Imaging of Thoracolumbar Spinal Injury
George Koulouris
Amy Y.I. Ting
William B. Morrison
Spinal trauma affects a variety of osteoligamentous supports that make up the vertebral column. The pattern of injury serves as a diagnostic clue as to the mechanism of injury, the latter being critically dependent on the degree of force involved and the force vectors on the portion of the spine affected. The potential for significant mechanical disruption exists, and, therefore, devastating neurologic deficits can occur with irreversible morbidity or even death. The goals of therapy primarily are to stabilize the patient in order to preserve life and to maintain and maximize neurologic function by providing a painless and stable vertebral column.
The imaging evaluation of the traumatized patient with possible spinal injury depends on many variables, including the nature of the injury, whether the patient is conscious, local imaging protocols, and the preference of trauma physicians/surgeons. In addition, institutions, particularly trauma centers, have adopted individual protocols based on what their clinicians feel is best practice given their patient population and imaging facilities available. To date, no standard uniform imaging algorithm is in widespread use; reflecting the complexity of possible spinal injuries, but also on the evolving nature and advances made in the field of diagnostic radiology and trauma. Indeed, controversy exists not only with issues pertaining to diagnosis, but with respect to the selection of surgical candidates, the preferred operative approach, and timing of surgery.
Despite the controversies, certain basic principles exist. Imaging assessment usually commences with radiographs, which are useful in screening the entire spine, without exposure to significant amounts of radiation. Most institutions will radiograph the entire spine as a minimum in the presence of one spinal fracture in order to exclude at least a noncontiguous fracture. The reported time to diagnosis for missed fractures has been recorded as high as 53 days (1). The second injury may be adjacent to the primary fracture (contiguous) or distant (noncontiguous) (2) with almost three quarters of noncontiguous fractures occurring at the cervicothoracic and lumbosacral transition zones (3). Computed tomography (CT), in particular with the advent of multidetector CT (MDCT), allows for excellent depiction of bone detail (particularly the cortex) and superbly demonstrates on multiplanar reformatted views vertebral alignment without osseous and soft tissue superimposition; a significant inherent limitation of radiographs. Even the most subtlesubtlest disruption can be visualized in all three planes, providing improved three-dimensional (3D) appreciation by allowing accurate depiction of osseous displacement. As spinal trauma patients often have coexisting visceral trauma requiring CT evaluation, images obtained from a MDCT study of the abdomen and pelvis can be used to create secondary reconstructions targeted to the spine. Overlapping thin slice multiplanar reformatted images (4,5) and 3D surface reconstructions (6) are of sufficient
quality to obviate the need for standard radiographic assessment (7, 8, 9 and 10). The latter may be performed at the physician’s request as required (11).
quality to obviate the need for standard radiographic assessment (7, 8, 9 and 10). The latter may be performed at the physician’s request as required (11).
Magnetic resonance imaging (MRI) is a complementary examination to CT, often assisting in diagnosing subtle fractures as well as assisting in cases where a possible fracture is identified with CT, but cannot be confidently excluded. Also, minor alterations in alignment, possibly indicative of severe soft tissue injury, may be further evaluated with MRI. The main strength of MRI lies in its exquisite depiction of normal and pathologically affected soft tissue structures. This includes accurate visualization of the intervertebral discs, supporting ligaments, and of most importance, the status of the spinal cord and nerves. Extra- and intra-axial lesions can also be visualized, such as a hematoma, which may be seen in the context of trauma.
Thoracolumbar Anatomy
The vertebral column consists of 32 vertebral segments, each of these segments is further subdivided into the weight-bearing vertebral body and the neural (dorsal) arch, the latter protecting the spinal cord, which occupies the spinal canal. The morphology of the vertebral bodies reflects their function. The vertebral bodies progressively increase in size in the thoracic and lumbar spine, consistent with an increased weight-bearing role (Fig. 7-1). Unique to the thoracic spine are facets for the costal articulations and characteristically long inferiorly directed overlapping spinous processes (Fig. 7-2A). The lumbar vertebral bodies are broad and flat, with large pedicles and facet joints orientated in the sagittal plane (Fig. 7-2B).
