Fig. 1.1
Basal ganglia. Human basal ganglia shown in a frontal section and in a lateral view (inset). The basal ganglia receive cortical inputs and return processed information to the cerebral cortex through the thalamus (cortico-basal ganglia loop) and to the brain stem (Modified from Nambu (2009) with permission)
1.
The striatum, which comprises the caudate nucleus, putamen, and ventral striatum (including the nucleus accumbens and olfactory tubercle).
2.
The globus pallidus, which consists of the external (GPe) and internal (GPi) segments.
3.
The subthalamic nucleus (STN).
4.
The substantia nigra, which is divided into the pars reticulata (SNr) and pars compacta (SNc).
The above nuclei can be grouped functionally into four categories.
1.
Input nuclei: striatum and STN, which receive cortical inputs.
2.
Output nuclei: GPi and SNr, which project outside the basal ganglia to the thalamus and the brain stem.
3.
Connecting nucleus: GPe, which connects the input nuclei to the output nuclei.
4.
Modulatory nucleus: SNc, which modulates the activity of the basal ganglia.
The caudate nucleus and putamen can be considered as a continuum, as they share similar morphological and electrophysiological properties. Actually, they form a single entity in rodents. The striatum of primates and carnivores is separated by dense fiber bundles of the internal capsule into the caudate nucleus and putamen. The caudate nucleus receives inputs mainly from the prefrontal cortex and the putamen from the motor cortices. The GPi and SNr can also be considered as a continuum separated by the internal capsule. In rodents and carnivores, the GPi is small and buried in the fibers of the internal capsule, thus classically named the entopeduncular nucleus, and the GPe is called simply as the globus pallidus.
The basal ganglia receive inputs from wide areas of the cerebral cortex. Information processed in the basal ganglia returns mainly to the cerebral cortex, especially to the frontal lobe, via the thalamus (Fig. 1.1). Thus, the basal ganglia and the cerebral cortex form a loop circuitry (cortico-basal ganglia loop) (Alexander et al. 1986; Albin et al. 1989; Alexander and Crutcher 1990; Middleton and Strick 2000). Through this loop, the basal ganglia control cortical activity. Minor output of the basal ganglia descends to the brain stem.
1.3 Circuitry of the Basal Ganglia
The striatum as the input nucleus receives excitatory glutamatergic inputs from the wide areas of the cerebral cortex. Striatal projection neurons can be classified into two groups based on projection sites and neurotransmitters. Information in the striatum is transferred to the output nuclei, GPi/SNr, via following two different pathways (Fig. 1.2) (Albin et al. 1989; Alexander and Crutcher 1990).
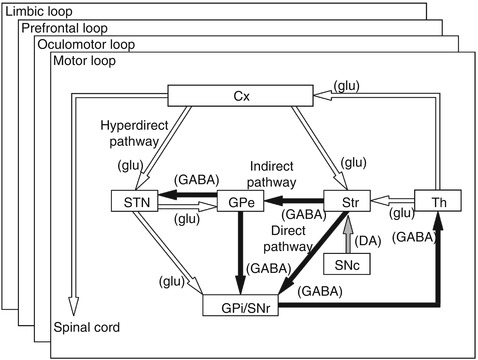
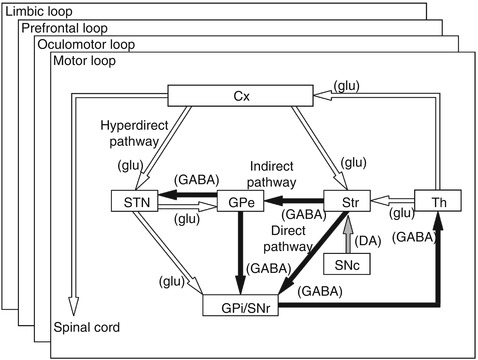
Fig. 1.2
Basic circuitry of the basal ganglia. Open and filled arrows indicate excitatory and inhibitory projections, respectively. Cx cerebral cortex, DA dopamine, GABA gamma-aminobutyric acid, glu glutamate, GPe and GPi external and internal segments of the globus pallidus, SNc substantia nigra pars compacta, SNr substantia nigra pars reticulata, STN subthalamic nucleus, Str striatum, Th thalamus (Modified from Nambu et al. (2002a) with permission)
1.
Direct pathway: striatal neurons containing gamma-aminobutyric acid (GABA), substance P, and dopamine D1 receptors project monosynaptically to the GPi/SNr.
2.
Indirect pathway: striatal neurons containing GABA, enkephalin, and dopamine D2 receptors project polysynaptically to the GPi/SNr by way of sequential connections with the GPe (composed of GABAergic neurons) and STN (composed of glutamatergic neurons).
Signals through the direct pathway have inhibitory effects on GPi/SNr neurons. On the other hand, signals through the indirect pathway have net excitatory effects on GPi/SNr neurons, because both striato-GPe and GPe-STN projections are inhibitory and STN-GPi/SNr projections are excitatory.
3.
