Abstract
Transcranial magnetic-resonance-guided focused ultrasound surgery (MRgFUS) has recently received attention as a novel noninvasive therapeutic option that can be applied as an alternative to the established neurosurgical interventions in the treatment of neurologic disorders such as essential tremor, tremor-dominant Parkinson’s disease, and chronic neuropathic pain syndrome. Furthermore, a recently reported study has suggested that MRgFUS can also be used to treat patients with treatment-refractory obsessive–compulsive disorder (OCD) without inducing side-effects. In this chapter the authors describe the current understanding of the neural circuitry involved in OCD and review the history, general principles, and typical treatment protocols of MRgFUS. The chapter discusses the results of clinical investigations that utilized MRgFUS for patients with treatment-refractory OCD.
Keywords
Anterior capsulotomy, High-intensity focused ultrasound, Magnetic-resonance-guided focused ultrasound surgery, Obsessive-compulsive disorder, Psychosurgery
Outline
Introduction 1045
Neural Circuitry of Obsessive–Compulsive Disorder 1046
History of High-Intensity Focused Ultrasound 1047
Principles of Transcranial Magnetic-Resonance-Guided Focused Ultrasound Surgery 1047
Bilateral Thermal Capsulotomy With MRgFUS as a Treatment for Obsessive–Compulsive Disorder 1048
Patient Selection 1048
Surgical Procedures 1050
Follow-Up Examination and Clinical Evaluations 1051
Clinical Results of the Bilateral Thermal Capsulotomy Using MRgFUS 1051
Controversial Issues 1053
Skull-Related Factors: Skull Density Ratio and Skull Volume 1053
MR-Induced Vertigo 1053
Limitation of the Treatment Area According to the Transducer Frequency 1053
Conclusions 1054
References 1054
Introduction
Obsessive–compulsive disorder (OCD) is a common and disabling neuropsychiatric disorder that affects 2%–3% of the global population at some point in their life; additionally, it is known that 6%–8% of the general population has subclinical symptoms ( ). OCD is characterized by recurrent obsessions (intrusive anxious thoughts, desires, or images) and/or compulsions (repetitive ritualized behaviors used to prevent or reduce distress) ( ). In most cases patients are aware that their obsessive thoughts and compulsive behaviors are unreasonable or illogical, but they have difficulty defying them; in some cases this disorder causes marked distress, anxiety, or significant impairments in daily normal functioning and social relationships. Currently the standard therapeutic options consist of pharmacological therapies, such as selective serotonin reuptake inhibitors (SSRIs), and cognitive behavioral therapy (CBT). Although most patients with OCD will at least partially respond to standard treatment, a considerable number will continue to experience symptoms, leading to chronic functional impairments with little to no relief ( ). In such patients with disabling, treatment-resistant OCD, surgical treatments can be considered.
Several surgical procedures, including lesioning and chronic electrical stimulation of various areas along the neural circuitry associated with OCD, have been used to treat patients with severe refractory OCD. Stereotactic ablative techniques using radiofrequency, such as anterior capsulotomy and cingulotomy, have also been effective ( ). However, such procedures are invasive and the outcomes vary. Additionally, since these procedures are irreversible, concerns have been raised regarding the potential for permanent surgical complications. Compared to radiofrequency thermal ablation, stereotactic radiosurgery (e.g., gamma-knife radiosurgery) is a fully noninvasive procedure with similar clinical results, but the procedure is associated with potentially unpredictable radiation-induced adverse effects, which could be transient or permanent ( ). The biological and clinical responses to radiosurgery are also variable; but the most important limitation of radiosurgery is the considerable delay in the appearance of clinical effects, i.e., weeks to months. Thus both radiofrequency ablative procedures and stereotactic radiosurgery have irreversible features and neither can be adjusted to correct the size and/or location of the target during the procedure.
