mounted may either be designed for over-the-wire delivery, whereby the microwire exits the proximal hub of the delivery catheter, or monorail delivery, whereby the microwire exits the side wall of the distal aspect of the catheter. Over-the-wire delivery allows for the transmission of greater forward pressure from the proximal microcatheter to the distal aspect. The monorail delivery provides a system that is, in general, easier to use, particularly for a single operator. In most cases, access across the targeted landing zone for stent deployment is achieved with a standard microcatheter and 0.014-inch microwire combination under high-magnification fluoroscopic roadmap control. The microcatheter is manipulated well beyond the targeted landing zone into the distal intracranial vasculature and the microwire is removed. An injection of contrast material through the microcatheter or guiding catheter can be performed to verify an adequate intravascular position of the microcatheter. An exchange-length, 300-cm, 0.014-inch microwire is then placed through the microcatheter, which is then removed. The BMCS is then placed over the microwire and manipulated over this wire and across the targeted landing zone. When the stent is in adequate position, an angiographic run may be performed to verify optimal positioning and to assess for any complications of stent navigation or wire manipulation. When the operator is satisfied with the stent positioning, the stent is deployed through balloon inflation. Inflation of the angioplasty balloon is achieved using a calibrated insufflator. As contrast material is introduced into the balloon, the balloon expands to dilate the stent into the form of a rigid cylinder that is pressed into the adjacent vessel wall (Fig. 376-8). The balloon catheter can then be completely deflated and removed, leaving the deployed stent behind within the artery. At this point, stent-supported aneurysm coiling can be performed.
Device Characteristics
A newer generation of balloon-mounted stents are being designed for intracranial use. These devices have been engineered to be more deliverable and less traumatic than the predicate devices designed for coronary applications. These devices may have indications for the treatment of both cerebral aneurysms and intracranial atherosclerosis.36–38
Current Applications
Clinical Experience
Because of issues with delivery and deployment, most reported experiences with balloon-expandable stents have been limited to relatively small series.7,8,11,39,40 Even the highest-volume centers performed only small numbers of aneurysm embolizations using these devices during the late 1990s and into the next decade. Han and associates7 reported technical success in 10 of 13 patients undergoing attempted coronary stent–assisted aneurysm treatment over 8 years at the Barrow Neurological Institute. In the three cases in which attempted stent placement failed, the stent could not be navigated through the tortuous anatomy of the carotid siphon. Two patients in this series sustained permanent neurological injury as a result of attempted stent placement. Aneurysm recurrence was noted in two of the five patients in this series for whom angiographic follow-up was available.
Lylyk and colleagues31 reported the largest series of BMCS used for cerebral aneurysm treatment, composed of 62 wide-necked saccular aneurysms and 10 dissecting or fusiform aneurysms. By effectively selecting cases, these operators were able to place these balloon-mounted stents with a technical success rate of 90%. At the 3- to 6-month follow-up evaluation, these authors observed a 92% rate of complete or near-complete occlusion for saccular aneurysms and a 100% rate of complete or near-complete occlusion for fusiform aneurysms. Incidentally, this series included 13 aneurysms that were treated with stents alone, of which 5 progressed to complete thrombosis without coiling, demonstrating the effectiveness of endovascular remodeling in achieving aneurysm occlusion even without endosaccular embolization coils.
Self-Expanding Intracranial Microstents
Neuroform
Stent delivery is achieved in a manner similar to that of the BMCS. Access into the cerebrovasculature distal to the targeted landing zone is achieved with a standard microcatheter and 0.014-inch microwire. The microcatheter is then removed over a floppy 300-cm, 0.014-inch wire (e.g., Transcend, Boston Scientific, Fremont, CA; or Xcelerator, ev3 Endovascular, Irvine, CA), which maintains access within the distal vasculature. The entire Neuroform delivery apparatus is then navigated over the exchange microwire to the targeted landing zone within the intracranial vasculature. Stent deployment is achieved by fixing the stabilizer in position and withdrawing the microcatheter to release the stent, which expands to its preset cylindrical morphology (Video 376-1, Part 1).
The devices come in sizes ranging between 2.5 and 4.5 mm in diameter and 10 and 30 mm in length. The recommended diameter for placement is 0.5 mm greater than the largest diameter of the parent artery to be stented. The length is chosen such that the stent extends for at least 5 mm proximal and distal to the aneurysm neck. The struts composing the stent measure about 60 µm in thickness. The cell size is large enough to accommodate the passage of a 2F microcatheter through the interstices, allowing trans-stent coiling of the aneurysm after placement. The ends of the stent are demarcated by four radiopaque markers (Fig. 376-9). The body of the stent is essentially radiolucent using standard fluoroscopy. After stent deployment, the operator is left to intuit the position and configuration of the stent by approximating a cylindrical construct within the confines of the vascular anatomy. Often, resistance is felt when traversing the stent with the microcatheter, leaving the operator to perform the trans-stent catheterization primarily by feel.
