Alzheimer’s disease in adults with Down syndrome
As described in Chapter 1, Down syndrome (DS) is one of the most common genetic diseases. Adults with DS carry three copies of chromosome 21, and triplication and overexpression of the amyloid precursor protein (APP) gene leads to excess amyloid-β (Aβ) peptides. This eventually leads to the neuropathological manifestations of Alzheimer’s disease (AD) in DS at younger ages than in the general population (see Chapter 2). Until the 1960s, few individuals with DS lived beyond 60 years of age [1, 2]. In the past several decades, life expectancy has increased dramatically such that children born with DS today are expected to live into their 60s and often beyond [2]. Consequently, most adults with DS will have developed AD-associated neuropathology by 40 years of age, with 60% of them developing dementia by 60 years of age [3–5].
As the prevalence of AD increases in adults with DS, AD is becoming a public health problem in adults with DS as it is in the general population. The two classes of widely used medications—acetylcholinesterase inhibitors and NMDA receptor antagonists—that are used to delay or prevent the progression of AD have little success. These treatments may slow down symptoms such as memory loss; however, they do not stop the underlying disease progression toward AD. Moreover, the elevated prevalence of multiple comorbidities and polypharmacy in adults with DS may further complicate the treatment of AD in these adults [6, 7] (see Chapter 11). Given the limited success of these medications, it would be important to identify novel biomarkers and pathways that can predict the development of AD in individuals and alter the risk of AD to identify additional therapeutic targets (see Chapter 16). One way to achieve this is to examine which factors, genetic or otherwise, protect against AD among individuals with a high risk of developing AD. Novel genes may provide molecular insight into the mechanisms toward AD, which may guide us to the development of effective intervention before irreversible neurodegeneration occurs or may ameliorate the symptoms and slow down the neurodegenerative processes toward AD.
Advantages of studying the genetics of Alzheimer’s disease in Down syndrome
Gene dosage. Genetic studies of AD in adults with DS can examine the role of extra allelic dosage of the genes on chromosome 21 along with nontrisomic genes outside chromosome 21, which influence AD-related phenotypes. To date, most genetic studies of adults with DS have had small sample sizes and thus have focused on candidate genes that have been identified in the general population to overcome limited statistical power to detect risk variants without strong effects. Most published candidate gene studies have examined the association between common genetic variants and multiple AD-related phenotypes, including AD risk, age at onset of AD, levels of amyloid β (Aβ) peptides in plasma, and now imaging data. Most candidate genes identified in adults with DS tend to overlap with those identified in the general population as evident in publications on APOE [8, 9], IDE [10, 11], PICALM [12].
Gene-gene interaction. Genetic studies of DS allow us to examine how other genes on chromosome 21, such as BACE2 and Sβ100, contribute to AD-related phenotypes [13]. Since genes on chromosome 21 other than APP are also trisomic and may also be overexpressed, this presents a unique opportunity to examine gene-gene interaction where both genes are trisomic. Genetic variants in DS studies show stronger risk ratios than those in the general population, and this attribute allows DS studies to gain statistical power to detect gene-gene interaction, especially when most genetic variants tend to have weak to modest effects in the general population. Even for genes outside chromosome 21, excess levels of Aβ peptides may allow detection of genetic modifiers with weak to modest effects.
Partial trisomies. Partial trisomy 21 cases, where only a segment of chromosome 21 is present in three copies, are extremely rare, but are biologically insightful. For example, reported partial trisomy 21 that was disomic at the APP gene and the flanking region, but trisomic in the remaining chromosome 21 regions failed to present AD-associated phenotypes, even though they had many DS-associated phenotypes, such as intellectual disability, ophthalmologic problems, distinctive facial features, etc. [14, 15] Although the numbers of known partial trisomies are small, these rare special cases can shed light on how extra dosage or lack of extra dosage of genes on chromosome 21 contribute to variable phenotypic expressions [16], which may be examined at molecular levels by examining multiple layers of intermediate endophenotypes.