For the past two decades deep brain stimulation (DBS) has been validated as an alternative therapeutic option to ablative procedures for movement disorders, including Parkinson’s disease, essential tremor, and dystonia. The first study to use DBS in patients with treatment-refractory OCD was performed by , who positioned an electrode within the anterior limb of the internal capsule (ALIC), adapting the anatomical target used in the ablative procedure. Since this initial study the use of DBS in patients with OCD has been investigated with various targets, including the ALIC ( ), ventral caudate ( ), subthalamic nucleus ( ), inferior thalamic peduncle ( ), and nucleus accumbens ( ). DBS has certain advantages over ablative procedures, specifically its reversibility and adjustability. However, it also has disadvantages, including its inherent hardware-related complications, expense, and maintenance demands, as well as the potential for infection and hemorrhage ( ).
Recently a novel thermal ablation procedure using high-intensity focused ultrasound (HIFU), namely magnetic-resonance-guided focused ultrasound surgery (MRgFUS), has been gaining increased attention as a promising and noninvasive alternative to traditional neurosurgery owing to its unique ability to focus acoustic energy through the intact skull and on to a precise target within the brain. Unlike the neurosurgical modalities mentioned above, MRgFUS can be monitored continuously in real time with magnetic resonance imaging (MRI) and MR thermography while avoiding the potential side-effects of ionizing radiation. Several clinical phase I trials have demonstrated the feasibility and safety of using MRgFUS to treat various neurological disorders, such as chronic neuropathic pain syndrome ( ), essential tremor ( ), and tremor-dominant Parkinson’s disease ( ). In a previous study we also demonstrated that bilateral anterior capsulotomy with MRgFUS can be utilized to treat patients with medically refractory OCD without side-effects ( ).
In this chapter we describe the current understanding of the neural circuitry of OCD and review the history, general principles, and typical treatment protocols of MRgFUS. The chapter also discusses the clinical results of studies that applied MRgFUS to patients with treatment-refractory OCD to establish its clinical feasibility.
Neural Circuitry of Obsessive–Compulsive Disorder
Despite the remarkable developments in neuroimaging techniques and the abundant experience gained in performing functional neurosurgeries, the exact pathophysiology of OCD currently remains unknown. Several studies that examined OCD using positron emission tomography demonstrated increased regional cerebral blood flow and metabolic activity within the orbitofrontal cortex, anterior cingulate cortex (ACC), and caudate nucleus ( ) which could be magnified by provoking OCD symptoms ( ). Interestingly, decreased activity in the dorsolateral prefrontal cortex was also noted in individuals with OCD ( ). Executive functions, such as planning, are often impaired in OCD patients, and functional MRI during planning tasks revealed decreased frontal–striatal responsiveness, mainly in the dorsolateral prefrontal cortex and caudate nucleus ( ). Frontal–striatal control of the limbic structures which mediate the inputs to the amygdala may also be decreased in patients with OCD and may be responsible for certain clinical manifestations, including the fear of contamination ( ). These findings indicate that abnormalities in the frontal–striatal neural circuitry play important roles in the pathogenesis of OCD.
Based on these findings, the general organization of the neural circuits involved in OCD is as follows. These circuits project from several separate but functionally connected specific territories in the frontal cortex to corresponding targets within the striatum, and then connect to the basal ganglia and thalamus via direct and indirect pathways, finally sending recurrent projections back to the original frontal territory where each loop began ( ). A multicircuit hypothesis of OCD mentioned that the primary pathogenic mechanism is dysregulation of the basal ganglia and limbic–striatal circuitry, which modulate neuronal activity in and between the orbitofrontal cortex and ACC ( ). In more simplified terms, the neural circuitry involved in OCD consists of three components ( ): the cortico–thalamic pathway, a positive feedback loop from the orbital and prefrontal cortex to the thalamus via the ALIC; the cortico–striato–thalamo–cortical loop, an inhibitory pathway connecting the orbitofrontal cortex, caudate nucleus, globus pallidus, and thalamus that receives serotonergic projections from the midbrain into the striatum; and portions of the limbic system, including the hippocampus, mammillary bodies, and fornix, linking to the thalamus and ACC. Because excessive stimulation of the cortico–thalamic pathway or decreased inhibition of the cortico–striato–thalamo–cortical loop might lead to OCD symptoms, the ALIC, ACC, and nucleus accumbens are often used as therapeutic targets in patients with OCD ( ).
