Fig. 1
Backyard chicken liver infected with Toxocara canis. Macroscopically numerous white spots are seen. Cross section revealed the presence of Toxocara larvae
In toxocariasis, OLM is the most common clinical manifestation presenting as a monocular retinitis/uveitis. Granuloma formation in the retina is often misdiagnosed as retinoblastoma. Recently, Shields et al. (2013) reviewed 607 cases of patients having lesions suspected of retinoblastoma and found that 22 % of them were due to toxocariasis. OLM can also occasionally be associated with optic neuritis or loss of eyesight when the parasites have migrated to the optic nerve (Komiyama et al. 1995).
Typical clinical features of toxocaral NLM are eosinophilic meningitis, encephalitis and myelitis, or a combination (Vidal et al. 2003). In cases of acute meningitis, detection of eosinophils in CSF is a strong indication of NLM. When Toxocara larvae are trapped in the small vessels of cortical or subcortical gray or white matter, they will cause vasculitis or microabscesses seen as single or multiple small nodular lesions (<1 cm) by radioimaging (Xinou et al. 2003). Although less common, Toxocara infection also causes myelitis manifested as sensory, motor, and autonomic dysfunctions, predominantly in the lower extremities (Jabbour et al. 2011). Interestingly, only 2 out of 17 patients showed a high eosinophil count in the CSF, while high blood eosinophilia was seen in six patients. Although the effects of Toxocara infection on mental, psychological and/or behavioral status remain unclear, its association with epilepsy has been clearly demonstrated (Quattrocchi et al. 2012). The majority of toxocariasis myelitis cases are caused by T. canis infection; a case of serologically confirmed T. cati myelitis was recently reported in Japan (Fukae et al. 2012). Taira et al. (2011) reported that T. cati larvae can persist and retain high infectivity in the muscles of experimentally infected chickens, posing another risk factor for humans.
2.2 Ascaris suum
Phills et al. (1972) reported that VLM, after intentional infestation of A. suum eggs, causes pulmonary infiltrates, asthma and eosinophilia. Outbreaks of VLM due to A. suum infection were also reported in southern Japan (Maruyama et al. 1996). The possibility of Toxocara VLM due to immunological cross-reactivity between A. suum and T. canis was pointed out by Petithory (1996) in the diagnosis of VLM by immunoblotting technique (Nunes et al. 1997). However, with the improvement of immunodiagnostic methods (Nakamura-Uchiyama et al. 2006), sporadic cases of serologically confirmed A. suum infection have been reported in Japan (Sakakibara et al. 2002; Izumikawa et al. 2011). In the Netherlands, Pinelli et al. (2011) demonstrated the clear-cut difference in the seroepidemiological trends of Toxocara and Ascaris among VLM/OLM-suspected patients and emphasized the importance of A. suum as the pathogen for VLM/OLM.
Like toxocariasis, eosinophilic meningitis/myelitis can be a clinical feature of A. suum larva migrans. A severe encephalopathy due to A. suum infection was reported (Inatomi et al. 1999). A new disease entity, atopic myelitis, has been established by Kira and his colleagues (Kira et al. 2001; Osoegawa et al. 2003; Isobe et al. 2012). This disease is characterized as myelitis associated with high frequency of present and/or past history of atopic disease with hyperIgEemia, high mite-specific IgEemia and high CSF levels of interleukin 9 and CCL11/eotaxin. Several patients with atopic myelitis cases have shown to be seropositive either to A. suum or T. canis (Osoegawa 2004). A spinal cord tumor-like lesion was noted in one case of A. suum infection (Osoegawa et al. 2001). Serial magnetic resonance imaging (MRI) in four patients with myelitis caused by either T. canis or A. suum infections revealed spinal cord swelling with or without gadolinium enhancement in three of them (Umehara et al. 2006). T2-weighted images showed high signal intensities preferentially located in both lateral and posterior columns; one patient with T. canis infection relapsed, which was associated with reappearance of MRI abnormalities.