Phenotype variation. Even among individuals with full trisomy, there is wide variation in neurocognitive performance and age at onset of AD. For example, Krinsky-McHale and colleagues [17] described a male adult with DS who survived to 70 years of age with full trisomy 21 whose longitudinal assessments of his cognitive performance showed no significant decline suggestive of dementia. Such unusual clinical cases are ideal persons to examine potential protective genetic factors for AD risk. It is also interesting to note that up to 15% of people with DS do not appear to develop dementia well into older ages, which also emphasizes the need to examine genetics in the context of this phenotypic variation [18].
Changes in endophenotypes over time. It is possible to enhance our understanding of the causal relations between genetic factors and risk for AD by measuring multiple endophenotypes at the cellular level, to better understand the pathways from genes to proteins and to metabolites, reflecting the central dogma in molecular biology. When these endophenotype biomarkers are measured longitudinally, long before the signs and symptoms of dementia appear, it may be possible to gain biological insight into how a given gene may have contributed to each layer of endophenotype and eventually influence clinical endpoints, including AD status [2]. These layers of endophenotype can range from blood-based biomarkers (e.g., levels of Aβ-42, tau, neurofilament light chain—see Chapter 6 and 7), to cerebrospinal fluids, to imaging markers representing structural and functional alterations in images from MRI and PET (see Chapters 8 and 9). In addition to conventional biomarkers, a large set of proteins and their interactions can now be studied through proteomics, and a similarly large set of small molecules and their interactions can be studied through metabolomics. Together, these biomarker data can now be used to further refine signature biomarkers to predict AD risk. Until recently, these techniques were unavailable for large-scale studies because the costs would have been prohibitive. Fortunately, the costs have been lowered substantially such that studies are beginning to incorporate these state-of-the-art techniques to better understand how genetic variants may contribute to the neurodegenerative processes of dementia mechanistically, allowing us to evaluate functional relevance, beyond statistical association, of the identified genes and variants within.
Other high-risk populations: Autosomal dominant Alzheimer’s disease (ADAD)
Studies of Autosomal Dominant Alzheimer’s Disease (ADAD) forms, along with those of DS, representing high risk populations, can allow biological insight into the AT(N) (Amyloid β, Tau, Neurodegeneration) system, which provides a widely accepted sequence of events leading to AD [19]. However, it is important to note that at every point along the clinical disease stage, levels of biomarkers can vary widely, which may affect clinical (sub-)phenotypes. Modifier genes may explain some of the observed phenotypic variations. While this is possible to detect in any population, it is much more likely to be detected in these high-risk cohorts because their effect sizes are likely to be greater in magnitude than in the general population. The three genes that have been implicated for ADAD are the amyloid precursor protein (APP) gene on 21q21.3, presenilin 1 (PSEN1) on 14q24.2, and presenilin 2 (PSEN2) on 1q42.13. PSEN1 and PSEN2 encode for the presenilin 1 and presenilin 2 proteins, as part of the γ-secretase complex, and are responsible for cleaving APP into Aβ peptides. As with individuals with DS, those who carry mutations in ADAD suffer from the burden of high levels of Aβ peptides, and tend to have early onset of cognitive decline and high prevalence of AD.
These mutation carriers have inspired multiple observational and therapeutic studies since they are likely to experience early onset cognitive decline and are likely to develop AD. These carriers allow identification of protective as well as risk genetic variants that are difficult in the general population without large-scale studies. As in adults with DS, these studies of mutation carriers have reported a wide range of phenotypic variation. When some people who are carriers develop AD at a young age, they can be examined to see the consequence of high levels of Aβ peptides and for therapeutic interventions. On the other hand, when carriers develop AD in their 70s-80s or “escape” AD, they can potentially provide valuable information on protective genetic factors. The largest international study with both observational and therapeutic components is the Dominantly Inherited Alzheimer’s disease (DIAN) study [20]. In addition to DIAN, two other cohorts that carry founder mutations in the PSEN1 gene are being studied to our knowledge: the PSEN1-G206A mutation in Puerto Rico [21, 22] and the PSEN1-E280A mutation in Colombia [23, 24]. Lee and colleagues [21] have identified genes on 4q35 and 2q13, namely SH3RF3, SNX25, SORBS2, that were associated with a delay in age at onset by 10 or more years in mutation carriers, and then replicated the findings in a cohort with late onset familial AD and in the general population. Lopera and colleagues identified APOE Christian Church mutation that protects against AD [25]. These modifier genes and their functional relevance identified in ADAD need to be examined in adults with DS. These modifier genes may shed light on the relations among levels of Aβ peptides, Tau, and the novel modifier.