History of High-Intensity Focused Ultrasound
The first investigation to use HIFU for noninvasive ablation was performed by Lynn et al. in . They produced focal lesions deep inside the brain and spinal cord, which generated well-demarcated tissue changes through the intact skull without damaging the surrounding nontargeted tissue. However, the delivered ultrasound energy invariably caused thermal injuries to the skin and underlying tissues, including the scalp, muscles, and even the meninges, resulting from absorption of the ultrasound while passing through the skull bone ( ). Moreover, the irregular thickness and inhomogeneous density of the intact skull caused ultrasonic beam aberrations that disturbed focusing. In the early 1950s William and Francis Fry demonstrated that HIFU could be used after a craniectomy to target deep-seated areas of the basal ganglia in primate models ( ); thus in the following years investigations of HIFU used craniectomies to avoid skull heating, ultrasound attenuation, ultrasonic beam distortion, and reflection ( ). Over time, substantial important technical developments were achieved, and as a result several studies investigated using HIFU as a potential alternative modality to contemporary neurosurgical procedures and radiosurgery ( ). However, as distinct from the need to create an acoustic window by removing parts of the skull, another factor limiting the utility of HIFU on the brain was the lack of adequate imaging modalities that could accurately visualize the target and detect real-time temperature changes during thermal ablation.
To overcome this problem, Fry et al. attempted to sonicate through the intact skull without performing a craniectomy in the 1970s and 1980s. In doing so, the authors demonstrated that while it was possible to focus the ultrasound energy in a manner that would create focal brain lesions through the intact skull, the resultant foci were severely distorted and shifted ( ). A solution to this problem only became clinically feasible in the 1990s with the development of the multielements phased array ultrasound transducers; these transducers permitted the focusing of ultrasound beams by correcting for phase distortions based on computed tomography (CT) scans, which were used to measure the skull thickness and density ( ).
The other major revolution that permitted HIFU to be used clinically was the improvement in MRI techniques. MRI, which was developed in the 1980s, has a much better sensitivity than other imaging modalities in terms of target localization and is capable of measuring temperature changes with considerable accuracy. Because of its temperature sensitivity, the focal volume of a thermal lesion can be localized and controlled well before irreversible tissue injury is induced. Thus with the development of ultrasound phased arrays to correct for cranial distortions, MRI to provide intraoperative guidance, and MR thermography to monitor the thermal deposition during treatment, modern HIFU technology is able to overcome its previous limitations ( ).
Principles of Transcranial Magnetic-Resonance-Guided Focused Ultrasound Surgery
High-Intensity Focused Ultrasound
HIFU is thought to exert two types of effects on biological tissues: thermal and nonthermal. As the name indicates, thermal effects stem from the temperature rise resulting from the absorption of ultrasound energy. As numerous ultrasound waves propagate through biological tissue, the ensuing pressure variations cause mechanical and elastic vibrations at the point of convergence of the ultrasound energy, resulting in energy absorption. This absorbed ultrasound energy is transformed into thermal energy, which heats the targeted tissue and is dependent on the intensity of the acoustic energy applied and the absorption coefficient of the tissue involved. The absorbed energy can elevate the tissue temperature of the focal target to 20–30°C above body temperature, causing thermal tissue necrosis owing to irreversible protein denaturation ( ). The nonthermal effects of HIFU are not well established but are thought to arise from cavitation. Cavitation presents when the negative-pressure amplitude t of the acoustic wave causes liquid components to fail under tension, resulting in the formation of gas- and/or vapor-filled “cavities” or bubbles ( ). These bubbles (predominantly at tissue interfaces) oscillate in the acoustic field (stable cavitation), can grow rapidly and collapse violently (inertial cavitation), and can change the permeability of cell membranes or lead to the complete destruction of tissue ( ).
