Abstract
This chapter discusses the pros and cons of rehabilitation gaming as an approach to upper extremity rehabilitation after a stroke. Gaming as a means of rehabilitation can expand access to rehabilitation services for rural dwellers or those with limited access to transportation. It can expand the scope of care, increase repetitions, and increase engagement. Virtual rehabilitation approaches appear favored over usual care for treating upper limb disability. When adopting virtual reality into rehabilitation programs, it is critical to combine in-game practice with techniques to promote carryover of training into daily living. Through analysis of continuous motion capture, gaming systems have the potential to advance the field by enabling personalized treatment recommendations.
Keywords
Disability, Gaming, Motor, Physical therapy, Physiotherapy, Rehabilitation, Stroke, Upper extremity, Virtual reality, VR
Outline
Rehabilitation Gaming Can Address Major Care Disparities 465
The Pros and Cons of Available Gaming Technologies 466
Theoretical Differences That Favor Gaming Rehabilitation 467
Theoretical Differences That Favor Conventional Therapy 468
Comparative Effectiveness of Rehabilitation Gaming Systems 469
Future Directions 471
References 473
Rehabilitation Gaming Can Address Major Care Disparities
Upper extremity motor disability following damage to the brain makes everyday tasks such as dressing and preparing meals extremely challenging and time-consuming to accomplish. These difficulties exert notable effects on a person’s quality of life ( ). Upper extremity disability is also hugely costly to society. There are an estimated 2.4 million chronic stroke survivors in the United States ( ), of whom approximately 50% have difficulty moving an upper extremity and 26% require assistance for activities of daily living ( ). Care for stroke survivors and lost productivity cost an estimated $34 billion annually in the United States ( ). In the United Kingdom, poststroke care comprises 5% of total healthcare costs ( Fig. 33.1 ).

Poststroke disability is made worse by inadequate access to long-term rehabilitation care ( ). Clinical practice guidelines recommend outpatient rehabilitation for anyone who remains disabled after discharge from inpatient rehabilitation ( ). Although these guidelines would recommend that the majority of stroke survivors receive at least some outpatient rehabilitation ( ), substantially fewer stroke survivors actually receive this care ( ). Inadequate rehabilitation results in higher disability as well as increased mortality ( ).
Among those individuals who do undergo outpatient rehabilitation, most do not receive evidence-based treatments. found in an observational study of 312 rehabilitation sessions involving 83 occupational and physical therapists (OT/PTs) at seven rehabilitation sites across the United States that functional rehabilitation (i.e., movement that accomplishes a functional task, such as eating, as opposed to strength training or passive movement) was provided in only 51% of the sessions of upper extremity rehabilitation, with only 45 repetitions per session on average. Functional upper extremity movements are most likely to generalize to everyday tasks ( ), an aspect of recovery that is critically important to patients and their families ( ). Yet, passive movement and non-goal-directed exercise are more frequently administered ( ). Meta-analytic data suggests that the intensity of motor intervention, as measured by hours of treatment, is associated with the magnitude of the treatment effect ( ), yet the Centers for Medicare & Medicaid Services (CMS) therapy cap limits the number of sessions of occupational and physical therapy combined to about 20 per year.
The standard of care for upper extremity rehabilitation is thus characterized by inadequate provision of rehabilitation services, particularly for rural and underserved populations ( ), as well as poor utilization of interventions that are sufficiently intense. Moreover, traditional home practice programs are not sufficiently engaging to result in adequate adherence ( ), making traditional home practice programs an infeasible alternative for most people. The end result is that motor function often remains generally unchanged after discharge from inpatient rehabilitation and actually declines in underserved populations ( ). Innovative and cost-cutting rehabilitation approaches are thus sorely needed to reform the rehabilitation care delivery system.
Enter rehabilitation gaming. The use of “smart” gaming rehabilitation systems has the potential to increase access to rehabilitation, particularly for those residing far from rehabilitation centers or with limited access to transportation. By reducing task setup and intensely engaging the client, a greater portion of session time can be spent in active motor practice, potentially increasing the efficiency of rehabilitation sessions. Finally, widespread adoption of rehabilitation gaming could markedly reduce the costs associated with rehabilitation by enabling the majority of rehabilitation to be self-led between consultations with a therapist. Rehabilitation gaming thus has the potential to address a huge social need in the field of rehabilitation.