The stent structure is so delicate that individual cells can sometimes be disrupted or displaced during microcatheter traversal. In addition, the chronic outward radial force holding the stent in position is sometimes inadequate to prevent migration during microcatheter traversal of the device. When the stent is placed in curved anatomy, the stent cells are prone to opening, producing gaps in stent coverage along the outer curvature of the vessel.41,42 These gaps can conceivably lead to incomplete coverage of the aneurysm neck and coil prolapse from the aneurysm into the parent artery during embolization.
Enterprise Vascular Reconstruction Device and Delivery System
The Enterprise measures 4.5 mm in diameter when unconstrained and as such is only indicated for use in vessels measuring between 2.5 and 4 mm in diameter. The device comes in 14-, 22-, 28-, and 37-mm lengths. The struts of the Enterprise, like those of the Neuroform, are about 60 µm thick. The stent ends are demarcated by four radiopaque markers; the cage portion of the stent is also entirely radiolucent. Enterprise is a closed-cell stent. This provides several key advantages. First, before full deployment, the device is reconstrainable—if the operator judges the deployment of the stent to have started in a suboptimal position, the stent can be reconstrained and repositioned. Second, the closed-cell design prevents the stent from splaying open along the outer curvature of vascular bends. Third, the closed-cell design results in the incorporation of each cell into the entire device structure, making the individual cells more durable and less likely to become damaged during attempted traversal. At the same time, the loss of segmental flexibility created by the continuous closed-cell structure may result in the device kinking or forming a “cobra head” configuration around tight vascular curves (Fig. 376-10), potentially resulting in poor vessel wall apposition and suboptimal parent vessel protection.15,41 The interstices between stent struts are also smaller and less deformable in some anatomic configurations, making the traversal of the device with a microcatheter more difficult in some situations. Finally, although the closed-cell structure provides higher radial resistive force (i.e., resistance to outward compression once deployed), it also exerts less chronic outward force (i.e., outward pressure on the vessel wall), potentially making it more prone to migration during attempted catheterization of the aneurysm or, in some anatomic configurations, spontaneously.43
Current Indications
The SEIMs have become incorporated into routine clinical practice for the treatment of wide-necked intracranial aneurysms. The availability of these devices has increased the scope of lesions amenable to endovascular therapies.14,44 As they have become available, an increasing number of different applications of these devices have been innovated.
Stent-Assisted Coil Embolization
There are two general approaches to standard stent-assisted coil embolization—trans-stent coiling and “jailing”45 (Video 376-1, Part 2).
Rescue and Salvage
During coil embolization, it is possible for detached coils or the entire coil mass to become inadvertently displaced from the aneurysm into the parent artery. In these cases, a SEIM can be navigated across the herniated coil and deployed to either displace the coil mass back into the aneurysm fundus or tack up the displaced strands of coil against the vessel wall to prevent further migration and decrease their intravascular surface area available to function as a source of distal emboli44,46 (Fig. 376-11).
Balloon-Assisted Coiling Followed by Stenting
Some operators prefer to begin the coiling of wide-necked aneurysms using a balloon-assisted technique, with stent placement occurring later during the case. In these cases, the stent is placed after coiling to stabilize the coil mass and avoid delayed migration of coils into the parent vessel, to act as a scaffolding within the parent artery to facilitate endovascular remodeling of the aneurysm neck, and potentially to induce some degree of flow redirection (Fig. 376-12).
Advanced Techniques
Y Stent
In some cases, a single stent is insufficient to provide parent vessel protection for coil embolization. This situation is most commonly encountered with bifurcation aneurysms arising from the basilar apex or carotid terminus. In these instances, two stents can be placed, the first extending out one limb of the bifurcation and the second introduced through the interstices of the first stent, extending into the other limb of the bifurcation. This configuration forms a Y-shaped construct at the bifurcation and provides robust support for the coil embolization of terminal aneurysms.16,21,22 This Y-stent technique has also been applied to treat middle cerebral artery aneurysms21 and anterior communicating artery aneurysms (Henry H. Woo MD, personal communication, 2006) (Figs. 376-13 and 376-14).
Stenting across the Circle of Willis
In some cases, the creation of a Y construct is challenging or impossible owing to the anatomy of the vessels as they arise from the distal bifurcation. In these cases, it is sometimes possible to accomplish a reconstruction of a terminal bifurcation aneurysm by placing a stent across the circle of Willis—such as from P1 to P1 through the posterior communicating artery, from A1 to M1 through the anterior communicating artery, or from the ipsilateral A1 to contralateral A1 through the anterior communicating artery.19 This technique provides a means by which to achieve protection of both limbs of the bifurcation with a single SEIM (Fig. 376-15).
Waffle-Cone Technique
Rarely, Y-stent reconstruction and stenting across the circle of Willis are not technically feasible for the treatment of a wide-necked terminal aneurysm. In these situations, some investigators have deployed a stent from the parent artery directly into the aneurysm (i.e., “intra-extra-aneurysmal stent placement”) to achieve parent artery protection. Using this technique, a single stent can be used to stabilize an intra-aneurysmal coil mass.47 However, the final construct sets up a vector of flow redirection directly into the terminal aneurysm sac and actually disrupts flow into the bifurcation branches. One might expect that such a construct could lead to high rates of recanalization. In addition, if this technique fails or leads to recanalization, other available means of treatment (surgical clipping, endovascular therapy with balloon remodeling, or Y-stent reconstruction) are made considerably more difficult, if not impossible.