Magnetic-Resonance-Guided Focused Ultrasound Surgery
The principles of MRgFUS are very simple. Similar to directing sunlight into a small focus using a magnifying glass to generate fire, ultrasound energy can also be focused electronically through the intact skull with specially designed large-scale hemispherical transducers consisting of up to 1000 array elements. This transducer technology permits the beams of ultrasound energy to be electronically steered in a manner that obviates the defocusing effect of the intact skull; thus these transducers not only help to avoid overheating the skull but also can correct for the ultrasound aberrations that are induced by the uneven thickness of the skull. Because of the high-intensity ultrasound energy, the tissue at the focal point heats up within seconds, neural cells are thermally denatured, and well-defined tissue necrosis occurs without damage to the surrounding nontarget tissue. As the name suggests, MRgFUS is a noninvasive thermal ablation procedure that uses both HIFU and MRI to guide and monitor the procedures. As mentioned above, MRI has obvious advantages over other imaging modalities, including superior tissue discrimination and lesion definition, excellent spatial resolution, and improved anatomic context for the surrounding structures. Moreover, from among the other imaging modalities, MRI is the only currently available technique with the proven capability to create quantitative temperature maps for image guidance. This capability is necessary in HIFU ablation therapy because it ensures that the appropriate ultrasound intensities are being applied to ablate the target volume safely and effectively without affecting the surrounding tissues.
Bilateral Thermal Capsulotomy With MRgFUS as a Treatment for Obsessive–Compulsive Disorder
Patient Selection
Because of the notorious legacy of indiscriminate psychosurgery prior to the stereotactic era, in contemporary practice patient selection is the most important issue when considering using neurosurgical techniques to treat psychiatric illnesses, including OCD. According to the guidelines for psychiatric neurosurgery developed based on a report by the National Commission for the Protection of Human Subjects of Biomedical and Behavioral Research ( ), patients who are being considered for neurosurgical intervention should have evidence of severity, chronicity, and disability, and should have shown treatment failure or been refractory to pharmacological and psychotherapeutic treatments. Additionally, patients should be referred to the psychiatric neurosurgery team by their primary psychiatrist, who should also be willing to resume postoperative psychiatric care of the patient. Thus decisions regarding a patient’s candidacy should be made by a multidisciplinary team with unanimous agreement.
Based on these guidelines, we selected and treated four patients with medically refractory OCD using MRgFUS at our institute (Severance Hospital, Seoul, Korea) between February and May 2013. The inclusion criteria were a primary diagnosis of OCD according to the structured clinical interview of the Diagnostic and Statistical Manual of Mental Disorders , fourth edition; at least a 3-year history of OCD symptoms with psychosocial dysfunction (determined by a Global Assessment of Functioning Scale score of <50); a minimum score of 28 on the Yale–Brown Obsessive–Compulsive Scale (YBOCS); and treatment-refractory status—that is, nonresponsive to pharmacological treatment (more than two types of SSRIs at the maximum tolerated dose for more than 12 weeks) and CBT (a minimum of 20 sessions of primarily therapist-guided exposure and response prevention). OCD patients with current or previous psychiatric illnesses, significant cognitive impairment (based on a Mini Mental Status Examination score of ≤24), a history of substance abuse, and/or other neurological disorders that may affect brain function, such as idiopathic Parkinson’s disease or neurodegenerative disease, were excluded. Before MRgFUS all patients were stably medicated, and medication doses were not changed during the entire follow-up period. All patients provided written informed consent before undergoing the procedure, and this study received full ethical approval from the Korean Food and Drug Administration and our local institutional review board. The demographic and clinical characteristics of the enrolled patients are presented in Table 86.1 . Details about the inclusion and exclusion criteria for our study (OCD001) can be found on the United States website for clinical trials ( http://www.clinicaltrials.gov/ct2/show/NCT01986296?term=OCD001&rank=1 ).
