10 Primary neoplasms of the spine, spinal cord, and spinal peripheral nerves are significantly less common than their counterparts in the brain (Table 10-1). Because of the rarity of these tumors, no prospective randomized studies have been performed in these groups of patients, and management is based on institutional retrospective reports. In some instances, the use of radiation therapy is so infrequent that one must extrapolate from the experience for the same neoplasms in the intracranial cavity. This chapter deals exclusively with the use of conventionally fractionated radiation therapy over several weeks in the management of primary neoplasms in this region. Other chapters review brachytherapy and stereotactic radiosurgery for spinal tumors.
External Beam Radiation Therapy of Tumors of the Spine, Spinal Cord, and Peripheral Nerves
Location | Incidence (%) |
Extradural | 20 |
Intradural extramedullar | 60 |
Intradural intramedullary | 20 |
Radiation Biology and Physics
A brief review of radiation biology and physics is appropriate for the nonradiation oncologists. Several excellent chapters on this topic appear in general oncology textbooks; accordingly, this section discusses only the fundamental concepts of radiation therapy in an effort to give readers a basic understanding of the topic.
The radiation therapy used to manage tumors in humans possesses sufficient energy to strip electrons from cells; hence the term ionizing radiation. As a result of removing electrons from atoms, highly reactive radicals form to cause immediate chemical reactions. Because water is the most ubiquitous compound in the human body, the most commonly formed radical is the highly reactive hydroxy radical. It is thought that deoxyribonucleic acid (DNA) is the critical cellular target, and hydroxy radicals that form in the vicinity of DNA in the cell nucleus, damage the DNA. At least four postradiation effects on cellular viability are known to occur: necrosis, apoptosis, accelerated senescence, and terminal differentiation. These effects may occur immediately or in a delayed fashion, depending on how mitotically active the cells are.
As a consequence of the instantaneous chemical damage done to cells during irradiation, the biologic effects of irradiation may be delayed days, weeks, or months, often mirroring the level of mitotic activity in the specific cell population, both for normal and cancerous tissue.
Daily small fractions administered over several weeks yield superior rates of tumor control and decrease rates of normal tissue complications associated with radiation therapy. The use of small daily fractions is based on the radiobiologic principles underlying radiation therapy, including repair of sublethal damage, reoxygenation, cell-cycle progression, and repopulation during the course of several weeks of radiation therapy.
In most instances, radiation therapy is given using photons, but occasionally subatomic particles such as protons or electrons are used. The penetrating power of radiation is highly dependent on its energy, and modern linear accelerators yield photons in the range of 4 to 25 million electric volts (MeV). Such energetic photons have the capacity to penetrate to the appropriate depths in the body to treat tumors of the spinal region. The second most important factor in the management of spinal tumors is the ability to use radiation beams originating from several different directions. All beams from the different directions are aimed at the tumor volume but enter and leave the body through separate paths. Therefore, high-dose radiation is delivered to the tumor target, which is caught in the crossfire. Concomitantly, by entering and exiting though different normal tissues the radiation dose to normal tissues is minimized (Fig. 10-1).
The basic principles involved in determining the specific volume of tissue to be targeted for a radical course of radiation therapy can be summarized into three processes: the location of the tumor, where there may be microscopic spread of tumor, and how to minimize the radiation dose to normal tissues in this volume.
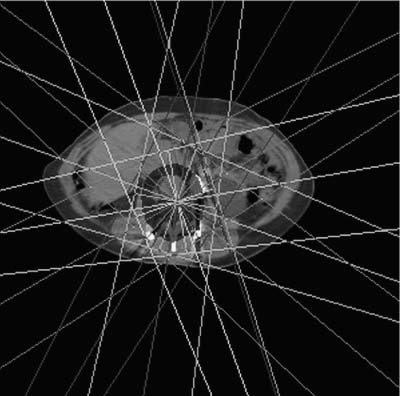
FIGURE 10-1 A prone axial computed tomography (CT) planning scan of a patient with a chordoma of a lumbar vertebrae. Seven different beams from different directions result in a high-dose volume that incorporates the primary tumor and additional volume to deter possible microscopic spread.