Treatment of toxocariasis and ascariasis VLM is still controversial (Othman 2012). In general, antihelmintic and corticosteroid treatment was reported to yield improvement in neurologic deficits and spinal lesions. In cases of OLM, treatment is also controversial (Woodhall et al. 2012). The American Society of Ophthalmology recommends corticosteroids and not the use of antihelmintics because Toxocara OLM is considered to be a self-limiting disease and antihelmintic drugs often have side effects of transient liver dysfunction.
2.3 Baylisascaris procyonis
Baylisascaris procyonis is an intestinal nematode of raccoons, Procyon lotor. This parasite is, together with its natural final host raccoons, still prevalent in some areas of the USA (Sexsmith et al. 2009; Yeitz et al. 2009; Hernandez et al. 2013). The mode of infection and the migration route in the hosts are essentially the same as those of Toxocara or Ascaris species. However, differently from other ascarid parasites, a small but potentially devastating number of B. procyonis larvae (typically 5–7 %) enter the CNS leading to the debilitation or death of the intermediate hosts including humans (Gavin et al. 2005). Since the first human case of fatal eosinophilic meningoencephalitis and VLM by this parasite was reported in USA by Fox et al. (1985), up to 20 cases of NLM including recent fatal cases (Wise et al. 2005), mostly in children, have been reported in USA (Gavin et al. 2005; Murray and Kazacos 2004; Perlman et al. 2010). In addition, three cases were reported in Canada; two cases of children (Hajek et al. 2009; Haider et al. 2012) and one case of pathologically proven asymptomatic case in an elderly person (Hung et al. 2012). Baylisascaris procyonis infection is known also to cause OLM (Mets et al. 2003; Brasil et al. 2006; Saffra et al. 2010) or VLM (Boschetti and Kasznica 1995). There is increased risk of B. procyonis infection spreading out from North America to Europe (Bauer 2011) and also to Japan (Sato et al. 2003, 2005).
2.4 Lagochilascaris minor
Lagochilascaris minor is a nematode parasite of sylvatic carnivores found from Central to South America. Natural infections were reported in cats in Argentina (Romero and Led 1985) and Uruguay (Sakamoto and Cabrera 2002), bush dogs, Speothos venaticus (Volcán and Medrano 1991) and Costa Rican ocelots, Felisparadalis mearnsi (Brenes-Madrigal et al. 1972). Experimental infection in laboratory animals revealed that mice were the intermediate host in that Lagochilascaris larvae encysted in the skeletal muscles, while cats are the suitable definitive hosts in that the larvae developed into adult stage in the cat soft tissues around neck, nasopharyngeal region or trachea (Volcan et al. 1992; Campos et al. 1992).
Original description of this nematode was made by the morphological observation of the worms isolated from human neck soft tissue in Trinidad (Leiper 1909). A detailed human case report was first made in Suriname (Winkel and Treurniet 1956) followed by a case from Trinidad and Tobago (Draper 1963). The majority of Lagochilascaris infection in humans is, however, reported from Brazil, especially from Parà state (Moraes et al. 1983, 1985). A few sporadic cases have been reported from several Latin American countries, e.g., Venezuela (Volcan et al. 1982), Bolivia (Ollé-Goig et al. 1996), Mexico (Vargas-Ocampo and Alvarado-Aleman 1997; Barrera-Pérez et al. 2012), Colombia (Moncada et al. 1998), Suriname (Oostburg and Varma 1968). As described by Leiper (1909), adult worms were found in the soft tissues of the neck and also from nasal discharges of patients (Barrera-Pérez et al. 2012). Moreover, as all stages of parasites, (eggs, larvae and adults) were found in the surgical specimens of the nodular lesions of soft tissues around the neck (Barrera-Pérez et al. 2012), auto-infection seemed to occur in humans accompanied by severe clinical symptoms (Rosemberg et al. 1986). Ocular, ear and meningeal involvement was reported in one case (Aquino et al. 2008). Encephalopathy was observed in one of the fatal cases (Rosemberg et al. 1986), but generally CNS involvement is rare in Lagochilascaris infection.