Patient No. | Sex/Age (Years) | Primary OCD Symptoms | Psychiatric Comorbidity | Disease Duration (Years) | Current Medication | CBT | Baseline YBOCS | Baseline HAM-A | Baseline HAM-D |
---|---|---|---|---|---|---|---|---|---|
1 | M/24 | Contamination fear/hand washing/counting | Major depressive disorder | 11 | Escitalopram 40 mg | Ineffective | 38 | 34 | 27 |
Valproic acid 250 mg | |||||||||
Alprazolam 0.25 mg | |||||||||
2 | M/29 | Contamination fear/hand washing | Obsessive–compulsive personality traits | 17 | Fluoxetine 30 mg | Ineffective | 34 | 17 | 18 |
Escitalopram 40 mg | |||||||||
Buspirone 20 mg | |||||||||
3 | M/22 | Aggressive obsession/compulsive washing/counting | Major depressive disorder | 13 | Sertraline 200 mg | Ineffective | 35 | 31 | 25 |
Clomipramine 100 mg | |||||||||
Aripiprazole 15 mg | |||||||||
Risperidone 10 mg | |||||||||
Olanzapine 5 mg | |||||||||
4 | F/44 | Pathologic doubt/checking | Major depressive disorder | 24 | Fluoxetine 80 mg | Ineffective | 34 | 26 | 20 |
Olanzapine 10 mg | |||||||||
Mean ± SD | 16.3 ± 5.7 | 35.3 ± 1.9 | 27 ± 7.4 | 22.5 ± 4.2 |
Surgical Procedures
MRgFUS was performed in a clinical 3-T MR scanner (GE Medical System, Milwaukee, WI, USA) using ExAblate 4000 (InSightec, Haifa, Israel) ( Fig. 86.1 ), which featured a 30-cm diameter hemispherical 1024-element phased-array transducer operating at 650 kHz and held by a mechanical engineer. The scalp of the patient was completely shaved to avoid gas bubbles in the circulating degassed water and inspect for scars and other lesions that could compromise the passage of the ultrasound waves. The head was fixed with an MRI-compatible stereotactic frame (Cosman–Robert–Wells frame, Radionics, Burlington, MA, USA) following the application of local anesthesia. Once the frame was in place, a flexible silicone membrane with a central hole was fitted over the patient’s head and sealed to the outer face of the transducer. This membrane held the degassed and chilled (15–20°C) water that circulated in the area between the head and the transducer and served as the medium through which the ultrasound waves traveled. This membrane was tight enough to prevent water leakage, but did not impede blood circulation to the scalp. Before the treatment all required preoperative checks were performed, including blood pressure, O 2 saturation, and electrocardiogram monitoring. After entering the MRI room the stereotactic frame was coupled to the table, which was part of the ExAblate 4000 device, and the patient underwent a series of anatomical MRI scans; the MR images were fused with the preoperative CT scans to perform the skull correction algorithm. Next, the neurosurgeon reviewed the images on a workstation, identified a target volume and location, delineated the treatment contours on the images, and planned the therapeutic strategies. We targeted the bilateral ALIC for OCD (7 mm anterior to the anterior margin of the anterior commissure in the same anterior commissure–posterior commissure plane, extending 2–3 mm along the capsule in a coronal view). During the procedure an ultrasound transducer generated a point of focused ultrasound energy, referred to as a “sonication.” Patients underwent several preoperative test sonications of 10 s each to induce a peak temperature of 40–42°C. These temperatures are unlikely to cause tissue coagulation but are easily visualized by MR thermometry. By this step, the exact location of the focus was confirmed and the overall safety profile of the applied sonication parameters could be validated. Then therapeutic sonications were implemented using an iterative process guided by MRI and MR thermometry, with stepwise increases in acoustic power and energy to achieve a peak temperature in the target region of 51–56°C for >3 s, until the prescribed treatment volume was covered. Before and after every sonication, the patients were evaluated physically and neurologically to determine whether the MRgFUS capsulotomy procedure had induced any adverse effects. In our experience, the number of sonications required over the entire lesioning procedure ranged from 23 to 36, each with a duration of 10–31 s, and the overall procedure duration was 5−7 h. The MRgFUS system was equipped with two “stop sonication” buttons, one for the patient and one for the treating physician, so in the event of discomfort or pain the patient could abort the sonication at any time by activating this button.