Intervening intervertebral discs represent symphyseal articulations, the disc consisting of the tough outer annulus fibrosus and the inner notochordal remnant, the nucleus pulposus. The annulus fibrosus consists of laminated type-I and type-II collagen fibers, which are orientated in an oblique direction (Fig. 7-3). Fibers on either side then run in an opposite direction, a feature designed to resist tensile forces in all directions. The nucleus pulposus is somewhat posteriorly located within the intervertebral disc complex and consists of large macromolecules that trap water molecules, giving this structure its gelatinous and mucoid consistency. Interposed between the disc and vertebral body cortical endplates is a layer of hyaline (articular) cartilage.
Dorsal (spinous process) and lateral (transverse) processes project from the neural arches and serve as levers upon which powerful muscles attach, conferring both mobility as well as dynamic stability when undergoing eccentric contraction (Fig. 7-4). The erector spinae muscle complex lies deep to the thoracolumbar fascia and is divided into three major subgroups, the spinalis
(medial), iliocostalis (intermediate), and longissimus (lateral). These muscles attach to the spinous processes. The thicker multifidus group arises from the sacrum and mammillary processes of the lumbar spine and attaches to the posterior aspect of the laminae and spinous processes. The muscles anterior to the thoracolumbar fascia are the quadratus lumborum, psoas major, and the variably present psoas minor. The psoas major gains its origin from anteromedial aspect of the transverse processes, and annulus and adjacent vertebral bodies from the T12 to first sacral segments. The quadratus lumborum originates from the anterolateral portion of the transverse processes from L1 to L4. Further osseous projections, superiorly and inferiorly, consist of inferior and superior articular processes respectively (with the intervening pars interarticularis), which when interlock with their counterparts above and below and form the synovial facet joints. The orientation of the facet joints is critical with respect to the range and orientation of movement that is permitted in that region of the vertebral column.
(medial), iliocostalis (intermediate), and longissimus (lateral). These muscles attach to the spinous processes. The thicker multifidus group arises from the sacrum and mammillary processes of the lumbar spine and attaches to the posterior aspect of the laminae and spinous processes. The muscles anterior to the thoracolumbar fascia are the quadratus lumborum, psoas major, and the variably present psoas minor. The psoas major gains its origin from anteromedial aspect of the transverse processes, and annulus and adjacent vertebral bodies from the T12 to first sacral segments. The quadratus lumborum originates from the anterolateral portion of the transverse processes from L1 to L4. Further osseous projections, superiorly and inferiorly, consist of inferior and superior articular processes respectively (with the intervening pars interarticularis), which when interlock with their counterparts above and below and form the synovial facet joints. The orientation of the facet joints is critical with respect to the range and orientation of movement that is permitted in that region of the vertebral column.
The ligamentous constraints of the thoracolumbar spine are extensive, functioning to confer stability while allowing motion, and are best demonstrated with MRI (12,13) (Fig. 7-5). The principal ligaments include the anterior and posterior longitudinal ligaments (ALL and PLL) (14), which share a reciprocal relationship with respect to size, with the ALL thinner cranially and thicker caudally. The ALL gains its origin from the basiocciput and inferiorly is broader, terminating anteriorly on the sacrum. Its primary role is to resist extension. At the level of C1 and above, the ALL is continuous as the anterior atlanto-occipital membrane. The PLL is broad in the cervical spine (15) and possesses a serrated appearance throughout its course, being widest at the intervertebral disc level, to which it is adherent though loosely attached to the vertebral bodies. It terminates on the posterior aspect of the sacrum and acts to resist flexion. Both longitudinal ligaments are composed of fibers deep and superficial
relative to the vertebral bodies, the former coursing only over one vertebral body and the latter crossing three to four levels. Superiorly, the PLL continues as the tectorial membrane, attaching to the anterior aspect of the foramen magnum to insert and become inseparable from the dura mater.
relative to the vertebral bodies, the former coursing only over one vertebral body and the latter crossing three to four levels. Superiorly, the PLL continues as the tectorial membrane, attaching to the anterior aspect of the foramen magnum to insert and become inseparable from the dura mater.
The posteriorly positioned ligamentum flavum is composed of elastic fibers (16), giving this ligament its characteristic yellow color. It connects the laminae between vertebral bodies and prevents separation of the posterior structures by acting as a dynamic restraint against excessive flexion. The interspinous ligaments are composed of short fascicles connecting the spinous processes together to adjacent spinous process and arise from the origin of the process to its tip. At the tip, the ligament fuses with the supraspinous ligament, which forms a continuous superficial longitudinal ligament, extending from the sacrum to the C7 vertebral body (and continues to the occiput as the ligamentum nuchae). Both these ligaments act as further restraints of hyperflexion.