Because of the small number of reported cases, recommendations of chemotherapy for Lagochilascaris infection are not established. Thiabendazole had been used for the therapy of L. minor infection (Oostburg 1971), but this has been gradually replaced by ivermectin (Bento et al. 1993). In vivo larvicidal effect of ivermectin has been shown in mice experimentally infected with this parasite (Barbosa et al. 1998).
3 Angiostrongyloidiasis
Human angiostrongyliasis is an important food-borne helminthic zoonosis, mainly caused by Angiostrongylus cantonensis (Prociv et al. 2000; Wang et al. 2012). A. cantonensis larvae are neurotropic and migrate into the arachnoid space of the human brain to cause eosinophilic meningoencephalomyelitis. Thousands of human cases have been recorded worldwide and outbreaks have been reported in endemic areas. Since the first outbreak was recorded in Wenzho in 1997 (Wang et al. 2002), many outbreaks and sporadic cases have been reported from China (Wang et al. 2008, 2012). The parasite has spread from its traditional endemic regions of the Pacific islands and Southeast Asia to the American continent including the USA, Caribbean Islands and Brazil (Wang et al. 2008, 2012). A recent molecular phylogenetic study on various A. cantonensis isolates from different geographic areas revealed widespread multiple lineages of this parasite (Tokiwa et al. 2012).
Human infections occur by consuming raw or semi-cooked food contaminated with the infective third stage larvae (L3) of A. cantonensis with the majority of infections due to eating intermediate land snails or slugs. Infection also occurs by eating animals harboring infective larvae (planarians, crustaceans, frogs, monitor lizards, wild boar, etc.) or contaminated fresh vegetables (Tsai et al. 2004).
Human angiostrongyliasis is classified into three main forms (1) eosinophilic meningitis, (2) eosinophilic encephalitis or meningoencephalitis and (3) OLM (Sawanyawisuth and Sawanyawisuth 2008; Wang et al. 2008; Diao et al. 2011). Eosinophilic meningitis is the most common form constituting of >90 % of the cases. Eosinophilic encephalitis or meningoencephalitis is less common but often leads to permanent sequelae or death in some cases (Martínez-Delgado et al. 2000; Chotmongkol and Sawanyawisuth 2002; Sawanyawisuth et al. 2009). Hearing loss, intestinal obstruction, radiculomyelitis and Bell’s palsy are rare clinical manifestations of angiostrongyliasis (Sinawat et al. 2008). Postmortem examination of the brain has revealed worms and bilateral changes in the frontal and temporal lobes. Charcot-Leyden crystals, a sign of eosinophil degeneration, were observed in granulomatous meningeal lesions surrounding worms (Tangchai et al. 1967; Punyagupta et al. 1975).
High protein concentrations and high leukocyte counts in the CSF indicate a blood-CNS barrier dysfunction (Lee et al. 2006), which are mediated by several cytokines, i.e. eotaxin, urokinase-type plasminogen activator and matrix metalloprotease-9 (Chen et al. 2006; Intapan et al. 2007; Sanpool et al. 2010).
Diagnosis of human angiostrongyliasis is suggested by clinical manifestation and a coconut juice-like CSF, with eosinophils >10 % of total CSF leukocytes, or >10 cells/mm3 CSF, accompanied by a history of A. cantonensis exposure (Chotmongkol and Sawanyawisuth 2002). A history of exposure should be within 3 months of the onset of the disease. Incubation period varies from 1 day to 6 months (Sawanyawisuth and Sawanyawisuth 2008; Kirsch et al. 2008). For ocular angiostrongyliasis, the worm can be found in any part of the eye.