Genes on chromosome 21
APP on 21q21.3. A surprisingly small number of studies have reported any allelic association with variants in the amyloid precursor protein (APP) gene in cohorts with DS or in the general population. One population-based genomic study examined a cohort of Icelandics without DS (n = 1795) and reported a rare coding variant in the A673T mutation in the APP gene (rs63750847), where the A allele converts alanine to threonine (A673T) [26]. Elderly carriers of the A673T mutation in APP had a lower likelihood of memory decline and a reduced risk of AD. The A673T mutation was associated with a ~ 40% reduction in the formation of Aβ peptides in vitro, supporting the hypothesis that reducing the β-cleavage of APP may protect against AD. Furthermore, the A673T allele was protective against cognitive decline in nondemented elderly, suggesting that both cognitive decline with normal aging and with AD may share the same or similar pathway. This variant is quite rare in Icelandics (allele frequency of 0.62%) and in other Scandinavian populations (ranging from 0.21% to 0.51%). Globally, as estimated by the Genome Aggregation Database (gnomAD), the allele frequency is 10-fold lower at 0.0446% in the general European population.
A subsequent genome-wide association study by Jones and colleagues [12] did not find any common variants in APP. However, Lee and colleagues [11] did report several different common variants in the APP gene that were associated with an elevated risk of AD in adults with DS, with risk ratios ranging from 1.43 to 2.04. Given these risk ratios, these common variants may have weak to modest effects on biomarkers or clinical phenotypes, or they may be physically located near (or are in linkage disequilibrium with) variants with stronger effect size.
Korenberg and colleagues [16, 27] pioneered ways to genetically dissect genotype-phenotype relations in adults with DS by carefully examining partial trisomies. These rare individuals have a part of their chromosome 21 that is trisomic at birth [28, 29] unlike low level acquired mosaicisms (12%–25% of the cells) that occur in older adults with DS as they age [30–32]. Partial trisomies allow comparison of three groups: individuals with full trisomy vs. individuals with partial trisomy (who may be trisomic or disomic at a given locus) vs. individuals without DS. For example, Prasher and colleagues [14] and Doran and colleagues [15] reported that some adults with DS successfully survive to old age without observable cognitive decline as a result of having a deletion in the chromosomal region containing the APP gene. In their paper, Prasher and colleagues [14] showed that one woman with DS with partial trisomy karyotype [46,XX,rec(21)dup q, inv(21)(p12q22.1)], who had two copies of the APP gene, had no phenotypic features of AD. In 2017 Doran and colleagues [15] reported that an adult with DS who was disomic at the APP gene [47,XY,+del(21)(q11.2q22.1)[18]/46,XY[2]] did not show AD-related symptoms and had substantially lower levels of Aβ-42 and Aβ-40 peptides. These studies confirm that the APP gene is crucial in risk for AD and additional modifier genes are likely to contribute to phenotypic variation. Studies of partial trisomies involving other genes on chromosome 21 can shed light on the biology of AD in adults with DS.