The location of the tumor is determined by obtaining a complete history and physical examination, obtaining the appropriate blood tests, and most critically, obtaining the appropriate imaging to appreciate the three-dimensional (3D) extent of macroscopic tumor and its relationship to normal tissues.
Where tumor might have spread microscopically relates to the tumor’s specific histopathology, its anatomic compartment of origin, and other tissues that it may have invaded. For example, a malignant glioma of the cerebellum is associated with a low likelihood of spreading via the cerebrospinal fluid (CSF). Therefore, the appropriate high-dose volume would be the primary tumor plus a few centimeters of adjacent normal brain to account for microscopic spread. Alternatively, a medulloblastoma arising in exactly the same area of cerebellum is associated with a very high likelihood of spreading via the CSF. Even if a magnetic resonance imaging (MRI) study of the brain and spine and CSF cytology show no evidence of CSF spread, the initial high-dose volume would include the entire craniospinal axis for potential undiagnosed microscopic spread.
Once the volume of high-dose radiation therapy is determined from the perspective of controlling the tumor, whatever normal tissues are part of this volume must be assessed. The first step involves using several beams from different directions to target the tumor and tissues microscopically involved by tumor in an effort to minimize the radiation dose to adjacent normal tissues. The next step usually involves introducing shielding materials into the radiation beams to shape the radiation volume to exclude or minimize the dose delivered to normal tissues (Fig. 10-2). Often, a certain radiation dose must be delivered to control microscopic disease, after which the radiation beams can be reduced to focus on the macroscopic tumor for the final boost dose. In many instances normal tissue is shielded after a certain radiation dose has been reached in an effort to keep the risk of severe long-term damage to an acceptable level, even if it means accepting a lower likelihood of tumor control.
Another method of delivering radiation therapy is with the use of charged particles, the most common example being protons. Protons travel a specific distance within tissue and combine with nuclei as they slow down near the end of their path. The result is the release of high-energy nuclear fragments that travel only a very short distance in tissue. A very large amount of energy is deposited at the end of their path at a predictable and finite depth in tissue such that these beams can be made to stop in front of a critical tissue adjacent to tumor. Because of these characteristics, proton beam therapy can be used to deliver high doses of radiation therapy to tumors situated adjacent to critical normal tissues, and this ability may improve the local control in some instances; however, because of the mass of the protons relative to photons, which have no mass, high-energy cyclotrons in the range of 250 MeV are required to accelerate protons to sufficient energy to penetrate the body at an appropriate depth. In addition, protons have a relative biologic efficiency that differs from photons. The dose for protons is usually referred to as cobalt gray equivalent (CGE), or equivalent to a dose of cobalt radiotherapy that would yield the same biologic effect on tissue.
FIGURE 10-2 A beam’s-eye view of a digitally reconstructed radiograph of one of the fields from Figure 10-1. The field incorporates the gross tumor volume (1) and the clinical target volume for microscopic spread (2) and utilizes shielding in both superior corners to avoid irradiating the kidneys.
A recent development in radiation therapy is the concept referred to as intensity-modulated radiation therapy (IMRT). Traditionally, the tumor would be placed at the center of radiation beams, and shielding would be introduced at the periphery of the radiation fields to shape the field to the shape of the tumor. IMRT requires the radiation oncologist to determine where the radiation dose is to be distributed both within and around the tumor and surrounding normal tissues. The computer program then uses a series of radiation beams from multiple directions and modifies the intensity of individual beamlets (subsegments of the whole beam) within the larger radiation field by moving shielding material in and out of the radiation field. This process modulates the intensity of the radiation beam to deliver the correct amount of radiation to the desired location while minimizing radiation dose in normal structures. Radiation distributions, therefore, conform the high-dose volume to the shape of the intended volume. In many instances the outcome represents a marked improvement compared with what could be achieved with the more conventional approach. IMRT is just beginning to be introduced and used in clinical departments.