The eosinophilic-meningitis shows no specific characteristics. Its duration is normally less than 1 week. Fever occurs only in about 10 % of adult patients and half of them have neck stiffness. In encephalitis patients, acute loss of consciousness leading to coma is seen, while seizures have not been reported. In elderly patients, at presentation, prolonged headache and fever are the risk factors for developing encephalitis (Sawanyawisuth et al. 2009). Ocular involvement is rare in angiostrongyliasis. Larva is usually found in the vitreous (Fig. 2) or retina of one side leading to visual impairment (Sawanyawisuth et al. 2007). Optic neuritis, necrotizing retinitis (Sawanyawisuth and Kitthaweesin 2008; Wang et al. 2006; Liu et al. 2006) and oculomotor palsy (Fig. 3) have also been recorded. The ocular form might occur without eosinophilic meningitis. The initial visual acuity is a predictor for vision outcome of this condition. No effective treatment for ocular angiostrongyliasis has been reported and the permanent damage always persists.
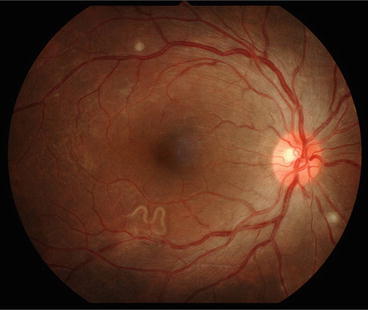
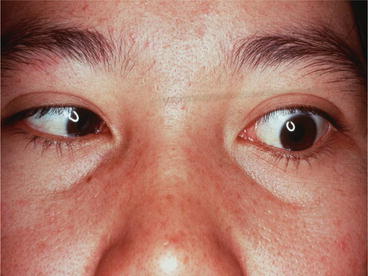
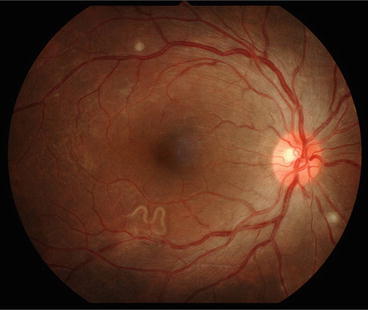
Fig. 2
Ocular angiostrongyliasis. A fundoscopic finding showing the presence of a living A. cantonensis larva in the vitreous chamber. (Courtesy of Dr. Patanaree Luanratanakorn)
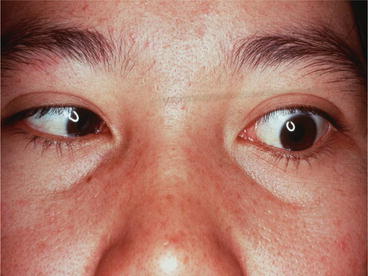
Fig. 3
Left lateral rectus muscle palsy caused by A. cantonensis infection. The patient has been suffering from eosinophilic meningitis. (Courtesy of Prof. Veerajit Chotemongkol)
Although rarely done, definitive diagnosis is based on the detection of larvae or immature worms in the CSF or in the eye. Diagnosis is principally based upon neurological symptoms, CSF eosinophilia and a history of possible exposure to A. cantonensis larvae. CSF is cloudy, but not grossly turbid or xanthochromic. The CSF leukocyte count ranges from about 20 to 5,000 cells/mm3 and is usually between 150 and 2,000 cells/mm3. The CSF eosinophilia exceeds 10 % (ranging from 20 to 70 %), in most cases. The CSF protein level is usually elevated, but glucose level remains normal or only slightly reduced (Schmutzhard et al. 1988; Kuberski and Wallace 1979). Peripheral blood eosinophilia usually accompanies, but does not correlate with, the CSF eosinophilia.