The thoracic spine from T1 to T8 is positioned in kyphosis and is less mobile than the cervical and lumbar spine because of the rib cage, smaller intervertebral discs, and the significant overlap provided by the articular facets and associated musculoligamentous supports. The sternum and ribs limit the thoracic spine’s inherent tendency to hyperflex when subjected to axial forces. To an extent, this relative rigidity protects the thoracic spine from injury and accounts for the relative decreased incidence of fractures. The weight-bearing axis of the body is anterior to the thoracic spine, so that the anterior vertebral structures undergo physiologic compression, whereas the posterior aspect is under tension. This anatomy results in a flexion force during axial load injuries with the result of characteristic anterior flexion wedge-type fracture patterns. However, with a greater amount of force, fractures may involve the posterior vertebral body and posterior elements, as well as the ribs.
Several key anatomic points account for the increased susceptibility of the thoracolumbar region to injury. The
thoracolumbar junction is a region of transition (17), the junction of the relatively fixed thoracic spine and the highly mobile lumbar spine and simultaneous transition from a posture of kyphosis to lordosis (Fig. 7-1). The lower two thoracic vertebral bodies possess short ribs, which, unlike at higher levels, possess no sternal articulation and hence offer no additional protection. Furthermore, a sudden alteration in the plane of orientation of the facet joints from coronal (thoracic) to sagittal (lumbar) also accounts for a greater propensity for injuries in this region (18). The orientation of the facet joints is felt to be important with respect to injury mechanism; for example, sagittal-orientated facets, as seen in the lumbar spine (relative to the thoracic facets) allow for a greater range in motion with respect to hyperflexion, and thus such injuries are common (Fig. 7-2). Conversely, injuries secondary to a rotational force are less common. These factors make the thoracolumbar junction (T9 to L3) particularly susceptible to injury; two thirds of all thoracolumbar fractures occur at T12, L1, or L2, and an estimated 90% occur between T11 and L4. Axial load injuries to this region are common, with additional rotation, lateral bending, and shearing vectors demonstrated in this biomechanical transition zone. Also concentrated in this area are flexion/distraction injuries resulting from restraint by automobile lap belts.
thoracolumbar junction is a region of transition (17), the junction of the relatively fixed thoracic spine and the highly mobile lumbar spine and simultaneous transition from a posture of kyphosis to lordosis (Fig. 7-1). The lower two thoracic vertebral bodies possess short ribs, which, unlike at higher levels, possess no sternal articulation and hence offer no additional protection. Furthermore, a sudden alteration in the plane of orientation of the facet joints from coronal (thoracic) to sagittal (lumbar) also accounts for a greater propensity for injuries in this region (18). The orientation of the facet joints is felt to be important with respect to injury mechanism; for example, sagittal-orientated facets, as seen in the lumbar spine (relative to the thoracic facets) allow for a greater range in motion with respect to hyperflexion, and thus such injuries are common (Fig. 7-2). Conversely, injuries secondary to a rotational force are less common. These factors make the thoracolumbar junction (T9 to L3) particularly susceptible to injury; two thirds of all thoracolumbar fractures occur at T12, L1, or L2, and an estimated 90% occur between T11 and L4. Axial load injuries to this region are common, with additional rotation, lateral bending, and shearing vectors demonstrated in this biomechanical transition zone. Also concentrated in this area are flexion/distraction injuries resulting from restraint by automobile lap belts.
The lumbar spine is positioned in lordosis and is very mobile, making it susceptible to varying force vectors. Because of the lordotic curve, the center of gravity is shifted more posteriorly; flexion injuries often straighten the spine and result in an axial load type burst injury, with little or no anterior wedging. The thoracolumbar spine has a higher incidence for visceral trauma when compared to the cervical spine (19). The radiologist must have a high index of suspicion for associated chest injuries, which may be seen in up to 26% of cases (19), as well as being aware of a 6% to 10% (20,21) incidence of abdominal and pelvic visceral trauma. Particular fractures that have extremely high associations with visceral injury require extremely careful abdominal scrutiny, such as the classic Chance fracture, resulting from a flexion-distraction force, which has had a previously reported incidence as high as 89% (22).