Vertebral Body Tumors
Plasmacytomas
Solitary plasmacytomas of the bone represent a localized form of multiple myeloma in which local radiation therapy may be curative. Plasmacytomas involve vertebral bodies in a third of the patients and frequently affect men (70%) at a younger age (median, 56 years).1 Extramedullary (extraosseous) plasmacytomas are diagnosed less frequently, and these patients require an extensive staging evaluation to rule out disseminated disease. The optial therapy for true solitary plasmacytoma is curative-dose radiotherapy in the range of 4000 to 5000 cGy.2–4 Liebross et al4 reported 57 patients with solitary bone plasmacytomas treated from 1965 to 1996 at the M. D. Andersen Cancer Center. In 23 of these cases, the primary tumor involved the thoracolumbar spine. The radiation dose ranged from 30 to 70 Gy (median 50 Gy), and local control was achieved in 55 of 57 (96%) patients. Of the 57 patients, disseminated relapse (development of multiple myeloma) occurred in 29 (51%). In eight patients staged only with plain radiographs of the spine, seven went on to develop multiple myeloma, whereas of seven patients whose spine was imaged with MRI at diagnosis, only one developed multiple myeloma. This finding supports the importance of including MRI of the whole spine during diagnostic evaluation to rule out the involvement of additional vertebrae. Such involvement represents disseminated disease in which aggressive local radiotherapy would be inappropriate.
McLain and Weinstein5 reviewed 84 cases of solitary plasmacytoma of the vertebral column in the literature and found a 5-year disease-free survival rate of 60% and supported definitive radiotherapy as the treatment modality of choice for this patient population.
Chordomas and Chondrosarcomas
Chordomas are rare neoplasms of notochord remnants that almost exclusively arise in the skull base, sacrum, and least frequently, the mobile spine. Typically, chordomas are slow-growing neoplasms. They only rarely metastasize but are frequently lethal due to uncontrolled local progression. These tumors are either immediately adjacent to or involve critical skeletal and neural structures. Consequently, complete surgical resection is rarely achieved. Postoperative radiation therapy is almost always indicated in an effort to control gross residual or microscopic residual disease. Within the spine, the most common location is the sacrum, and the least common site is the mobile spine, most often the lumbar spinal vertebrae (Figs. 10-3 and 10-4).
York et al6 reported a 40-year experience with sacral chordomas in 27 patients (19 males, eight females) at the M. D. Anderson Cancer Center. Most patients underwent several resections each. Altogether these 27 patients underwent 28 radical and 39 subtotal resections. Conventional photon radiation therapy was delivered in 13 cases. The median disease-free survival was 2.27 years for the radical resections and 8 months for the subtotal resections. The addition of radiation to the subtotal resections increased the median disease-free survival from 8 months to 2.12 years.
Boriani and colleagues7 reported 21 cases of chordoma of the mobile spine above the sacrum. Ten patients died of disease, seven were alive with disease, and four were alive with no disease activity. Of 15 patients who received conventional photon radiation therapy after incomplete resections, 12 developed local recurrences. The three patients with no recurrent disease all had undergone complete excisions. Two of four patients alive with no disease recurrence also received postoperative adjuvant radiation therapy. Similar results have been reported for spine chordomas.8,9
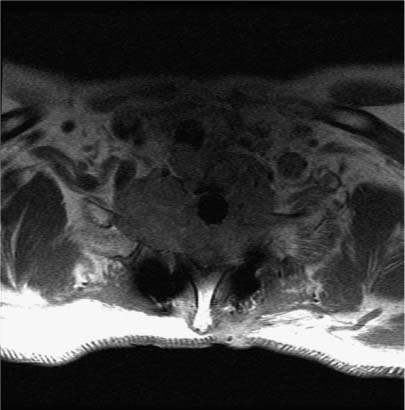
FIGURE 10-3 Sagittal T1-weighted MRI of a 60-year-old woman with a chordoma of her third lumbar vertebra.