On computed tomography (CT) or MRI imaging, A. cantonensis meningitis can be distinguished from other helminthic infections of the CNS by the presence of focal lesions in the brain (Weller and Liu 1993). MRI imaging of the brain of 13 A. cantonensis eosinophilic meningitis patients in Taiwan (Tsai et al. 2003) showed high signal intensities over the globus pallidus and cerebral peduncle, punctate areas of abnormal enhancement within the cerebral and cerebellar hemisphere on gadolinium-enhancing T1 imaging, and a hyperintense signal on T2-weighted images. MR imaging and MR spectroscopy of six cases of meningoencephalitis in Thailand (Kanpittaya et al. 2000) revealed prominence of the Virchow-Robin spaces, subcortical enhancing lesions, and abnormal high T2 signal lesions in the periventricular regions (Fig. 4, Kanpittaya et al. 2000).
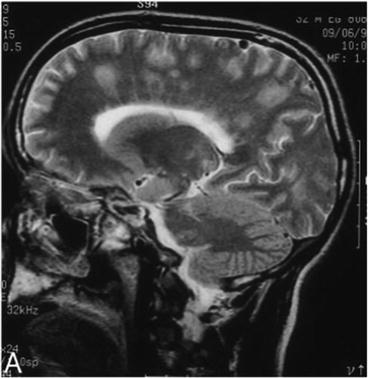
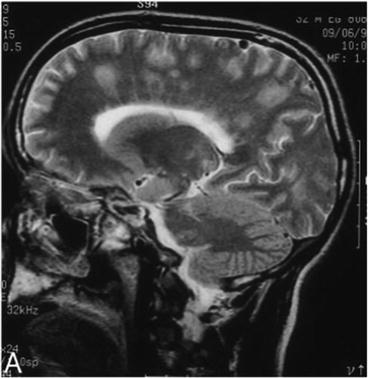
Fig. 4
MR image of eosinophilic meningoencephalitis caused by A. cantonensis. The patient ate raw meat of an infected monitor lizard. Sagittal T2-weighted image showing fuzzy hyperintense signal areas in the left fronto-parietal regions and centrum semiovale. (Kanpittaya et al. 2000)
Among various serological tests developed, ELISA has been widely used and still is the standard by which to evaluate new methods (Intapan et al. 2002). The intrathecal synthesis pattern of IgG1 + IgG2 and IgE antibodies is also useful for the diagnosis of eosinophilic meningoencephalitis due to A. cantonensis (Dorta-Contreras et al. 2003, 2005). The measurement of Th1/Th2 cytokines in the CSF by sandwich ELISA provides a suggestive evidence for the diagnosis of parasitic meningitis (Intapan et al. 2008). By immunoblotting, 29, 31 and 32-kDa antigens are potential diagnostic antigens for immunodiagnosis of human angiostrongyliasis (Nuamtanong 1996; Maleewong et al. 2001; Lai et al. 2005).
An empirical therapy of 2-week course of oral corticosteroid is effective for treatment of eosinophilic meningitis (Chotmongkol et al. 2000). Albendazole therapy was not effective as corticosteroid (Jitpimolmard et al. 2007). The combined therapy with corticosteroid and anthelminthics (Chotmongkol et al. 2004, 2006), or predonisolone and albendazole (Chotmongkol et al. 2004) was not significantly different from corticosteroids alone. Lumbar puncture is required not only for diagnosis but also for the reduction of CSF pressure (Sawanyawisuth et al. 2004a). The recommended analgesic is acetaminophen. NSAID is not superior to acetaminophen (Tsai et al. 2001) and has a risk of causing upper gastrointestinal tract bleeding if combined with corticosteroid. No effective treatment has been established for ocular angiostrongyliasis, although corticosteroid and anti-helminthics may be justified.
4 Gnathostoma Species
Human gnathostomiasis is an important food-borne helminthic zoonosis caused by the spirurid nematode Gnathostoma spp. and is endemic in Asia and the Americas (Miyazaki 1960; Daengsvang 1981; León-Règagnon et al. 2002). The disease has been emerging among travelers returning from the endemic areas (Moore et al. 2003; Katchanov et al. 2011). Generally, gnathostomiasis is not a life-threatening disease, and death has only been recorded occasionally among neurognathostomiasis patients (Daengsvang 1981; Katchanov et al. 2011).
