Imaging of the Previously Injured Spinal Cord
Steven Falcone
Up to 95% of acute spinal cord injury (SCI) patients will survive their initial hospitalization (1). This is primarily attributable to improved treatment of spine injury and associated injuries in the field, advances in spinal surgery, and improved recognition and treatment of associated medical conditions. Once past the acute stage, survival will greatly depend on the degree and success of rehabilitation therapy and psychological counseling. Major advances in the management of some of the long-term health medical complications of SCI patients such as bacterial infection related to urinary sepsis, pressure sores, pulmonary infections, and pulmonary emboli have been the primary contributor to improved survival and better quality-of-life for these patients.
Substantial improvement in the survival of the SCI patient has occurred over the recent years. Ten-year survival is inversely related to the severity and level of neurological injury. Patient survival at 10 years is 98% if neurologically normal, 91.8% if an incomplete paraplegic, 90.9% if a complete paraplegic, 86.2% if an incomplete quadriplegic, and 78.2% for a complete quadriplegic (2).
This improved survival leads to a heightened awareness of the neurological issues that are prevalent in this patient population. It has been found that 5% to 10% of patients with a spinal cord injury may suffer worsening motor or sensory function, increasing pain, or dysautonomia (3). Other studies have shown that posttraumatic myelopathy can occur in 0.3% to 3.2% of chronically injured spinal cord patients, and that these changes may ensue as early as 2 months after injury to as late as 36 years after injury (4, 5). If a paraplegic or quadriplegic patient presents with an ascending neurologic level, with loss of motor or sensory function, or with increasing pain, it is important to determine if there is a treatable cause and then make an attempt to preserve or regain function. Progressive myelopathy may be seen with a cord cyst, myelomalacia, and cord tethering (4). However, other less commonly described causes include arachnoiditis, cord compression secondary to spinal instability or bone fragments narrowing the canal, cord tethering, loculated subarachnoid cysts with resultant cord compression, cord atrophy, and microcystic spinal cord degeneration/gliosis (4). Imaging plays a key and crucial role in the evaluation of these patients.
There are essentially five imaging modalities/procedures that may prove useful in the evaluation of the previously injured spinal cord patient who presents with neurological deterioration. These include plain film radiography, computed tomography (CT), myelography with
plain film and CT, magnetic resonance imagery (MRI), and intraoperative sonography/ultrasound.
plain film and CT, magnetic resonance imagery (MRI), and intraoperative sonography/ultrasound.
Indications for Imaging and Imaging Techniques
In SCI patients, either complete or incomplete, the continued preservation of existing sensory or motor neurological function is important to the existing quality-of-life and the prevention of medical complications. For example, the patient may be able to move the thumb and index fingers of one of their hands that might allow for the operation of a motorized wheelchair. Any loss of this motor skill might result in a loss of independence and function. In addition, the loss of sensation of one area of the body that was previously preserved could result in an increased incidence of injury or pressure sores in this part of the body.
Imaging is indicated in those patients who suffer new loss of neurological function, new spasticity or loss of tone, ascending neurological deficit, and pain. The choice of imaging modality depends on many factors, but the combination of plain radiography and MRI can provide the needed information in most instances. In those instances where MRI is contraindicated or when metal artifact precludes sufficient MRI evaluation, CT with or without myelography may be necessary.
Plain radiography of the cervical spine is still an invaluable tool for evaluating the previously injured spinal cord patient. Many spinally injured patients have undergone previous surgery and plain radiographs are useful to check the position of any metallic hardware or bone grafts, to evaluate for signs of metallic hardware loosening, to assess healing of bone grafts, to check the position of graft material, and to look for signs of infection. In both the operated and unoperated spine, plain radiographs are useful to evaluate alignment and to assess for the presence of bony fragments within the canal. Recently, however, the emergence of multidetector CT with multiplanar reconstruction has relegated plain radiography to a slightly less important position in the workup of these patients. Multidetector CT with multiplanar reconstruction is at least as good if not better in the evaluation of the parameters discussed above. Plain radiography, however, is still used and may be best suited for the evaluation of spinal stability.
Delayed instability may be encountered in up to 20% of patients with hyperflexion sprain injury (6). A clinical definition of spinal stability is “the ability of the spine under physiologic loads to limit patterns of displacement so as not to damage or irritate the spinal cord or nerve roots and in addition prevent incapacitating deformity or pain due to structural damage” (7, 8). Spinal instability would therefore be the inability of the spine to limit patterns of displacement. Regarding radiographic evaluation, instability may be present when one of the following are encountered: (a) obvious dislocations and fracture dislocations, (b) 3.5 mm of horizontal displacement on lateral radiograph, (c) >11-degrees kyphosis, (d) widened intervertebral disc space, or (e) splaying of spinous processes (8). Cervical spine flexion and extension radiographs in the previously injured spinal cord patient can provide information as to whether instability is present (Fig. 11-1).