Neurologic injury depends partly on type and severity of injury, but is also related to the configuration of the spinal canal and cord. The spinal cord typically terminates at approximately L1 (L2 or L3 in children). The spinal canal size in the thoracic region averages 16 (anteroposterior) by 16 mm (transverse), whereas in the lumbar spine the canal averages 17 (anteroposterior) by
16 mm (transverse). Therefore, relatively minor thoracic trauma can result in significant neurologic compromise, which is also contributed to in part by the close approximation of the cord to the posterior cortex of the vertebral body. In contrast, the lumbar spine is more capacious and has the advantage of the cord terminating as the conus medullaris at the L1 level. Moreover, the nerve roots of the cauda equina, unlike the spinal cord, are relatively resistant to blunt trauma. Hence, a significant burst injury with retropulsed fragments has potential for relatively minimal effect on neurologic status in this region.
16 mm (transverse). Therefore, relatively minor thoracic trauma can result in significant neurologic compromise, which is also contributed to in part by the close approximation of the cord to the posterior cortex of the vertebral body. In contrast, the lumbar spine is more capacious and has the advantage of the cord terminating as the conus medullaris at the L1 level. Moreover, the nerve roots of the cauda equina, unlike the spinal cord, are relatively resistant to blunt trauma. Hence, a significant burst injury with retropulsed fragments has potential for relatively minimal effect on neurologic status in this region.
The spinal cord commences at the level of the foramen magnum as a continuation of the medulla oblongata and extends inferiorly to the level of the L1-2 intervertebral disc (L2-3 level in the newborn), where it terminates as the conus medullaris and its distal cordlike extension, the filum terminale. The latter inserts to the posterior aspect of the coccyx. Surrounding the cord are the typical coverings of the central nervous system, the dura mater (itself surrounded by epidural fat and venous plexus and terminating at the S2 level), the arachnoid and pia mater. Cerebrospinal fluid is present in the subarachnoid space, with the subdural space only being a potential space. The epidural space is a true space, principally composed of fat. Cervical and lumbar enlargement of the cord is secondary to the brachial and lumbar plexus.
Emanating from the spinal cord are tiny rootlets that coalesce to form spinal roots, the ventral (motor) and dorsal (sensory). As the cord terminates at the level of the L1-2 intervertebral disc, the spinal nerves inferior to this level must traverse a long segment of the spinal canal to finally reach their respective neural exit foramen, hence forming the characteristic cauda equina. The exiting nerves in the thoracolumbar spine are numbered from the vertebral body pedicle they pass under; hence, the L1 nerve root emanates from the L1-2 level, under the pedicle of L1.
Fracture Classification and Stability
Vertebral fractures, like fractures in the peripheral skeleton, occur in a predictable and reproducible manner, related to the kind of force applied to the bone. The purpose of a classification scheme is to act as a tool to assist the clinician in determining whether instability (mechanical or neurologic) is present. Classification schemes also allow for research to be reproduced, such that accurate comparison and conclusions are made possible. However, in some instances, classification schemes force stratification of processes that can be better characterized as a continuous spectrum. As such, the different magnitude and complex vectors of force applied to variably mobile vertebral segments, in any of the three regions of the spine, compounded by individual variability and possible concomitant disease processes, allow for an extremely wide variability of injury patterns, which may not fall neatly into a specific class of injury. Thus, if a scheme is relatively simplified, it is considered too broad and not specific enough; one that is comprehensive becomes cumbersome and unwieldy (23) with low interobserver agreement. Introduction of novel classification schemes, which evolve continuously, particularly with emerging imaging technology such as with MRI (24), only adds to the lack of consistency. Nevertheless, knowledge of basic traumatic force vectors, anatomy, and biomechanics allows for a reasonable understanding of injury patterns and hence, a comprehension of the etiology of vertebral column fractures.
As mentioned above, the more comprehensive the classification system, the lower the interobserver agreement. This creates a dilemma for the radiologist who wants to communicate with the referring clinician using relevant terminology. Therefore, as in many other instances of radiologic standard practice, it is prudent and best practice to be descriptive when reporting fractures as opposed to simply subcategorizing an injury into a classification scheme. This allows accurate communication between clinicians and other radiologists and will still remain a historically correct interpretation despite the emergence of further differing classification schemes. However, the radiologist needs to be aware of the relevant findings to report, for example, if there is an anterior wedge fracture indicated on an MRI, or if the integrity of the posterior longitudinal ligament should be described. Essentially, it is not so important to be aware of the classification as it is to understand the concepts of stability and the mechanism of injury. This approach enables accurate communication of the most important information and aids in detection of associated injuries, including any unusual injuries that might change management.