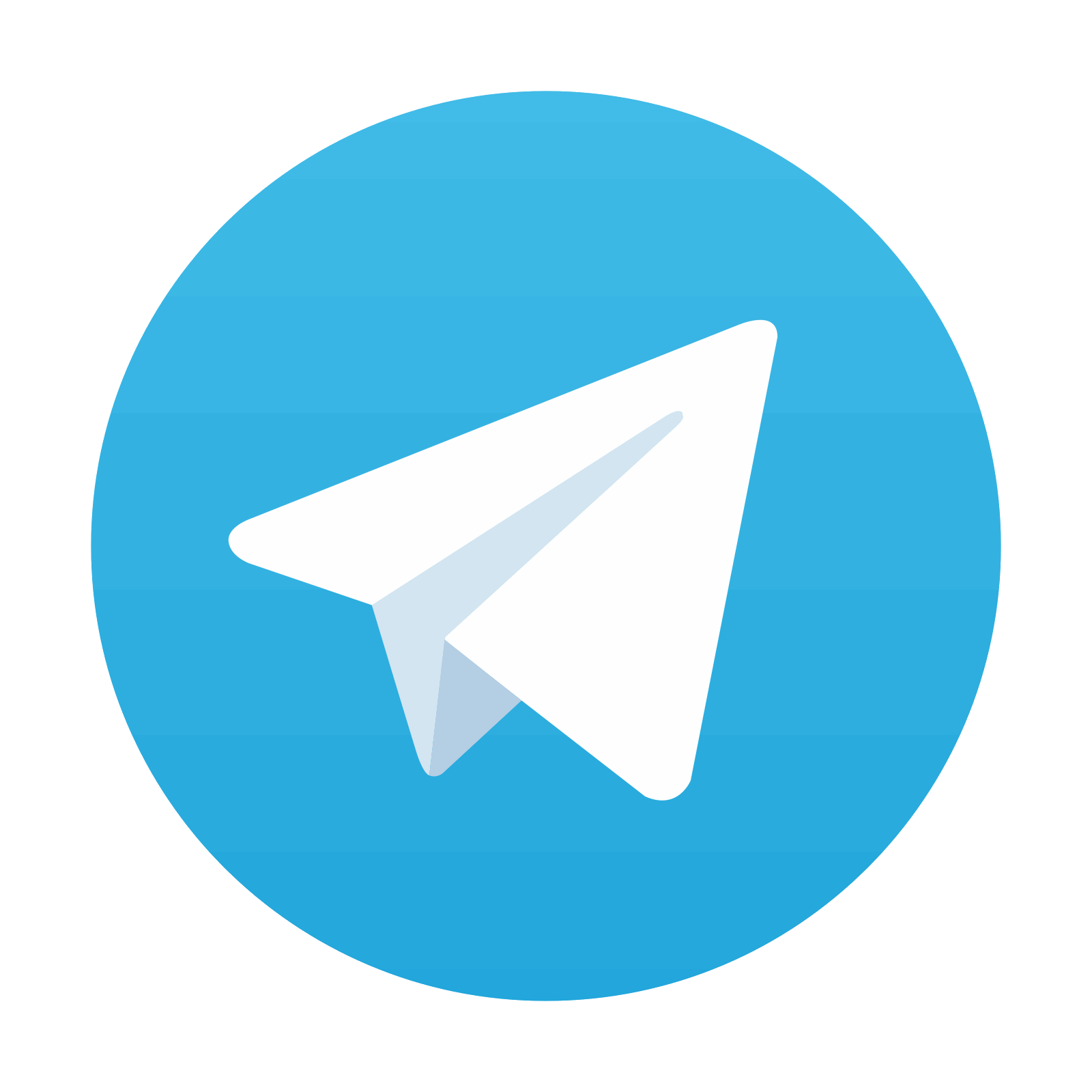
Stay updated, free articles. Join our Telegram channel

Full access? Get Clinical Tree
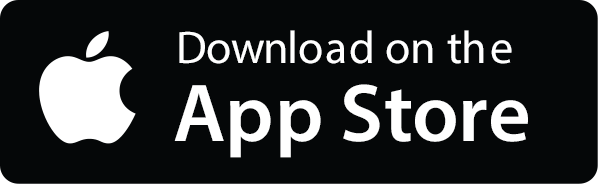
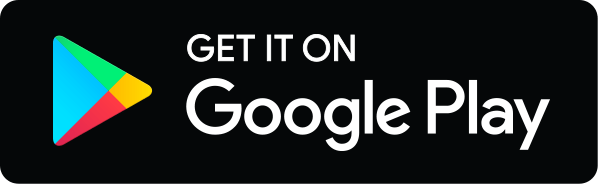