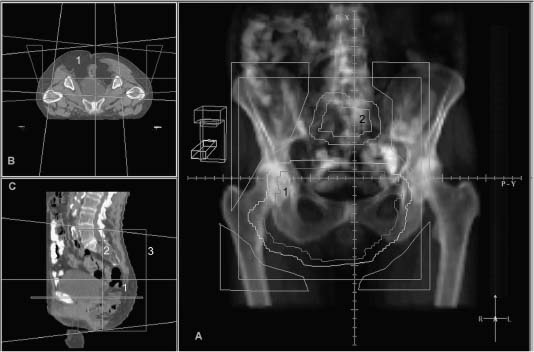
FIGURE 10-4 Seventy-five year old female with multiple recurrences of a sacral chordoma. Panel A is a digitally reconstructed radiograph from the planning CT which demonstrates a beam’s eye view of the posterior field which encompasses the 2 separate recurrent masses (volume 1 in sacrum, volume 2 in lumbar region), with corner shielding to shape the high dose volume. Panel B demonstrates the 3 fields utilized to treat this patient on an axial CT image: a posterior and two lateral fields encompassing the sacral tumor (1). Panel C is a view of the lateral field on a sagittal reconstructed image (3).
Chondrosarcomas of the spine are also rare neoplasms of the vertebra managed much like chordomas. Shives et al10 reported 20 patients managed at the Mayo Clinic. Their median survival was 6 years, and 15 patients died of locally progressive disease. Postoperative radiation therapy in various doses was delivered in only five patients. York and colleagues11 reported 21 patients managed at the M. D. Anderson Cancer Center. Of 28 surgical procedures, seven were radical procedures and 21 were subtotal excisions. Radiation therapy was administered after 10 of the 28 surgical procedures. A total resection was associated with a statistically significant disease-free interval, and the addition of postoperative radiation therapy increased the median disease-free survival from 16 to 44 months; however, this outcome did not reach statistical significance because of the small sample size. Boriani et al12 reported 22 cases of vertebral chondrosarcoma in which 33 surgical procedures had been performed (18 partial resections and 15 en bloc resections). Of 14 patients who underwent en bloc resections initially or at recurrence, 11 remain free of recurrence. In contrast, all 10 patients who underwent partial resections eventually developed a recurrence, with no reported benefit from postoperative radiation therapy.
The best results for chordoma and chondrosarcomas are achieved when attempts at a radical resection are combined with high-dose postoperative particle radiation therapy using 3D treatment planning. Hug et al13 reported that of 14 patients with chordomas and of six patients with chondrosarcomas of the axial skeleton, local control was achieved in nine and six patients, respectively, with combined high-dose photon/proton radiotherapy. The median dose was 74.6 CGE (range, 67.1 to 82.0) for the chordoma group and 72.2 CGE (range, 66.1 to 77.9) for the chondrosarcoma group. Berson et al14 reported 45 patients with chordomas and chondrosarcomas of the base of skull and cervical spine treated at the University of California Lawrence Berkeley Laboratory with helium ion or neon beams. The 5-year actuarial local control and disease-specific survival were 59% and 62%, respectively. The 2-year local control rate for those treated at initial diagnosis was 78% compared with 33% for those treated at recurrence.
The preceding series demonstrate several principles in the management of chordomas and chondrosarcomas of the spine: Disease-free survival is longer with radical than with subtotal resections. Most patients eventually develop a recurrence despite apparent completeness of surgical resection. Postoperative conventional photon radiation therapy increases the length of disease-free survival, although most patients eventually fail because of a local recurrence. Finally, results are better for chondrosarcomas than for chordomas. These series, however, spanned many decades. As a result the quality of the radiation therapy may not reflect the possible benefits that modern CT-based conventional radiation therapy would offer today. Nonetheless, any form of radiation therapy has a lower likelihood of controlling gross residual disease than radiation therapy administered adjuvantly for microscopic residual disease. The exception to this principle would be the results of high-dose combined photon/proton radiotherapy for gross residual chondrosarcoma. In this case the long-term control rates are quite high and radiotherapy is considered the standard of care for chordomas and chondrosarcomas.