Fractures of the vertebral column are divided traditionally into those affecting the thoracolumbar region (as the fracture pattern and mechanism share many similarities) and the cervical spine. Many theories and classification schemes have been proposed for cervical and thoracolumbar fractures; some deal primarily with mechanism of injury, others with the pattern of fracture (25), still others with stability or neurologic compromise. These systems are still not optimal for the evaluation of stability, prognosis, and management options and are perhaps simplified as two key concepts: mechanical and neurologic stability (either stable or unstable) with each having potential for being acute or chronic. A full discussion of the various classification schemes is beyond the scope of this discussion (Table 7-1). Denis (26) in 1983 reviewed the radiographs, pathology results, and surgical and operative
notes in 412 thoracolumbar injuries and modified the Holdsworth two-column concept to devise the “three-column spine concept” in acute spinal trauma (Fig. 7-6). This classification scheme forms the basis of the contents discussed in this chapter owing to its widespread use and relative simplicity. Though real-life vectors are a complex combination of forces, usually one force predominates to result in a main pattern of injury. Force vectors applied to certain areas of the spine result in predictable patterns of fracture, which essentially serve as a “fingerprint” (27). Force vectors can therefore be divided into two groups; rotational (flexion, extension, lateral bending, and torsion) and linear (compression, distraction, and translation) (28). Thoracolumbar fractures commonly result from falls (29,30) or motor vehicle accidents (31). By applying basic assessment of alignment, bone integrity, joint space, and ligamentous supports, in conjunction with appreciating the mechanism of injury, a highly accurate and clinically relevant radiologic interpretation can be communicated to the referring physician.
notes in 412 thoracolumbar injuries and modified the Holdsworth two-column concept to devise the “three-column spine concept” in acute spinal trauma (Fig. 7-6). This classification scheme forms the basis of the contents discussed in this chapter owing to its widespread use and relative simplicity. Though real-life vectors are a complex combination of forces, usually one force predominates to result in a main pattern of injury. Force vectors applied to certain areas of the spine result in predictable patterns of fracture, which essentially serve as a “fingerprint” (27). Force vectors can therefore be divided into two groups; rotational (flexion, extension, lateral bending, and torsion) and linear (compression, distraction, and translation) (28). Thoracolumbar fractures commonly result from falls (29,30) or motor vehicle accidents (31). By applying basic assessment of alignment, bone integrity, joint space, and ligamentous supports, in conjunction with appreciating the mechanism of injury, a highly accurate and clinically relevant radiologic interpretation can be communicated to the referring physician.
Table 7-1 Evolution of Thoracolumbar Fracture Classification Schemes | ||||||||||||||||||||||||||||||||||||||||||||||||||||||||||||||||||||
---|---|---|---|---|---|---|---|---|---|---|---|---|---|---|---|---|---|---|---|---|---|---|---|---|---|---|---|---|---|---|---|---|---|---|---|---|---|---|---|---|---|---|---|---|---|---|---|---|---|---|---|---|---|---|---|---|---|---|---|---|---|---|---|---|---|---|---|---|
|
Mechanical Stability
Essentially, the mechanically unstable spine is one that undergoes deleterious deformation when subjected to the normal physiologic force vectors and normal ranges of motion; this can be acute or delayed. Denis (26,32) proposed the three-column theory to help predict instability associated with different patterns of injury of the thoracolumbar spine.