Multidetector helical CT with rapid data acquisition and multiplanar reformations can provide exquisite bone detail and information regarding bony anatomic relationships in the previously injured spinal cord patient including: (a) bony canal or neural foraminal narrowing, (b) spinal alignment, (c) location of bone graft and hardware, (d) determination of adequate fusion, and (e) endplate erosion in the setting of discitis. In addition to standard sagittal and coronal reformations, oblique sagittal reformation may improve confidence in the assessment of neural foraminal stenosis (9).
CT of the postoperative patient who has metallic spinal fixation can be difficult given the associated streak artifacts that can limit evaluation of the implant as well as obscure or distort adjacent anatomy. The streak artifacts occur because the density of the metal is beyond the normal range that can be handled by the computer, resulting in incomplete attenuation profiles (10). X-ray beam hardening, partial volume, and aliasing further compound streak artifacts (10). Reduction of metallic artifact can be accomplished by many methods including increasing the kVp, decreasing the slice thickness, and utilizing various postprocessing algorithms (10). Multidetector CT with the capability of performing submillimeter slices can help to limit this artifact and provides better images than were obtained with single slice methods (10).
MRI has nearly replaced myelography in the evaluation of patients with spinal disease because it is noninvasive, does not use ionizing radiation, is less expensive, is less time consuming, and provides greater contrast resolution. Myelography still plays a role in select patients, particularly when high spatial resolution is necessary. A study by Bartynski and Lin (11) indicates that a myelographic study is useful and better than MRI in predicting lateral recess stenosis in the setting of degenerative disease. Myelography may also be useful in the evaluation of spinal leaks. By convention, its use in this setting is primarily limited to those patients who cannot undergo MRI.
A complete quality myelographic examination should include the combination of fluoroscopic observation, the acquisition of plain radiographic images, and postmyelography CT. Multidetector CT with the application of isotropic voxels allows for exquisite multiplanar reformations that can enhance evaluation. In the previously injured spinal cord patient, it may be useful to perform postmyelographic CT immediately after the myelogram and 4 hours after the myelogram (12). This delayed CT can be helpful to detect spinal cord cysts with contrast diffusing into the cyst on delayed evaluation (12).
MRI has proven extremely useful in the evaluation of patients who develop delayed or progressive neurologic deficits months to years after acute spinal injury (3, 13). MRI is particularly well suited for detection of the pathology encountered in these patients due to its superb contrast resolution and multiplanar capabilities. These pathologies include spinal cord cysts, spinal cord tethering/myelomalacia, and spinal cord compression related to bony deformities or disc herniations.
Special conditions or patient needs may be present when attempting to perform an MRI in the previously injured spinal cord patient. The presence of uncontrollable muscle spasms, ferromagnetic hardware, and respiratory difficulties can negatively affect image quality. It is important that the imager work closely with the patient and the referring physician to provide sufficient medical therapy before imaging so that satisfactory images are obtained. The application of fast scanning techniques and those techniques that reduce susceptibility in the presence of metallic hardware may also be necessary.
T1-weighted and T2-weighted sequences in both the axial and sagittal planes are needed in the complete evaluation of these patients. The use of T2-weighted fast spin echo sequences may decrease magnetic susceptibility artifact in these patients who have often undergone previous surgery with titanium plates or wires in place. Moreover, increasing the receiver bandwidth on fast spin echo sequences can markedly improve the visualization of tissues adjacent to hardware (14). As compared to the acute setting, a T2* sequence is not as useful in the previously injured patient because the detection of blood is not of prime consideration. Additionally, because these patients commonly have metallic hardware, the T2* sequence can be problematic because of susceptibility effects (Fig. 11-2). The addition of a conventional proton density spin echo sagittal sequence can be also beneficial in distinguishing between a confluent spinal cord cyst and myelomalacia. An additional sequence that we routinely employ in the previously injured spinal cord patient is a cerebral spinal fluid (CSF) flow study. Phase contrast CSF cine-flow studies are useful in the confirmation and diagnosis of posttraumatic and postoperative spinal cord tethering with or without subarachnoid cyst formation. CSF flow studies can also be useful in the determination of spinal cord cyst pulsatility (15).