Ewing’s Sarcoma
Ewing’s sarcomas of the spine are rare neoplasms that most often occur in children and young adults. Venkateswaran et al15 reported that of 344 primary Ewing’s tumors seen at St. Jude Children’s Research Hospital from 1962 to 1999, 33 (10%) occurred in the vertebrae. Of the 33 patients, six had metastatic disease at presentation. The most common site was the sacrum in 13, followed by thoracic vertebrae in 10, the lumbar in eight, and the cervical in two patients. Grubb et al16 reported 36 primary vertebral Ewing’s sarcoma seen at the Mayo clinic from 1951 to 1988. The mean age was 17 years (range, 5 to 40 years).
Ewing’s sarcomas are presumed to be associated with micrometastases in all instances even when staging investigations are negative. These tumors are exquisitely sensitive to both chemotherapy and radiation therapy. Accordingly, initial surgical approaches are limited to biopsy only, followed by intensive systemic chemotherapy for 12 weeks, followed by local management. A substantial response is usually achieved. For nonvertebrae sites, a surgical approach is usually undertaken because the more peripheral extremity and rib primaries are resectable. This issue is particularly important in younger children in whom radiation should be avoided because of the subsequent effects of radiation on growth and possible radiation carcinogenicity. In the vertebrae, however, gross total resection with microscopically clear margins is seldom achieved as a result of the invasion and involvement of critical neurologic structures. For that reason primary radical radiation therapy is generally undertaken.
Radiation therapy is currently delivered to a dose of 5580 cGy in 31 fractions. The initial 4500 cGy is initially delivered to a volume encompassing the original extent of the bony and soft tissue disease with a 2-cm margin. This dose is followed by a boost to a total dose of 5580 cGy to the original disease with a 1-cm margin. The major critical normal structure within the high-dose volume is the spinal cord for cervical, thoracic, and upper lumbar primaries. This consideration may limit the prescribed dose to 4500 to 5000 cGy. Various techniques have been utilized over the years, but currently, the use of multiple beams, either via proton-beam radiotherapy or IMRT is the preferred approach.
The overall survival and event-free survival rates from St. Jude’s for the 33 patients were 48.1% and 35.6%, respectively.15 The Mayo Clinic reported a 5-year survival of 33% for their 36 vertebral body Ewing’s sarcomas.16 Barbieri and colleagues17 reported a 5-year survival rate of 43.5% with radiation therapy and chemotherapy from a series of 28 cases of Ewing’s sarcoma of the vertebrae with no metastatic disease at presentation.
Osteosarcomas
Osteosarcomas of the vertebrae are uncommon primary sites and account for only 0.85 to 2% of all patients with an osteosarcoma.18,19 For osteosarcomas of bone, primary surgical resection is performed in combination with preoperative or postoperative chemotherapy. 20 The experience with the use of radiation therapy for definitive primary control is small, but in general osteosarcomas are regarded as a relatively radioresistant disease.20 Shives et al21 reported 27 patients with a primary osteogenic sarcoma of the vertebrae seen at the Mayo Clinic between 1909 and 1975. Of the 27 patients, 21 received radiotherapy in various dosages and only five received chemotherapy. All but one patient died of disease, with a median survival of 10 months. Barwick and coworkers19 reported 10 patients with vertebral osteosarcomas. Nine patients underwent resection and postoperative radiation therapy with doses between 25 and 36 Gy. Of these 10 patients, nine died of disease within 2 years. These latter two series are characteristic of the older literature on spinal osteogenic sarcomas in which, by today’s standards, inadequate radiation therapy was delivered.