Hyperdirect pathway: STN neurons receiving cortical inputs project monosynaptically to the GPi/SNr. The hyperdirect pathway conveys strong excitatory signals from the cortex to the GPi/SNr with shorter conduction time than the direct and indirect pathways.
The output nuclei of the basal ganglia, GPi/SNr, are composed of inhibitory GABAergic neurons and project to the ventral anterior and ventral lateral nuclei (VA/VL) of the thalamus and brain stem nuclei, such as the pedunculopontine tegmental nucleus (PPN) and superior colliculus. VA/VL thalamic neurons project to the frontal cortex. Ascending projections from the basal ganglia to the cerebral cortex through the thalamus control the learned movements of hands and the higher brain functions. On the other hand, descending projections to the motor centers in the brain stem control the innate motor functions, such as eye movements, trunk movements, locomotion, mastication, and vocalization.
Dopaminergic neurons in the SNc project to the striatum and modulate differentially the activity of striatal projection neurons in the direct and indirect pathways (Fig. 1.2) (Albin et al. 1989; Alexander and Crutcher 1990; DeLong 1990; Gerfen and Surmeier 2011). Dopamine excites the direct pathway striatal neurons through the dopamine D1 receptors, while it inhibits the indirect pathway striatal neurons through dopamine D2 receptors.
The cortico-basal ganglia loops are composed of the following several loops (Alexander et al. 1986; Albin et al. 1989; Alexander and Crutcher 1990; Middleton and Strick 2000). The motor loop controlling movements of forelimbs, hindlimbs, and trunk, starts from the motor cortices, such as the primary motor cortex (MI), supplementary motor area (SMA), and premotor cortex (PM), and goes through the somatomotor territories of the basal ganglia, and finally returns to the original cortices through the VA/VL of the thalamus. The somatomotor territories of the basal ganglia include the caudoventral putamen, the caudoventral GPe/GPi, and the dorsal STN, and each territory shows somatotopic organization. In addition to the motor loop, the oculomotor, prefrontal (composed of dorsolateral prefrontal and lateral orbitofrontal loops), and limbic loops connect the cerebral cortical areas (the frontal eye field/supplementary eye field, prefrontal cortex, and limbic cortex, respectively) with corresponding parts of the basal ganglia and thalamic nuclei, and form multiple, parallel, segregated, and functionally distinct but homologous loops (Fig. 1.2). Through these multiple loops, the basal ganglia control eye movements, higher brain functions and emotions, as well as limb movements.
The above basal ganglia circuitry may be oversimplified. There exist other significant projections, such as the following (Nambu 2008).
1.
It is supposed that striatal neurons projecting to the GPe and GPi belong to different groups. However, tracing studies of a single neuron have shown the existence of neurons projecting to both the GPe and GPi (Levesque and Parent 2005). It has also been reported that some striatal projection neurons express both D1 and D2 receptors. Moreover, electrophysiological experiments have failed to show the expected effect of dopamine on striatal projection neurons.
2.
Thalamic neurons, such as those in the VA/VL and centromedian-parafascicular complex (CM/Pf), project also to the striatum, suggesting a short circuit of striato-GPi-thalamo-striatal loop (McFarland and Haber 2000).
3.
The cortico-basal ganglia loops are considered to be composed of several segregated and parallel loops. However, there exists partial overlap of projections. Thus, these loops should be viewed more as a continuum rather than subdivisions with strict boundaries.
4.
The GPe gives rise to GABAergic projections to the GPi/SNr, striatum and GPe itself through local axon collaterals. Thus, the GPe may be viewed as a center nucleus projecting to multiple sites of the basal ganglia.
5.
The STN projects to the GPe, as well as to the GPi, using axon collaterals. The STN and GPe have intimate interconnections, and the interconnected groups of neurons in the GPe and STN innervate the same population of neurons in the GPi (Shink et al. 1996). Thus, the STN and GPe are coupled to each other and may work together.
6.
The basal ganglia and cerebellum are classically considered to function independently without interactions. However, recent anatomical studies suggest that the basal ganglia communicate with cerebellum through the cerebello-thalamo-striatal and STN-ponto-cerebellar pathways (Bostan et al. 2013).
1.4 Functions of the Basal Ganglia
Physiological functions of the basal ganglia are not yet fully understood; most probable hypothesis on the basal ganglia functions is the focused selection of intended movements, which may also explain pathophysiology of movement disorders of the basal ganglia origin.
Neurons in the output nuclei of the basal ganglia are inhibitory GABAergic and fire at high frequency (40–100 Hz), and thus, neurons in the target structures, such as the thalamus, are inhibited continuously (Fig. 1.3). When striatal neurons are activated by cortical inputs, the striatal neurons inhibit GPi/SNr activity through the striato-GPi/SNr direct pathway. The continuous inhibition from the output nuclei to the target structures is temporally removed (disinhibition), and neurons in the target structures, including thalamic and cortical neurons, are activated, resulting in the release of a selected motor program (Fig. 1.3) (Hikosaka et al. 2000). These mechanisms have been investigated in saccadic eye movements.