DYRK1A on 21q22.13. Dual-specificity tyrosine-(Y)-phosphorylation-regulated kinase 1A (DYRK1A) encodes a protein that plays a role in neurodevelopment of both physiological and pathological conditions in both individuals with and without DS. Numerous animal studies have reported that DYRK1A protein may contribute to brain defects, influencing cognitive ability [33–35]. Moreover, an inhibitor of DYRK1A along with cognitive training—given to young adults with DS (ages range: 16–34 years)—led to improvements in their visual recognition memory, working memory performance, and adaptive behavior, suggesting that the influence of DYRK1A at an early developmental stage may influence their memory abilities [36]. However, human genetic studies on DYRK1A are limited. To date, a limited number of candidate gene studies [37–41] and one genome-wide study by Jones and colleagues [12] have examined SNPs on DYRK1A. The study by Kimura and colleagues [42] was the only study that showed a genetic association between a genetic marker, rs2835740, and an increased risk of AD in a Japanese cohort with DS, and also showed a 7-fold expression of mRNA in the hippocampus of persons with AD without DS. Further studies are needed to clarify the role of DYRK1A.
SYNJ1 on 21q22.11. Miranda and colleagues [43] integrated human studies and transgenic mouse models to show that synaptojanin 1 levels regulate the function of cells in the aging hippocampus. In postmortem brain samples from the frontal cortex of individuals with DS, the relation between levels of synaptophysin, an indicator of synaptic integrity, was negatively correlated with levels of SYNJ1, a key regulator of synaptic vesicle endocytosis, suggesting that variants in SYNJ1 may affect changes in synapse and modify the risk of AD. Furthermore, when the PSEN1 mutation carriers were examined, some SNPs in SYNJ1 were associated with earlier age at onset or variable performance in memory tests, suggesting that genetic factors are likely to influence the neurodegenerative process in this high-risk group. Moreover, compared with wild type mice, aging Tg(Synj1) mice overexpressing Synj1 exhibited significant cognitive decline. Taken together, SYNJ1 may influence the aging hippocampus to accelerate the age at onset of AD or increase the risk of AD by affecting synaptic function in neurons.
BACE2 on 21q22.2-q22.3. BACE2 (Beta-Secretase 2) is associated with risk for late onset AD in the general population and is hypothesized to be involved in the amyloid cascade pathway. BACE2 is a homologue of BACE1, which is involved with cleavage of APP into amyloid β, and it was shown that BACE2 contributes to Aβ production in carriers of the Flemish missense mutation in APP [44]. Using a genome-wide association approach, Mok and colleagues [45–47] showed that rs2252576, a SNP, along with three other nearby SNPs exhibited a significant association with age at onset of AD in 67 adults with DS. Similarly, Schupf and colleagues [10] examined adults with DS and observed an allelic association between a SNP and the ratio of Aβ42/40 in plasma (empirical p = 0.0056). This SNP was located 7kb away from the SNP reported by Mok and colleagues mentioned before. Similarly, non-DS cohorts from Finland and from the US [48] also reported that late onset AD risk and levels of Aβ42 in cerebrospinal fluid were associated with SNPs that were 7–9 kb away from that reported by Mok et al. [49]. Further studies are needed.
Genes outside chromosome 21 with a reported association with dementia in adults with DS
APOE on 19q13.32. Multiple studies in adults with DS [9, 50–57] and in the general population show that APOE ɛ4 is associated with an earlier age at onset or an elevated risk of AD; therefore, the APOE ɛ4 isoform is the strongest genetic common variant known to date. In the general population, the risk of AD in APOE ɛ4 carriers is approximately threefold higher than those without (~ 40% vs. 14%) [58]. Moreover, heterozygous APOE ɛ4 carriers had an age at onset of AD that was eight years earlier, and homozygous carriers 16 years earlier [58–60]. ln adults with DS, the risk associated with APOE ɛ4 was four- to sevenfold higher than in those without [53, 61], risk ratios that are substantially higher in adults with DS than in the general population. It is of interest to note that APOE ɛ2 protects against AD [54, 62], in both adults with DS and in the general population. The current understanding is that APOE protein is an important cholesterol carrier, involved in lipid transport and injury repair [58]. APOE binds to Aβ and facilitates its removal from the brain; the APOE ɛ4 isoform is the least efficient compared with the other isoforms and is associated with a higher amyloid burden [60]. APOE ɛ4’s role in AD development highlights the delicate equilibrium between production and clearance of Aβ in the maintenance of homeostasis.