The Pros and Cons of Available Gaming Technologies
Three main s of technologies have been used in rehabilitation gaming systems: controller-based systems, camera-based motion-tracking systems, and wearable sensor systems. The oldest consumer-grade systems and those most commonly studied to date are accelerometer-based controller systems, such as the Nintendo Wii. This type of system has the advantage of being the lowest-cost system but has substantial drawbacks, including inability to track movements made by the player, requiring a user to be able to grasp a hand-held controller, and lack of sensitivity to movement patterns.
Camera-based motion-tracking systems, such as the Microsoft Kinect, are becoming the preferred platform for rehabilitation gaming. These systems integrate information from a traditional video camera and an infrared depth sensor to infer the approximate location in space of up to 25 parts of the body ( Fig. 33.2 ). As such, these systems offer several advantages over accelerometer-based systems, yet they remain relatively inexpensive. Gaming software can be written to require specific movement patterns to drive game play, thereby discouraging players from using atypical or compensatory movement patterns, such as disallowing the player to compensate for decreased shoulder abduction by leaning to the side. Because these systems track actual body movement, the rehabilitation principle of action–observation can be harnessed when a figure on the screen mirrors the player’s movements ( ). Personalization of rehabilitation can also be realized when the software automatically adjusts the difficulty of the movements required to drive game play based on a user’s current ability. This capacity allows the gaming system to respond to a player in the same way that a live therapist would, progressing the difficulty of a task as a client improves. Finally, fine motor skills and active grasp are not required because there are no buttons to push and no controller to hold, making these systems more widely accessible to individuals with a wide range of motor ability.

Very recently, advances in wearable motion capture technology have reduced the cost of such sensors, making them more accessible to the rehabilitation gaming market. These wearables can very precisely track the position and orientation of the sensors in space. Several sensors applied to the body allow for reconstruction of body movements, similar to that provided by the Microsoft Kinect. These sensor systems have the added advantages over the Kinect of portability and increased temporal precision but are more cumbersome to set up. They are also not yet available as consumer-grade systems and have not incorporated user-centric design, making them more challenging to implement for clinical and home-based gaming applications.
There is strong rationale for the use of rehabilitation gaming. However, it is unclear to what extent existing systems will live up to their potential to improve motor outcomes following neurologic injury. Rehabilitation gaming systems offer the following potential advances but also have potential drawbacks compared with conventional rehabilitation.
Theoretical Differences That Favor Gaming Rehabilitation
Random , rapid alternation in task demands and complexity: A meta-analysis of existing motor therapies for stroke has found that task-variability (greater variability of exercises that train each class of motor movements, with random ordering of exercises) optimizes retention of therapy gains, particularly when the tasks involve meaningful movements toward a clear functional goal (behavioral-relevance) ( ). Gaming therapy inherently harnesses the concurrent benefits of task variability and behaviorally relevant training ( ) by requiring a person to switch rapidly between motor movements to successfully navigate the virtual world.
More repetitions per time: advocated for using computer-assisted rehabilitation gaming to optimize motor recovery by motivating and engendering the full participation of the client. Those engaging in rehabilitation gaming often feel that they achieved more repetitions in a shorter time because they felt motivated by in-game “rewards.” Game-based rehabilitation also eliminates “down time” when switching between different motor tasks. Rehabilitation gaming systems can accommodate more than five times as many repetitions per hour than traditional rehabilitation ( ). The evidence is equivocal regarding whether more repetitions of movement performed during a training session enhances outcomes ( ), but it is intuitive to expect that some degree of repetition is necessary for motor improvement.