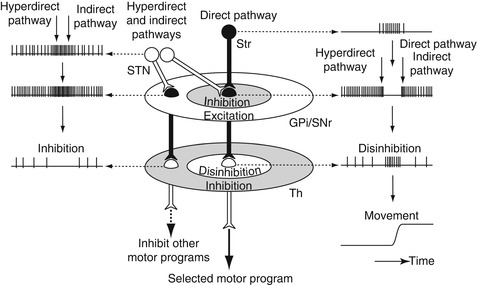
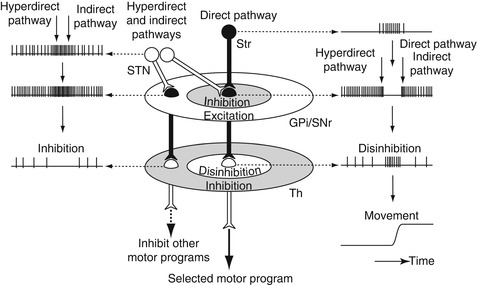
Fig. 1.3
Spatial and temporal distribution of basal ganglia activity during behavior. Signals from the motor cortices through the direct pathway inhibit GPi/SNr neurons, activate thalamic neurons by disinhibition, and finally release a selected motor program. On the other hand, signals through the hyperdirect and indirect pathways have broad excitatory effects on GPi/SNr neurons in temporal and spatial domains, make clear initiation and termination of the selected motor program, and inhibit other unnecessary competing motor programs (Modified from Nambu (2008) with permission)
On the other hand, signals through the hyperdirect and indirect pathways have excitatory effects on the GPi/SNr, and thus, have inhibitory effects on thalamic neurons. Considering the conduction time, signals through the hyperdirect pathway first actively inhibit thalamic neurons, then those through the direct pathway disinhibit them, and finally those through the indirect pathway inhibit them again. Thus, signals through the hyperdirect and indirect pathways make clear initiation and termination of the selected motor program (Fig. 1.3) (Nambu et al. 2002a; Nambu 2007).
In addition to such a temporal aspect, the enhancement by differential inputs through the hyperdirect, direct, and indirect pathways may work in spatial domain as well (Mink 1996). Anatomical studies have shown that STN-GPi fibers arborize more widely and terminate on more proximal neuronal elements than striato-GPi fibers. Signals through the hyperdirect and indirect pathways activate GPi/SNr neurons extensively, thereby inhibiting large areas of the thalamus, whereas signals through the direct pathway disinhibit thalamic neurons only in the center area (Fig. 1.3). Thus, signals through the hyperdirect and indirect pathways inhibit thalamic neurons in the surrounding area, which are related to other unnecessary competing motor programs (Mink 1996; Nambu et al. 2002a; Nambu 2007).
Based on the temporal and spatial inputs to the target structures through the hyperdirect, direct, and indirect pathways, only the selected motor program is executed at the selected timing, and other competing motor programs are canceled. In addition to the motor loop, the oculomotor, prefrontal, and limbic loops control the activity of corresponding cortical areas in a similar manner.
1.5 Firing Properties and Somatotopy of the Basal Ganglia
Neurons in each nucleus of the basal ganglia show characteristic firing rates and patterns reflecting their membrane properties, such as ion channels and receptors, and afferent inputs. Afferent and efferent connections of each nucleus of the basal ganglia maintain functional topography, and thus somatomotor territories of the basal ganglia show somatotopic organization. The following description on the firing properties and somatotopy of the basal ganglia and thalamus is derived mainly from animal experiments, especially using subhuman primates, and is also applicable to humans.
1.5.1 Striatum
The striatum is composed of major (80–95 %) projection neurons and minor interneurons (Alexander and DeLong 1985; Kawaguchi 1997; Wilson 2004). Projection neurons are inhibitory GABAergic, medium-sized neurons, and their dendrites are covered by numerous spines. Medium spiny projection neurons are usually silent and fire when they receive inputs, and thus, are described as phasically active neurons (PAN) in unit recording in vivo (Fig. 1.4a) (Vitek et al. 1997). Projection neurons can be divided into two groups, i.e., the direct and indirect pathway neurons, based on the transmitters and projection sites as described earlier, and both show similar firing properties. Interneurons lack spines and are classified into at least four groups: (1) cholinergic large aspiny neurons, (2) parvalbumin-containing GABAergic aspiny neurons, (3) somatostatin/nitric oxide synthase-containing GABAergic aspiny neurons, and (4) calretinin-containing GABAergic aspiny neurons. Interneurons, as well as projection neurons, receive inputs from the cerebral cortex, thalamus, and SNc, ultimately project to projection neurons and control their activity. Cholinergic large aspiny neurons fire spontaneously with broader spikes than PANs at 2–10 Hz and are described as tonically active neurons (TAN) in unit recording in vivo. The activity patterns of other interneuron in vivo remain to be elucidated.