CR1 on 1q32.2. Complement C3b/C4b Receptor 1 gene encodes the CR1 protein which plays a critical role in the capture and clearance of pathogens as part of immune response [63]. Patel, Prasher, and colleagues [64] examined the genotypic association in their cohort of 268 individuals with DS (109 AD vs. 159 no AD). The CR1 variant (rs3818361) was associated with an elevated risk of death (Hazard Ratio (HR) = 1.65), but not an elevated risk of dementia. It is possible that variants associated with increased mortality, but not with risk of AD may be associated with accelerated aging, but less so with neurodegenerative processes. They then computed the genetic risk score (GRS) using all nine SNPs from nine candidate genes identified from large-scale GWAS studies to estimate an overall genetic risk for AD, but failed to show a significant association between the GRS and AD. The authors recognized that their study lacked statistical power; therefore, negative findings should be interpreted with caution.
PICALM on 11q14.2. The PICALM gene encodes cytoplasmic adapter protein that is predominantly expressed in neurons [63], and plays a critical role in clathrin-mediated endocytosis which is important in processes such as internalization of cell receptors, synaptic transmission, or removal of apoptotic cells. In addition to binding to clathrin, PICALM mediates the endocytosis of small R-SNARES (Soluble NSF Attachment Protein REceptors) between plasma membranes and endosomes [65–67], and this PICALM-dependent SNARE endocytosis is needed for the formation and maturation of autophagic precursors [68], suggesting its potential involvement in autophagy.
In adults with DS, two studies found weak associations between SNPs in PICALM and age at onset of AD or AD risk [12, 64]. Based on 120 study participants (70 with dementia), Jones and colleagues observed a weak association in carriers of three SNPs in PICALM with an earlier age at onset or increased risk of AD, when compared with carriers of reference SNPs. Patel and colleagues [64], using a relatively small sample of adults with DS (n = 109 demented out of 295 participants), tested one protective SNP (rs561655) identified from a large-scale genomic study in the general population by Naj and colleagues [69]. They observed a modest protective effect on AD risk and mortality. A subsequent study by Kunkle et al. [70, 71] reported a different protective SNP (rs3851179) than that by Naj et al. We note that the three SNPs reported in the three studies are located within 60kb of each other, and this segment of chromosome that includes the haplotype may be worth investigating further using whole genome sequencing data to pinpoint contributing genetic risk factor(s).
TREM2 on 6p21.1. Triggering receptor expressed on myeloid cells 2 (TREM2) is part of the innate immune system expressed on microglia, macrophages, and dendritic cells. The R47H variant, coding variants of TREM2, was associated with a threefold increase in late onset AD and also with frontotemporal dementia (FTD) [72, 73]. Network analysis of gene expression has identified TREM2 as a hub that connects to other genes in multiple pathways implicated in AD. Weber and colleagues reported the relationship between a soluble product of TREM2, sTREM2, and inflammatory markers in young adults with DS without any dementia symptoms [74]. Using immune response markers, they assessed preclinical staging of dementia in adults with DS long before dementia-related symptoms appear. Even though this pilot study had a very small number of affected participants (n = 15) and a small nondemented comparison group (n = 16), the levels of plasma sTREM2 and other inflammatory markers were significantly elevated in cases only. In addition, Raha-Chowdhury and colleagues [75] showed that the TREM2 protein is essential for neuroplasticity and myelination, where having low levels of TREM2 protein can lead to accelerate aging and increased neuronal cell loss, leading to neuroinflammation. When extended to the general population, studies show that a soluble cleavage product of TREM2 is elevated in AD cerebrospinal fluid and is positively correlated with cognitive decline [74, 76, 77].