Combining “top-down” and “bottom-up” motor learning: Given that the brain tissue damage incurred through stroke is permanent, it is clear that the capacity of the brain to “rewire itself” is critical to the long-term success of rehabilitation. Rehabilitation-induced brain reorganization is driven by two complementary processes: “bottom-up” (increased use) and “top-down” (cognitive) motor learning. Therapies incorporating “bottom up” motor learning typically use intensive practice, whereas therapies incorporating “top-down” motor learning rely more heavily on focused attention and concentration to movement. Examples of “top-down” motor training include mirror training (patients “witness” normal movement of their impaired limb by moving their less-affected extremity in front of a midline mirror) ( ), action observation ( ), and mental practice ( ). Motor interventions that incorporate many hours of active motor practice promote “bottom-up” changes in brain structure and function through emphasis on intensive practice of behaviorally relevant movement. These interventions can greatly alter the size of the regional brain activity or activation pattern associated with the more affected arm ( ) and can increase gray matter volume in sensorimotor areas and hippocampus ( ). However, there is some evidence to indicate that “bottom-up” training may be enhanced (e.g., improved motor memory ( ) and force of movement ( )) by additionally incorporating “top-down” training ( ). Not surprisingly, the three aforementioned “top-down” interventions promote increased cortical excitability ( ) and functional activity ( ) in motor and motion-processing areas, as well as in a distributed network of brain areas not traditionally associated with motor function. In sum, the literature suggests that optimized brain recovery may involve both “bottom-up” and “top-down” therapies. The combination of “bottom-up” and “top-down” therapy is accomplished concurrently through rehabilitation games that incorporate avatars (game characters that mirror movements of the player). An avatar allows users to observe their own movements (“top-down” processing) as they are performing them (“bottom-up” processing). It is possible that the use of avatar-based feedback can activate similar neural circuits as other “top-down” motor therapies that aid recovery of function ( ).
Implicit learning: Implicit knowledge refers to procedural or “intuitive knowledge that one seems to have little conscious access to” ( ) (e.g., knowledge of how to ride a bike). This is in contrast to explicit/declarative knowledge that can be verbally articulated. Although stroke can impair explicit learning and recall, implicit learning is preserved ( ). Learning strategies designed to invoke implicit processes are therefore particularly effective at enhancing motor skill recovery among individuals living with poststroke deficits ( ), whereas the explicit instruction often incorporated in traditional therapy approaches can actually interfere with motor learning ( ). By nature of the trial-and-error learning environment (e.g., lack of verbal instruction), games provide patient-directed and motivating implicit learning paradigms.
Multi-modal feedback: Implicit feedback provided concurrently to more than one sensory pathway (e.g., visual and auditory) has been shown to reinforce learning and positively impact rehabilitation outcomes ( ). This type of feedback is highly stimulating and can be particularly helpful in motivating individuals who may have reduced attentional capacity secondary to stroke.
Increased engagement: Behavioral psychologists have long recognized the importance of reinforcement (i.e., “rewards”) in motivating desired behaviors. Enhancing motivation is critical in rehabilitation because the physically demanding tasks require extensive effort and yield frustration when movement attempts are unsuccessful ( ). Some patients shy away from intensive therapies due to these concerns ( ). This poses a significant problem for rehabilitation because training load needs to be sufficiently intense ( ). While the efficacy of “rewards” in stimulating interest in a task is well recognized by the video game industry, these behavioral reinforcers are often largely omitted from traditional rehabilitation paradigms. Bach-y-Rita et al. thus advocated for the use of computer-assisted rehabilitation gaming to optimize motor recovery by motivating and engendering the full participation of the patient ( ). The scheduled delivery of rewards in a gaming environment can use the principles of intermittent reinforcement ( ) and occasional “big wins” to motivate maximal performance and keep clients engaged in rehabilitation. “Rewards” during virtual reality motor training have the additional benefit of serving as real-time implicit performance feedback . For instance, a patient is rewarded only when performing the desired motor movements. Implicit performance feedback has been shown to increase motor skill reacquisition after stroke ( ).
Theoretical Differences That Favor Gaming Rehabilitation
Random , rapid alternation in task demands and complexity: A meta-analysis of existing motor therapies for stroke has found that task-variability (greater variability of exercises that train each class of motor movements, with random ordering of exercises) optimizes retention of therapy gains, particularly when the tasks involve meaningful movements toward a clear functional goal (behavioral-relevance) ( ). Gaming therapy inherently harnesses the concurrent benefits of task variability and behaviorally relevant training ( ) by requiring a person to switch rapidly between motor movements to successfully navigate the virtual world.
More repetitions per time: advocated for using computer-assisted rehabilitation gaming to optimize motor recovery by motivating and engendering the full participation of the client. Those engaging in rehabilitation gaming often feel that they achieved more repetitions in a shorter time because they felt motivated by in-game “rewards.” Game-based rehabilitation also eliminates “down time” when switching between different motor tasks. Rehabilitation gaming systems can accommodate more than five times as many repetitions per hour than traditional rehabilitation ( ). The evidence is equivocal regarding whether more repetitions of movement performed during a training session enhances outcomes ( ), but it is intuitive to expect that some degree of repetition is necessary for motor improvement.