Advanced MRI strategies that are currently in routine use in the brain, such as diffusion weighted imaging, perfusion studies, spectroscopy, and magnetization transfer, are not routinely used in the clinical setting for spinal cord lesions. As MRI hardware improves, these techniques may be used for the evaluation of spinal cord pathology. These advanced techniques may prove to be helpful in the clinical setting as diffusion-weighted imaging with apparent diffusion coefficient (ADC) maps have been shown to detect cystic lesions within spinal cord gray matter following experimental spinal cord injury before they were seen on conventional T1- and T2-weighted images (16).
Intraoperative spinal sonography is a modality that is not widely used in the community; however, it can provide invaluable information to the surgeon while operating
on the previously injured spinal cord patient (17). Although the clinical presentation in conjunction with preoperative imaging determines who will ultimately be operated on, intraoperative sonography can play an important role in improving surgical outcome (17). Since the introduction of ultrasound into the operating room in 1982 (18), high-resolution spinal sonography has provided the spinal surgeon with a view never before possible. Intraoperative spinal sonography provides a 360-degree circumferential view of the operative field, allowing the surgeon to precisely depict and view the canal contents directly opposite the exposure. The surgeon, for example, can view the ventral canal from a posterior approach. Intraoperative sonography provides real-time evaluation that can help limit or minimize damage to neural tissue and can help determine if an adequate result has been achieved. Intraoperative sonography might be critical in distinguishing between a confluent cyst and microcystic myelomalacia and is extremely useful in the evaluation of the patient who is undergoing cyst decompression and cord untethering to determine if adequate cyst decompression and untethering has been achieved. Additionally, intraoperative sonography is useful in the determination of adequate canal decompression of stenotic regions.
on the previously injured spinal cord patient (17). Although the clinical presentation in conjunction with preoperative imaging determines who will ultimately be operated on, intraoperative sonography can play an important role in improving surgical outcome (17). Since the introduction of ultrasound into the operating room in 1982 (18), high-resolution spinal sonography has provided the spinal surgeon with a view never before possible. Intraoperative spinal sonography provides a 360-degree circumferential view of the operative field, allowing the surgeon to precisely depict and view the canal contents directly opposite the exposure. The surgeon, for example, can view the ventral canal from a posterior approach. Intraoperative sonography provides real-time evaluation that can help limit or minimize damage to neural tissue and can help determine if an adequate result has been achieved. Intraoperative sonography might be critical in distinguishing between a confluent cyst and microcystic myelomalacia and is extremely useful in the evaluation of the patient who is undergoing cyst decompression and cord untethering to determine if adequate cyst decompression and untethering has been achieved. Additionally, intraoperative sonography is useful in the determination of adequate canal decompression of stenotic regions.
A portable sonographic machine equipped with preset parameters, high-resolution (7 to 10 MHz) sector, curved array, or linear transducers on a long cord, are satisfactory for image acquisition (17). The transducer and attached cord are placed in a sterile sheath along with sterile gel that serves as an acoustic couplet between the transducer and sterile sheath. Many patients undergo spinal surgery in the prone position and therefore are examined from a posterior approach (17). This must be kept in mind when correlating the sonographic images with the preoperative imaging. Once the surgeon retracts the paraspinal muscles and performs the laminectomy, sterile water can be poured into the operative field to serve as an acoustic path. The tip of the draped transducer is then placed in the water bath, and scanning of the spinal canal and its contents in the transverse and longitudinal planes commences.
Prior to further surgical manipulation, the initial images are reviewed for the pertinent findings and are correlated with the preoperative images. Based on the initial findings, the surgical plan may or may not be altered (17). The progress of surgery is monitored with sonography, and changes are made to the operative approach when necessary. Upon completion of the surgery, final sonographic images are taken to document the results of surgery (17).
There are some limitations to sonography, some of which are unique to intraoperative sonography. A major limitation of intraoperative spinal sonography is the sonographic window. The extent of the laminectomy or amount of bone removed ultimately determines the field of view. Intraoperative sonography cannot be used with microsurgical techniques because a window of at least 1.5 cm by 1 cm is necessary for adequate visualization of the canal and its contents. Bone is not the only substance that may interfere with the transmission of sound waves and obscure the anatomy of interest. Calcification of the dura mater, bullet fragments, and Gelfoam can all obscure anatomic detail. Small amounts of Gelfoam can be confused with pathologic processes, and, therefore, it is desirable to remove all Gelfoam from the operative field prior to scanning.