Following the treatment procedure the patient was removed from the table and a neurological examination was performed in the recovery room. The patient was taken to the Neurosurgical Intensive Care Unit for recovery and overnight neurological observation. After evaluating the patient’s neurological status and symptoms, the neurosurgeon decided whether to discharge the patient. Patients who were neurologically unstable remained in the hospital until the neurosurgeon indicated their discharge. If clinically stable, the patient was discharged the day after the procedure. As mentioned above, the patient’s previous medications were kept stable for at least 30 days before MRgFUS and the dose was maintained until the last visit. One week after MRgFUS patients were encouraged to utilize the cognitive and behavioral skills they had learned previously during the exposure and response prevention CBT.
Follow-Up Examination and Clinical Evaluations
Clinical outcomes were assessed with the YBOCS, Hamilton Rating Scale for Depression (HAM-D), and Hamilton Rating Scale for Anxiety (HAM-A) by the same psychiatrists at baseline, 1 week, and 1, 3, and 6 months after MRgFUS. The severity of OCD symptoms was assessed with the YBOCS, which is a reliable and valid instrument widely used in clinical trials for OCD. More specifically, the YBOCS is a 10-item scale in which higher scores reflect symptoms that are more intense, and a score of 24 or more (out of a possible 40) is considered “severe” illness ( ). A 35% reduction in the YBOCS score from baseline is a full-response criterion in trials, and patients with an improvement between 25% and 35% are considered partial responders. Moreover, patients who achieve a YBOCS score of ≤8 are regarded as recovered, and a score ≤16 means that patients are in remission. Potential adverse effects and any changes in a patient’s neurological and physical states were evaluated at every visit by a neurosurgeon and a psychiatrist. Neuropsychological tests, including the Wechsler Adult Intelligence Scale (Korean version), Memory Quotient of the Rey–Kim Memory Test, Controlled Oral Word Association Test, and Stroop test, were also administered to the patients by the psychiatrists at baseline and at 6 months after MRgFUS.
T1-weighted imaging with and without contrast enhancement, T2-weighted imaging, diffusion-weighted imaging, and fluid-attenuated inversion recovery sequences were performed immediately after MRgFUS to detect the lesion. Axial, sagittal, and coronal T1-and T2-weighted fast-spin echo images were obtained before MRgFUS and compared with the images acquired immediately, 1 week, and 1 and 6 months following MRgFUS to identify any changes.
Clinical Results of the Bilateral Thermal Capsulotomy Using MRgFUS
Clinical Outcomes
Before MRgFUS, the average YBOCS score was 35.3 (standard deviation or SD: 1.9; range: 34–38). The observed mean improvement rate in the YBOCS score was 33% (SD: 11; range: 24–47). After applying the classical full-response criterion of an improvement >35% in the YBOCS score, two of the four patients achieved a full response to the surgery during the 6-month follow-up period. In addition, considering the partial-response criterion of a >25% improvement, 75% of the patients were at least partial responders, but all four patients showed at minimum a gradual decline in their YBOCS score. We found that the maximum improvement occurred shortly after MRgFUS, within the first 3 months, and no significant changes were observed during the last 3 months of follow-up. This pattern of improvement is similar to the patterns noted for other ablative treatment modalities ( ).