Combining “top-down” and “bottom-up” motor learning: Given that the brain tissue damage incurred through stroke is permanent, it is clear that the capacity of the brain to “rewire itself” is critical to the long-term success of rehabilitation. Rehabilitation-induced brain reorganization is driven by two complementary processes: “bottom-up” (increased use) and “top-down” (cognitive) motor learning. Therapies incorporating “bottom up” motor learning typically use intensive practice, whereas therapies incorporating “top-down” motor learning rely more heavily on focused attention and concentration to movement. Examples of “top-down” motor training include mirror training (patients “witness” normal movement of their impaired limb by moving their less-affected extremity in front of a midline mirror) ( ), action observation ( ), and mental practice ( ). Motor interventions that incorporate many hours of active motor practice promote “bottom-up” changes in brain structure and function through emphasis on intensive practice of behaviorally relevant movement. These interventions can greatly alter the size of the regional brain activity or activation pattern associated with the more affected arm ( ) and can increase gray matter volume in sensorimotor areas and hippocampus ( ). However, there is some evidence to indicate that “bottom-up” training may be enhanced (e.g., improved motor memory ( ) and force of movement ( )) by additionally incorporating “top-down” training ( ). Not surprisingly, the three aforementioned “top-down” interventions promote increased cortical excitability ( ) and functional activity ( ) in motor and motion-processing areas, as well as in a distributed network of brain areas not traditionally associated with motor function. In sum, the literature suggests that optimized brain recovery may involve both “bottom-up” and “top-down” therapies. The combination of “bottom-up” and “top-down” therapy is accomplished concurrently through rehabilitation games that incorporate avatars (game characters that mirror movements of the player). An avatar allows users to observe their own movements (“top-down” processing) as they are performing them (“bottom-up” processing). It is possible that the use of avatar-based feedback can activate similar neural circuits as other “top-down” motor therapies that aid recovery of function ( ).
Implicit learning: Implicit knowledge refers to procedural or “intuitive knowledge that one seems to have little conscious access to” ( ) (e.g., knowledge of how to ride a bike). This is in contrast to explicit/declarative knowledge that can be verbally articulated. Although stroke can impair explicit learning and recall, implicit learning is preserved ( ). Learning strategies designed to invoke implicit processes are therefore particularly effective at enhancing motor skill recovery among individuals living with poststroke deficits ( ), whereas the explicit instruction often incorporated in traditional therapy approaches can actually interfere with motor learning ( ). By nature of the trial-and-error learning environment (e.g., lack of verbal instruction), games provide patient-directed and motivating implicit learning paradigms.
Multi-modal feedback: Implicit feedback provided concurrently to more than one sensory pathway (e.g., visual and auditory) has been shown to reinforce learning and positively impact rehabilitation outcomes ( ). This type of feedback is highly stimulating and can be particularly helpful in motivating individuals who may have reduced attentional capacity secondary to stroke.
Increased engagement: Behavioral psychologists have long recognized the importance of reinforcement (i.e., “rewards”) in motivating desired behaviors. Enhancing motivation is critical in rehabilitation because the physically demanding tasks require extensive effort and yield frustration when movement attempts are unsuccessful ( ). Some patients shy away from intensive therapies due to these concerns ( ). This poses a significant problem for rehabilitation because training load needs to be sufficiently intense ( ). While the efficacy of “rewards” in stimulating interest in a task is well recognized by the video game industry, these behavioral reinforcers are often largely omitted from traditional rehabilitation paradigms. Bach-y-Rita et al. thus advocated for the use of computer-assisted rehabilitation gaming to optimize motor recovery by motivating and engendering the full participation of the patient ( ). The scheduled delivery of rewards in a gaming environment can use the principles of intermittent reinforcement ( ) and occasional “big wins” to motivate maximal performance and keep clients engaged in rehabilitation. “Rewards” during virtual reality motor training have the additional benefit of serving as real-time implicit performance feedback . For instance, a patient is rewarded only when performing the desired motor movements. Implicit performance feedback has been shown to increase motor skill reacquisition after stroke ( ).