SORL1. SORL1 (Sortilin Related Receptor 1) was first reported to be associated with late onset AD in the general population by Rogaeva and colleague [78] and subsequently multiple studies have confirmed the role of SORL1 in late onset AD in multiple ethnic groups, including Europeans, Hispanics, African-Americans, and Israeli-Arabs [79–81]. The variants in SORL1 influence neuropathology by altering Aβ peptide levels and interfering with APP trafficking [82]. Extending the studies to adults with DS, Lee and colleagues [83] investigated seven variants in the gene for SORL1 to assess whether they influence age at onset and risk of AD in this population. Homozygosity for the minor allele in rs556349 and in rs536360 was associated with later age at onset and reduced risk of AD (HR = 0.26, 95% CI: 0.08–0.86; and HR = 0.40, 95% CI: 0.16–0.98, respectively). Mean age at onset was delayed by four years in individuals when homozygous carriers were compared with those who had at least one major allele. These findings indicate a modest association of variants in SORL1 with AD in this population with DS. When compared with earlier studies, variants associated with reduced risk found by Lee and colleagues were not the same as those identified in the original publication [78], suggesting that these SNPs may be in linkage disequilibrium with putative functional variants. Further studies are needed to identify functional variants that influence AD risk in this uniquely vulnerable population.
Recently identified genes associated with AD in the general population
To date, genes associated with AD in adults with DS have been limited due to a relatively small number of candidate gene studies, and these genes are likely to explain only a small proportion of the total genetic contributions toward AD in adults with DS. Thus it is necessary to examine whether or not genes associated with AD in the general population are also relevant