The most common species to cause human diseases in Asia is G. spinigerum. Human infections with G. hispidum, G. doloresi and G. nipponicum were reported only from Japan. In the American continents, G. binucleatum is the only proven species found in humans (Nawa 1991; León-Règagnon et al. 2002). Two outbreaks of gnathostomiasis, supposed to be due to infection with G. spinigerum, were recently reported in Africa (Hale et al. 2003; Herman et al. 2009).
Human infection occurs by consuming raw or inadequately cooked foods, e.g. fresh water fish, frogs, chicken, which harbors Gnathostoma advanced third stage larvae (aL3) (Fig. 5). In the human body, the larvae cannot sexually mature into adults and migrate around for variable periods of time causing irritation and inflammation in various organs. Migration in the subcutaneous tissue causes intermittent painful, pruritic migratory swelling (Fig. 6). Migration to visceral organs can cause various symptoms depending on the affected organs.
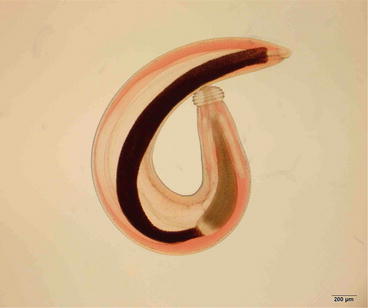
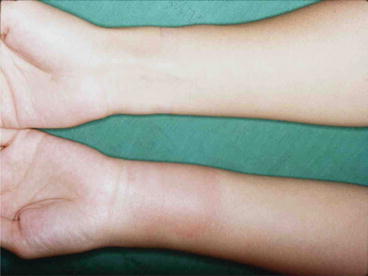
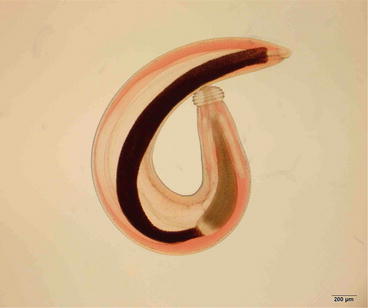
Fig. 5
Advanced third stage larva of Gnathostoma spinigerum. Note cephalic bulb armed with four rows of hooklets (scale bar = 200 μm)
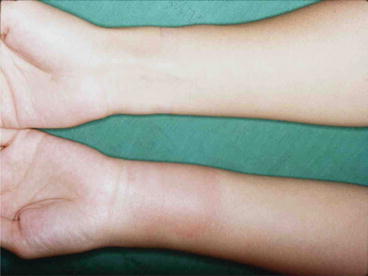
Fig. 6
Intermittent migratory swelling on the lateral forearm. A photo of a gnathastomiasis suspected case
The larvae migrate to the CNS to cause radiculomyelitis or radiculoencephalomyelitis. Subarachnoid hemorrhage can occur, sometimes leading to death (Chitanondh and Rosen 1967; Punyagupta et al. 1968; Boongird et al. 1977). For unknown reasons, G. spinigerum is up to now the only known causative agent of human neurognathostomiasis (Nawa 1991; Katchanov et al. 2011) and cases are almost exclusively found in Thailand (Katchanov and Nawa 2010). Genetic variation among geographically different populations would be of interest to examine in relationships to the pathogenicity of the nematode. G. spinigerum possibly penetrates the CNS by migrating along nerve roots, since the most distinctive clinical manifestation is radiculitis, characterized by excruciating nerve root pain in the extremities or trunk (Punyagupta et al. 1990). Paralysis of one or more of the extremities and urinary retention often develop, followed by involvement of the cranial nerves. Other findings include signs of myelitis and meningoencephalitis (Chitanondh and Rosen 1967; Punyagupta et al. 1968; 1990; Boongird et al. 1977; Schmutzhard et al. 1988; Kawamura et al. 1983). The neuropathologic changes of gnathostomiasis of the CNS arise primarily from mechanical damage caused by a migrating larva (Daengsvang 1981), which possesses hooklets on a head bulb and numerous spines on its body surface. The scattered, multiple hemorrhages with a tract-like appearance in the brain (Fig. 7, Kanpittaya et al. 2012) and spinal cord is a characteristic feature. The areas of hemorrhage differ from the sites of hypertensive hemorrhage (Punyagupta et al. 1968; Bunnag et al. 1970; Kanpittaya et al. 2012). Eosinophilic meningoencephalitis caused by A. cantonensis sometimes shows similar clinical manifestations and can be difficult to differentiate from those caused by G. spinigerum.