More recently, Hug and colleagues13 in Boston reported local control in 11 of 15 patients with primary osteogenic sarcoma of the axial skeleton and a 5-year survival rate of 44% associated with a combined photon-proton radiotherapy with a 3D approach to treatment planning.13 Seven cases involved the skull base, and the other eight cases involved the vertebrae. The dose ranged from 61.1 to 80.0 CGE, with a mean dose of 69.8 CGE.
In summary, clinical results with highly conformed high-dose radiation volumes using recent innovative techniques (protons, IMRT) can be associated with long-term local control and survival in a proportion of patients with vertebral body osteosarcomas.
Spinal Cord Tumors
Intramedullary spinal cord tumors are rare, but the most common pathologies include astrocytomas, gangliogliomas, ependymomas, and, much less frequently, hemangioblastomas. In a single institutional series of 294 intramedullary tumors, 117 occurred in a pediatric population and 177 occurred in adults.22 Of the 117 pediatric tumors, 49 (42%) were astrocytomas (45 fibrillary: 32 low grade, 10 anaplastic, three glioblastoma, and four pilocytic), 41 (35%) were neuronal tumors (31 gangliogliomas), and 19 (16%) were ependymomas. Of the 177 adult tumors, 93 (53%) were ependymomas, 44 (25%) were fibrillary astrocytomas (22 low grade: 21 anaplastic and one glioblastoma), and only 10 (6%) were gangliogliomas.
Gliomas (Non-Ependymomas)
Intramedullary spinal cord tumors are rare tumors derived from the spinal neuroectodermal cell population. Histologic subtypes include astrocytomas, malignant astrocytomas (glioblastomas multiforme, anaplastic astrocytomas), and, much less frequently, oligodendrogliomas, mixed gliomas, and mixed glial-neural neoplasms.