Clinical Presentation and Pathophysiology of Posttraumatic Myelopathy
In spinally injured patients, delayed or late deterioration of neurological function has been referred to as posttraumatic myelopathy or progressive myelopathy (19). This clinical syndrome has been described using various terms that refer to the clinical syndrome or the pathologic etiology, including chronic injury of the central cervical spinal cord (20), posttraumatic syringomyelia (21, 22), ascending cystic degeneration of the cord (23, 24), posttraumatic progressive myelopathy (13), posttraumatic cystic myelopathy (25), progressive posttraumatic cystic myelopathy (26), and progressive posttraumatic myelomalacic myelopathy (PPMM) (4). The presenting symptoms of these entities may be indistinguishable. The most common pathologic etiologies underlying posttraumatic myelopathy may be divided into the categories of spinal cord cysts and myelomalacia.
Spinal Cord Cysts
Posttraumatic spinal cord cysts affect as many as 3.2% of spinal cord injured patients and up to 8% of patients with complete quadriplegia (27). The incidence is on the rise for a variety of reasons that include increasing survival of SCI patients and improved visualization of the spinal cord with MRI (27). The time of symptom onset after injury ranges from 2 months to 30 years (28), with the most common initial complaint being pain (29). Other presenting symptoms include sensory loss, motor weakness, increased or decreased spasticity, autonomic dysreflexia, hyperhidrosis (above level of injury), sphincter loss or sexual dysfunction, Horner syndrome, respiratory insufficiency, and death (28). The classic sensory dysfunction is a distal loss of pain and temperature sensation with preservation of proprioceptive sensation and light touch (30).
The terminology of cystic cavities in the cord can be confusing. Hydromyelia refers to those cavities that are ependymal lined, syringomyelia to glial lined cavities, and syringohydromyelia to either a combined or indeterminate cyst. Imaging often cannot distinguish between these cysts, and hence the general term spinal cord cyst (or syrinx) may be used for all cysts other than those lesions that are clearly related to a dilated central canal. The most frequently reported pathologic feature of syringomyelia is cavitation of the gray matter adjacent to or directly involving the central canal with an associated inner layer of gliotic tissue (31, 32).
In a monograph published in 1973, Barnett (33) proposed a classification scheme for syringomyelia based on a variety of clinical and experimental observations and studies. This scheme has five divisions: (a) communicating syringomyelia (syringohydromyelia) subdivided into those associated with developmental anomalies at the foramen magnum and posterior fossa contents and those associated with acquired skull base abnormalities, (b) syringomyelia as a late sequel to trauma (posttraumatic cystic myelopathy), (c) syringomyelia associated with spinal cord tumors, (d) syringomyelia as a sequel to arachnoiditis confined to the spinal canal, and (e) idiopathic syringomyelia.
The pathogenesis for the development and growth of posttraumatic cysts remains controversial. It is likely that there are many contributing factors to the development and progression of posttraumatic spinal cord cysts. Cavitation of the spinal cord after trauma is a common pathologic change, as demonstrated by magnetic resonance studies and pathologic series (34, 35, 36 and 37). Posttraumatic spinal cord cyst formation can also be divided into two steps: initial cavity formation followed by cyst extension. Causal factors preceding initial cavity formation may include liquefaction of intraparenchymal hematoma, ischemia due to tethering, arterial or venous obstruction, release of intracellular lysosomal enzymes and excitatory amino acids, and mechanical damage from cord compression. Tethering of the spinal cord also figures prominently in initial cavitation (38).
Blockage of CSF flow has been proposed by Williams (39) as an explanation for spinal cord cyst formation and extension. One of the problems with this theory is that it requires a pressure differential between the ventricular system and spinal subdural space during valsalva maneuver, and subsequently Williams et al. (40) stressed that the majority of cases of traumatic syringomyelia had no evidence of a posterior fossa abnormality that may cause the blockage of CSF flow. It is likely that scar formation within the subarachnoid space plays a vital role in cyst formation and expansion. The tethered cord (which is commonly dorsal in location because of patient recumbency following injury) disrupts CSF flow in the subarachnoid space. The normal motion of the cord and CSF during the cardiac cycle is disrupted. The lack of cord motion results in stress forces that are transmitted to the cord and may initiate cavitation (41) (Fig. 11-3).