in adults with DS. Clearly, studies of AD in DS are well suited to understand the amyloid and tau pathways; however, there are multiple biologically relevant pathways that have been identified in the general population but not yet been explored in DS. The following table lists candidate genes and potential pathways—ranging from immune system/inflammation (see also Chapter 3), to lipid transport to cellular functions such as endocytosis—that have been reported in DS, familial AD, and sporadic AD in the general population (Table 1).
Table 1
Genes | Molecular pathway |
---|---|
Familial AD | |
APP, PSEN1, PSEN2 | Amyloid pathway |
Down Syndrome AD | |
APP (Chr21), APOE, PICALM, CST3, SORCS1, SORL1, CALHM1 | Amyloid pathway |
APOE, S100β (Chr21), IDE | Immune system/inflammation |
IDE | Insulin resistance |
APOE, SORL1, SORSC1 | Lipid transport and metabolism |
PICALM, SORL1 | Synaptic cell functioning/endocytosis |
DYRK1A (Chr21), MARK4 | Tau pathology |
RUNX1 (Chr21) | Gene regulation |
Nonfamilial (sporadic) AD | |
APOE, SORL1, CLU, CR1, PICALM, BIN1, ABCA7, CASS4, PLD3 | Amyloid pathway |
APOE, CLU, CR1, EPHA1, ABCA7, MS4A4A/MS4A6E locus, CD33, CD2AP, HLA-DRB5/DRB1 locus, INPP5D, MEF2C, TREM2/TREML2 | Immune system/inflammation |
APOE, CLU, ABCA7, SORL1 | Lipid transport and metabolism |
CLU, PICALM, BIN1, EPHA1, MS4A4A/MS4A6E, CD33, CD2AP, PTK2B, SORL1, SLC24A4/RIN3 locus, MEF2C | Synaptic cell functioning/endocytosis |
BIN1, CASS4, FERMT2 | Tau pathology |
PTK2B | Cell migration |
MEF2C, PTK2B | Hippocampal synaptic function |
CELF1, NME8 locus, CASS4 | Cytoskeletal function and axonal transport |
INPPD5 | Microglial and myeloid cell function |
FBXL7 | Phosphorylation-dependent ubiquitination |
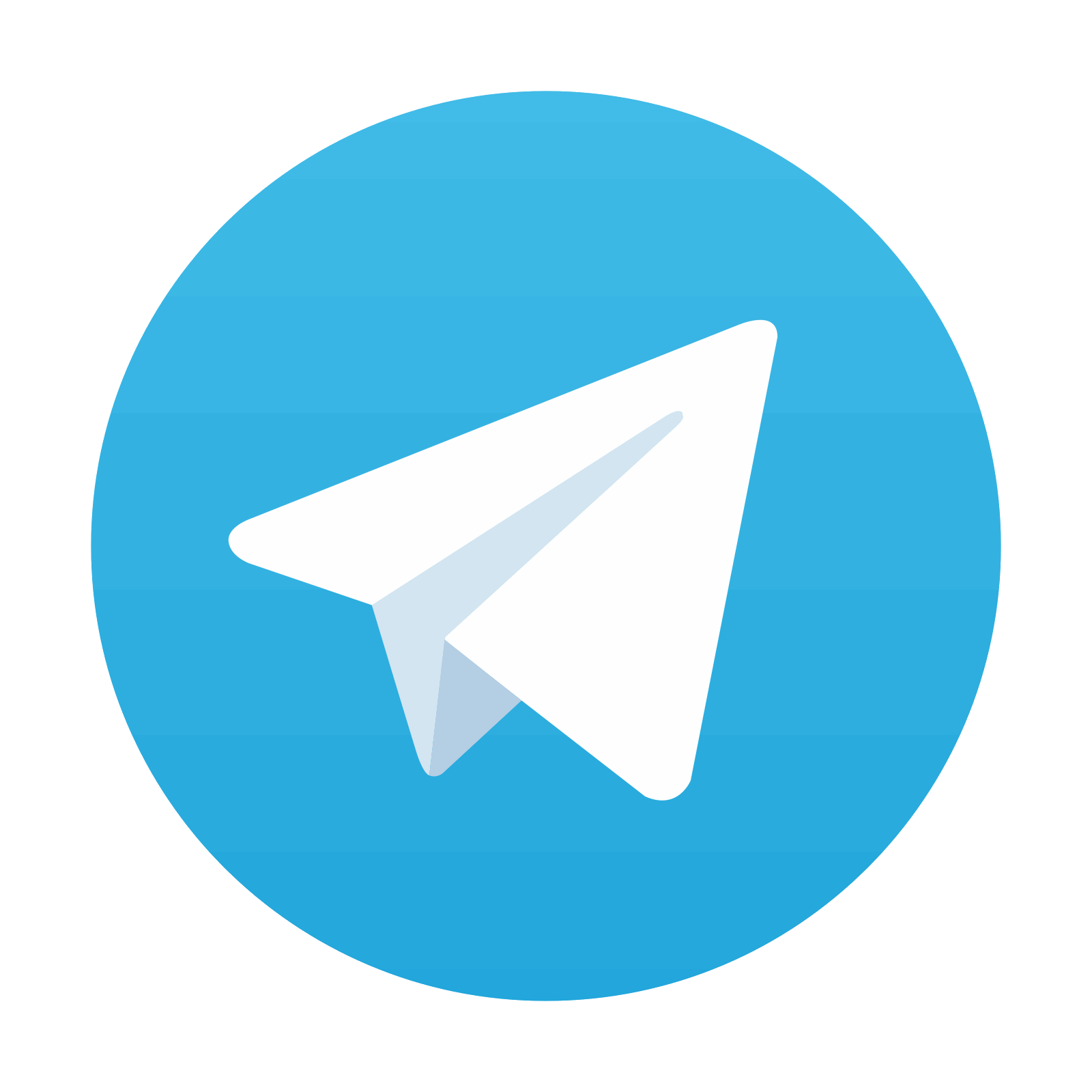
Stay updated, free articles. Join our Telegram channel

Full access? Get Clinical Tree
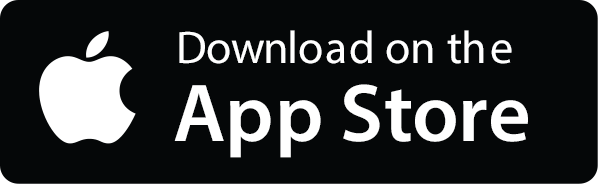
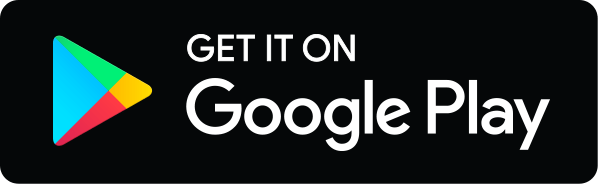