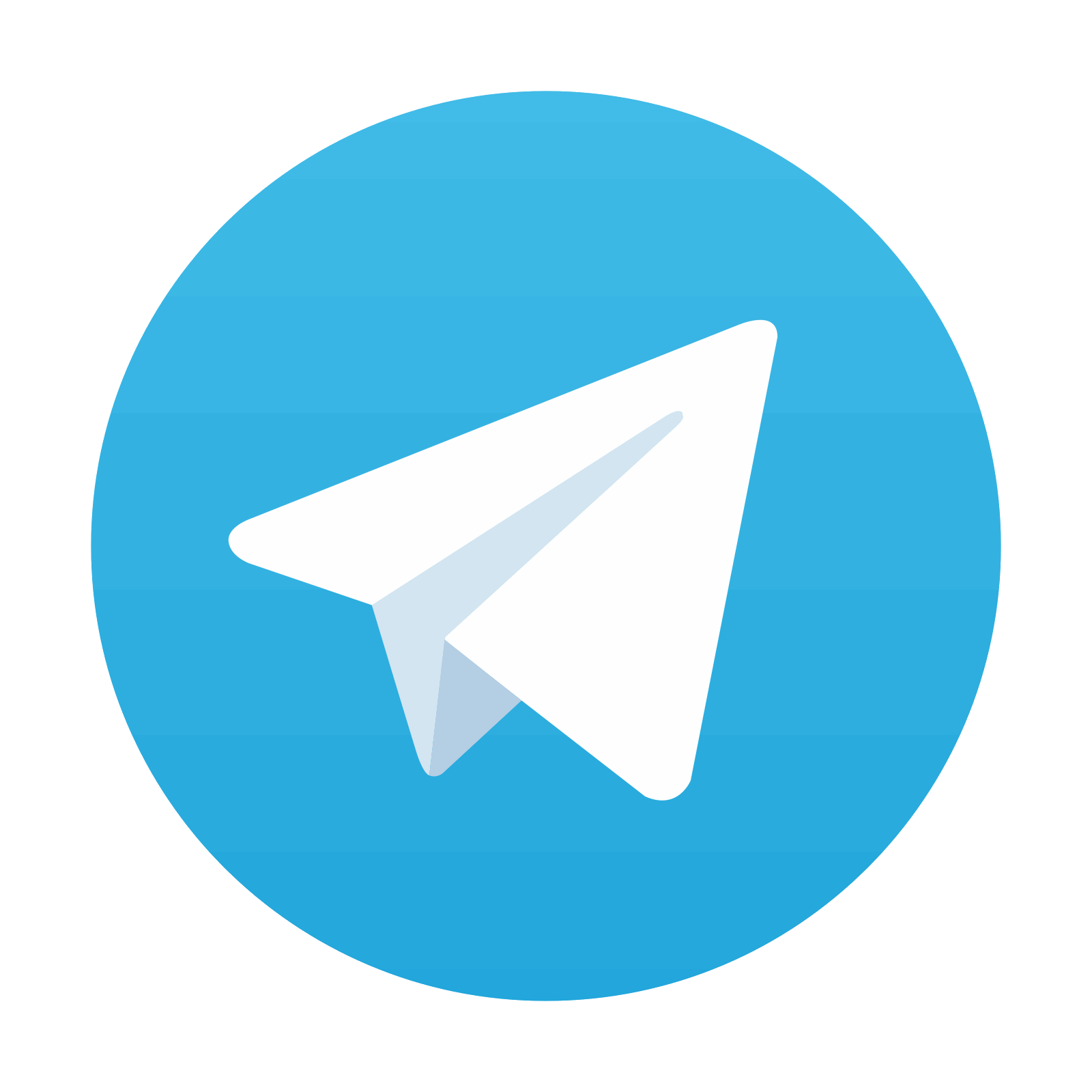
Stay updated, free articles. Join our Telegram channel

Full access? Get Clinical Tree
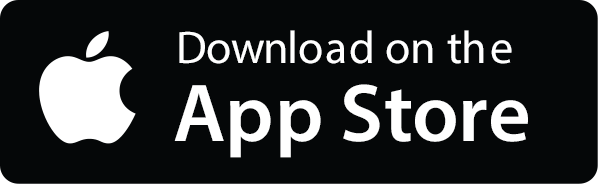
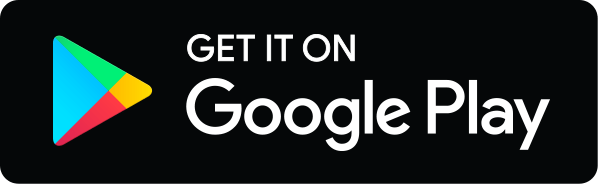