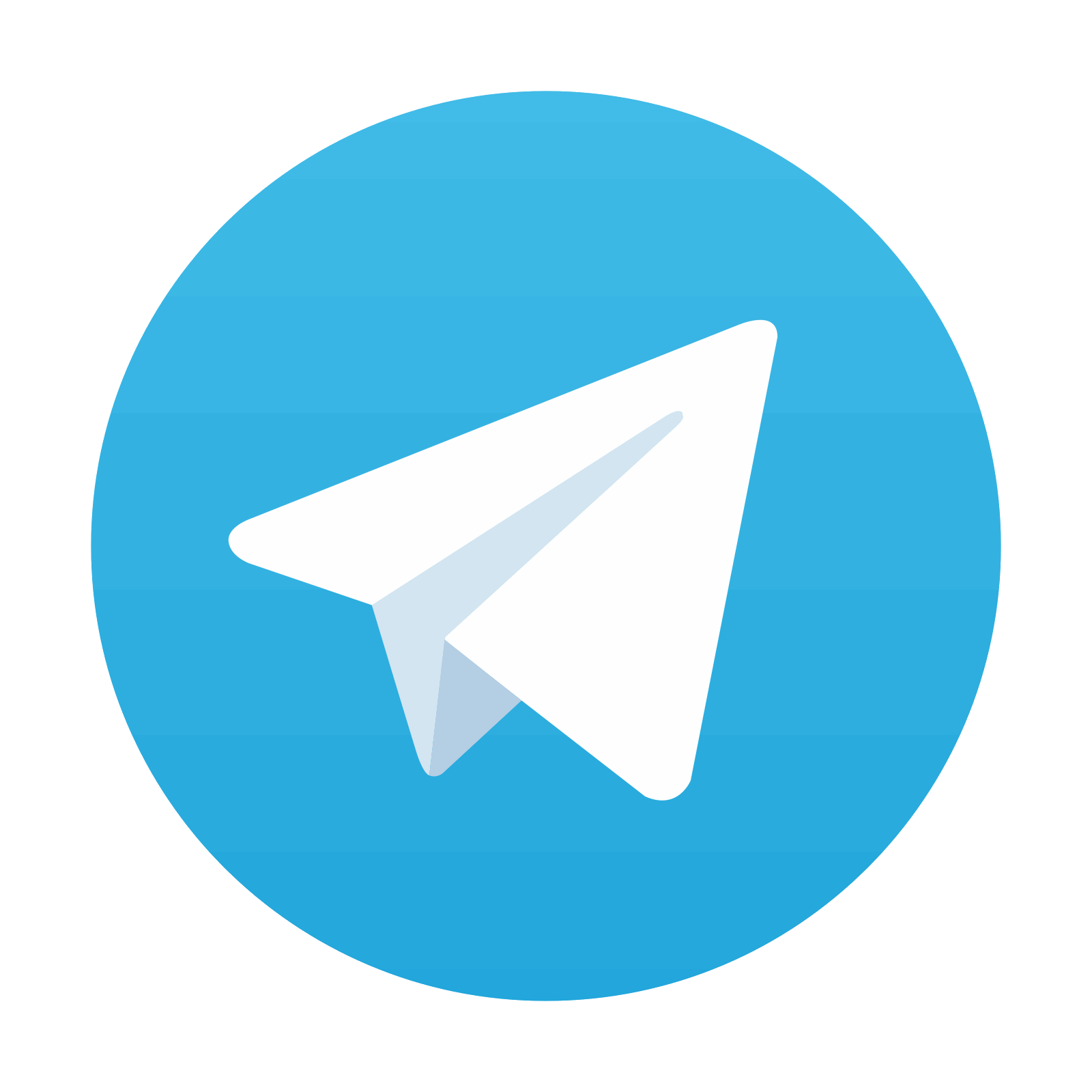
Stay updated, free articles. Join our Telegram channel

Full access? Get Clinical Tree
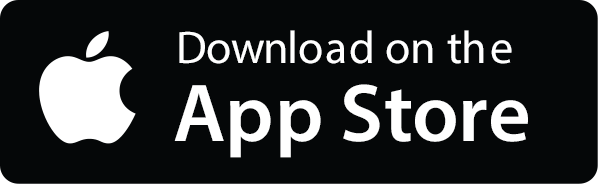
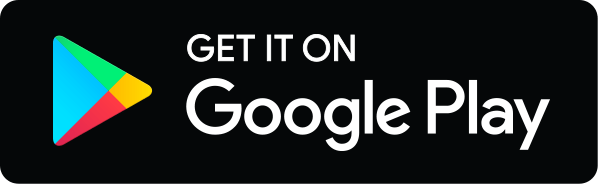