The three-column theory of Denis consists of subdividing the spinal column into three anatomical zones: the anterior, middle, and posterior columns (Fig. 7-6). The anterior column consists of the anterior vertebral body,
the anterior longitudinal ligament, and the anterior annulus. The middle column consists of the posterior vertebral body, the posterior longitudinal ligament, and the posterior annulus. The posterior column is comprised of the posterior elements (pedicles, lamina, facets, and spinous processes) and associated ligaments (ligamentum flavum, interspinous, and supraspinous ligaments), as well as the associated facet joint capsule. When only one column is disrupted, the injury is considered mechanically stable. For example, most thoracolumbar spine fractures have a hyperflexion component (27), such as the anterior wedge compression fracture (anterior column involvement only) with intact posterior ligaments. With increasing degree of flexion secondary to greater forces, a second column may be disrupted, hence resulting in mechanical instability. Using the above example, an anterior wedge compression fracture with posterior ligament disruption on MRI (or exceeding 50% loss in vertical vertebral body height anteriorly, considered to have disrupted the posterior ligamentous supports) represents a two-column injury and, therefore, is deemed mechanically unstable. Another typical example is that of a burst fracture, which by definition is a two- or three-column injury. Overall, of the three columns, more importance has been placed on the integrity of the middle column (33,34). The integrity of the middle column has been demonstrated on biomechanical testing to have a higher correlation with mechanical instability when compared to the anterior or posterior columns. In addition, assessment of the integrity of the posterior ligamentous complex is an important determinate for surgical stabilization.
the anterior longitudinal ligament, and the anterior annulus. The middle column consists of the posterior vertebral body, the posterior longitudinal ligament, and the posterior annulus. The posterior column is comprised of the posterior elements (pedicles, lamina, facets, and spinous processes) and associated ligaments (ligamentum flavum, interspinous, and supraspinous ligaments), as well as the associated facet joint capsule. When only one column is disrupted, the injury is considered mechanically stable. For example, most thoracolumbar spine fractures have a hyperflexion component (27), such as the anterior wedge compression fracture (anterior column involvement only) with intact posterior ligaments. With increasing degree of flexion secondary to greater forces, a second column may be disrupted, hence resulting in mechanical instability. Using the above example, an anterior wedge compression fracture with posterior ligament disruption on MRI (or exceeding 50% loss in vertical vertebral body height anteriorly, considered to have disrupted the posterior ligamentous supports) represents a two-column injury and, therefore, is deemed mechanically unstable. Another typical example is that of a burst fracture, which by definition is a two- or three-column injury. Overall, of the three columns, more importance has been placed on the integrity of the middle column (33,34). The integrity of the middle column has been demonstrated on biomechanical testing to have a higher correlation with mechanical instability when compared to the anterior or posterior columns. In addition, assessment of the integrity of the posterior ligamentous complex is an important determinate for surgical stabilization.
Despite the numerous classification schemes for fractures, it is perhaps more important to be familiar with the signs of instability, as this affects functional and neurologic outcome and is at least uniform in its description of the significance of a fracture despite its morphology (Table 7-2) (35).
White and Punjabi (36) proposed a point-scoring checklist for instability, combining clinical and radiologic parameters, with five or more points equating to instability. Disruption of either the anterior or posterior columns is assigned two points each. Cauda equina damage is designated three points, and “dangerous loading anticipated” a further one point. Radiographic signs satisfying criteria for instability are listed and valued at two points (Table 7-3).
Table 7-2 Radiologic Hallmarks of Instability | |||||||
---|---|---|---|---|---|---|---|
|
Neurologic Stability
Denis (32) further classified injuries as neurologically stable or unstable based on neurologic deficit at presentation, or impending neurologic deterioration secondary to impingement upon the spinal cord or nerve roots. Injuries therefore can be classified as having mechanical or neurologic instability, which can be helpful to guide management options. The greater the degree of canal compromise, the greater the probability of neurologic injury, however, the degree of spinal canal compromise is not indicative of the degree of neurologic deficit (37,38 and 39). Considering that the spinal cord terminates in the upper lumbar region, injury at the level of the lower lumbar spine does not result in
cord compression, however, the possibility exists for a conus medullaris or cauda equina syndrome with severe canal compression (40,41).
cord compression, however, the possibility exists for a conus medullaris or cauda equina syndrome with severe canal compression (40,41).