Gliomas of the spinal cord tend to occur in younger patients than their brain counterparts. In the Princess Margaret Hospital (PMH) experience of 52 cases, the median age was 32 years and 25% of the patients were younger than 18 years old.23 Because of the infiltrative nature of these neoplasms, complete excision of spinal gliomas with acceptable postoperative rates of neurologic morbidity can be difficult to achieve. Accordingly, subtotal resection or biopsy is more common (Fig. 10-5). For this reason postoperative radiation therapy is offered to most patients.
Radiation doses usually range between 50 to 50.4 Gy in 2- or 1.8-Gy fractions daily over 5 to 6 weeks via a direct posterior photon beam (Fig. 10-6). No doseresponse relationship is present in the ranges of 35 to 50 Gy and >50 Gy; however, identifying dose-response relationships is hampered by the small number of patients treated and by the inability to control for all known and unknown prognostic factors associated with these rare neoplasms.
A review of the literature on the results of radiation therapy for spinal cord gliomas, which included a mixture of adult and pediatric patients, yielded 11 reports that included seven to 52 patients, with an overall 5-year survival rate ranging from 43 to 59%.23–34 Two additional pediatric series of 32 and 15 cases reported overall 5-year survival rates of 67% and 86%, respectively.35,36
Major favorable prognostic factors include younger age, low-grade primary tumors, and long presenting symptom intervals. In the PMH series, the 5-year cause-specific survival rates for patients 18 years old or younger and for older patients were 100% and 56%, respectively. Comparable rates for patients with low-grade gliomas and high-grade gliomas were 73 and 30%, respectively.23
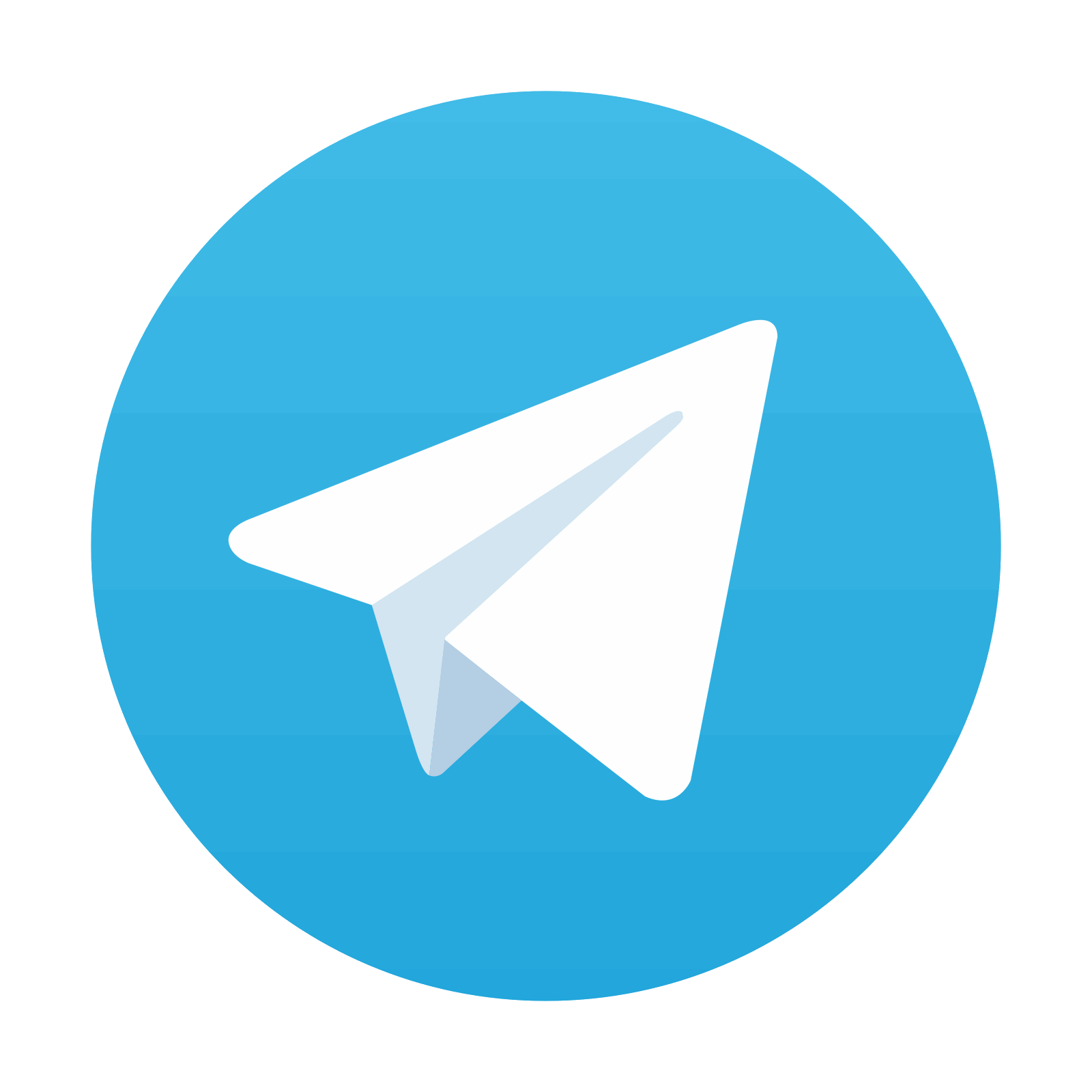
Stay updated, free articles. Join our Telegram channel

Full access? Get Clinical Tree
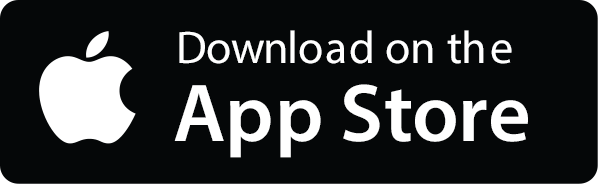
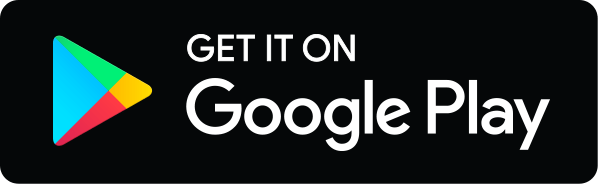