The mean HAM-A at baseline was 27 (SD: 7.4; range: 17–34), which decreased to 8.3 (SD: 6.0; range: 4–17) at 6 months; the mean HAM-D score at baseline was 22.5 (SD: 4.2; range: 18–27), which also improved to 8.8 (SD: 3.3; range: 4–11) at 6 months after MRgFUS. Both the HAM-A and HAM-D scores showed early improvements following MRgFUS, and these improvements were maintained throughout the 6-month follow-up period. The clinical course of the patients’ YBOCS, HAM-A, and HAM-D scores are shown in Fig. 86.2 . As mentioned above, the full battery of neuropsychological tests was conducted at baseline and at 6 months after MRgFUS; compared to the scores at baseline, no significant score changes were noted after the procedure.

Bilateral thermal capsulotomy with MRgFUS was first performed in the right hemisphere and a signal change appeared in T2-weighted images during sonication of the opposite target. Lesions in the ALIC could not be detected on T2-weighted or diffusion-weighted images during or immediately following sonication. Additionally, minimal enhancement of the lesion was observed immediately after sonication. This enhancement was presumably due to disruption of the blood–brain barrier. One week after MRgFUS the thermal lesions became more prominent and the previously observed enhancement on MR images disappeared. Perilesional edema was also noted 1 week after MRgFUS, but this resolved after 1 month. After 6 months, although high signals were was still observed on T2-weighted images, the total size of the lesions decreased slightly compared to the images acquired at 1 week and 1 month after MRgFUS ( Fig. 86.3 ).

These patterns of MRI changes were different from those observed after MRgFUS in patients with essential tremor. In our experience with MRgFUS thalamotomy ( ), lesions in the ventral intermediate nucleus of the thalamus were visible on T2-weighted images that were acquired during and immediately after sonication. This signal change was prominent until 1 month after MRgFUS, but it faded and finally disappeared on the images acquired at 3 and 6 months after the procedure, respectively. Furthermore, on immediate posttreatment MR images strong rim enhancement was observed at the sonication area, but this enhancement disappeared 1 day after MRgFUS. These different MRI patterns in the ventral intermediate nucleus and ALIC after MRgFUS may be due to the different characteristics of the target regions. The delayed emergence of lesions in the MRgFUS capsulotomy may evolve from demyelination during the subacute and chronic phases of injury, unlike the direct damage to the neurons in the gray matter by MRgFUS thalamotomy; that is, white-matter lesions may initially be more subtle than gray-matter lesions ( ).
Adverse Events
The patients tolerated the procedure well, as most presented with only minor and transient side-effects. All patients complained of several periodic mild headaches of short duration, which did not require analgesics, when the MR thermometry was above 50°C during the sonication. In addition, three of the four patients suffered from various vestibular symptoms such as dizziness, nausea, and vomiting, which lasted until the end of the procedure. These symptoms were considered to be a result of MR-induced vertigo, which is similar to motion sickness and is caused by prolonged exposure to high-field (>2 T) MR. However, no demonstrable and persistent physical, neurological, or psychological complications were observed, including confusion, personality changes, hypomania, apathy, disinhibition, or executive dysfunction; such complications typically occur following radiofrequency thermal capsulotomy, gamma capsulotomy, and DBS.