Table 7-3 Radiographic Criteria for Clinical Instability | |||||||||||||||||||||
---|---|---|---|---|---|---|---|---|---|---|---|---|---|---|---|---|---|---|---|---|---|
|
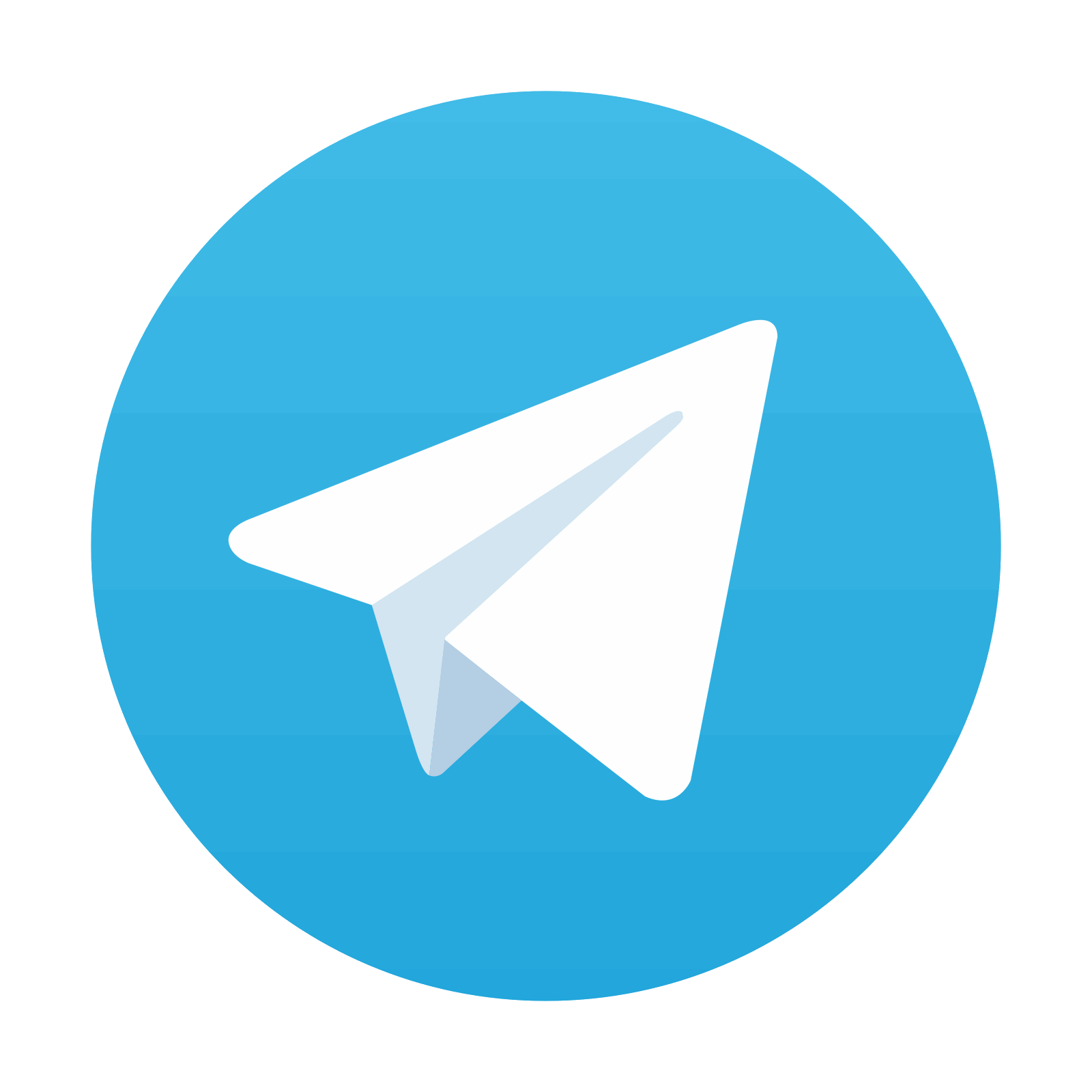
Stay updated, free articles. Join our Telegram channel

Full access? Get Clinical Tree
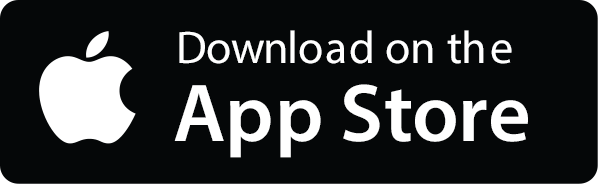
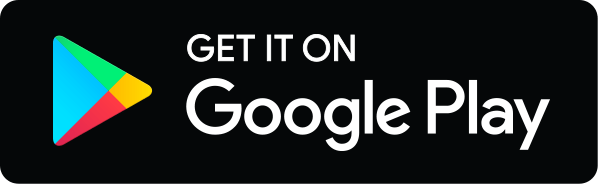