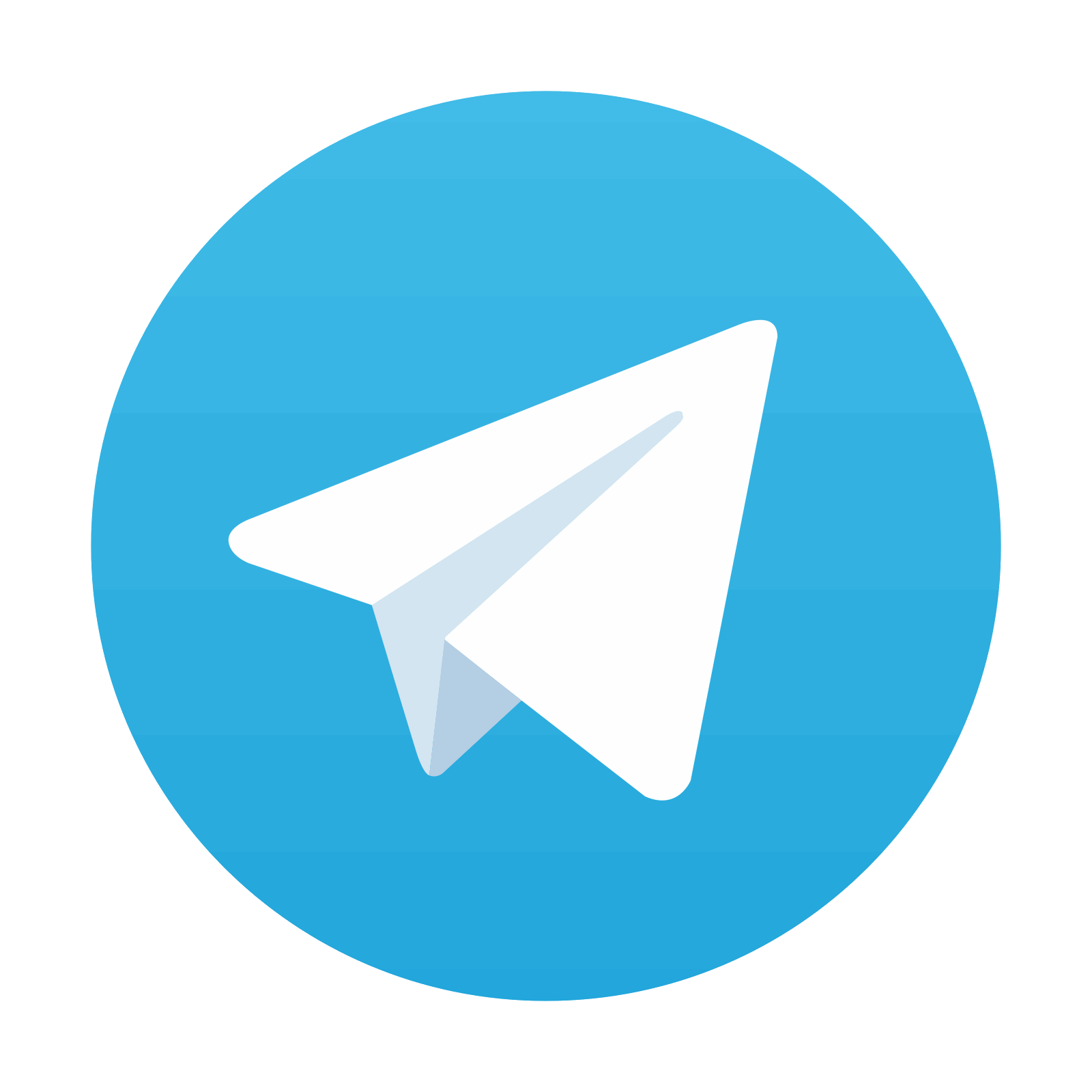
Stay updated, free articles. Join our Telegram channel

Full access? Get Clinical Tree
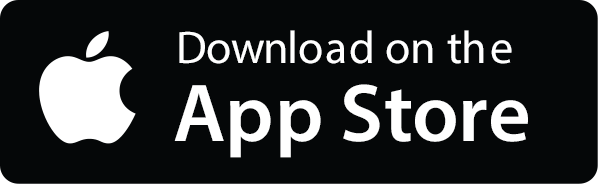
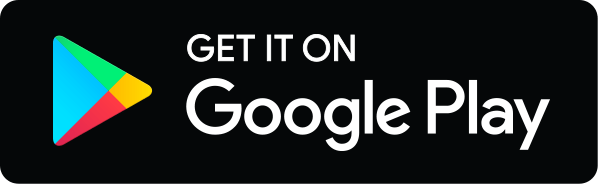