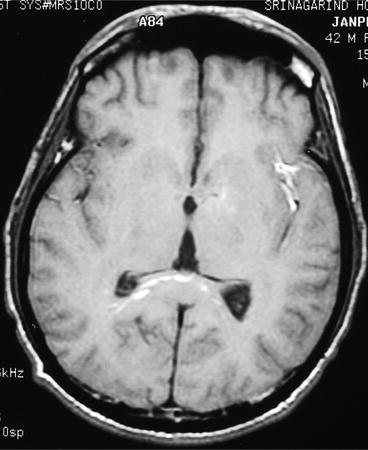
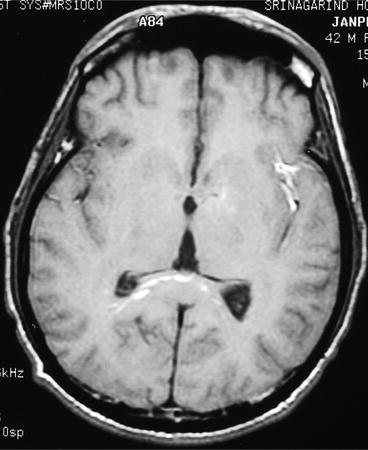
Fig. 7
MR image of cerebral gnathostomiasis. Axial T1-weighted image showing multiple linear, tiny spot of abnormal signals at the splenium of corpus callosum and possible hemorrhagic tract at the left sylvian fissure (Kanpittaya et al. 2012)
Definitive diagnosis of gnathostomiasis relies on identification of worms in biopsy specimen or spontaneous emergence from the skin, but the chance of finding the worms is small. Generally, diagnosis of gnathostomiasis is suspected in patients with a history of eating foods containing infective larvae and a history of intermittent migratory skin and/or subcutaneous swelling. The clinical manifestations of neurognathostomiasis are not specific and difficult to distinguish from the other tissue nematode infections, although xanthochromic or blood-containing CSF is an important diagnostic clue (Rusnak and Lucey 1993). More than half of infected patients have a CSF pleocytosis of less than 500 cells/μl (Punyagupta et al. 1990; Boongird et al. 1977). The degree of CSF eosinophilia may range from 14 to 90 % (Schmutzhard et al. 1988; Sawanyawisuth et al. 2004b). The CSF protein level is usually elevated, while the glucose level remains normal. Concomitant peripheral blood eosinophilia of 10 % or higher is seen in about 55 % of patients (Punyagupta et al. 1968; Boongird et al. 1977; Schmutzhard et al. 1988). The presence of focal lesion at CT scan (Fig. 8, Kanpittaya et al. 2012) shows evidence of large intracerebral hemorrhage. MRI findings that can aid in the diagnosis have been described above, e.g. intracerebral hemorrhages scattered as multiple lesions with a tract-like appearance (Fig. 7) or myelitis patterns in the cervical, thoracic, and conus medullaris areas (Fig. 9, Kanpittaya et al. 2012). In contrast, most of angiostrongyliasis patients have normal brain CT findings, and if any, less parenchymal brain lesions.
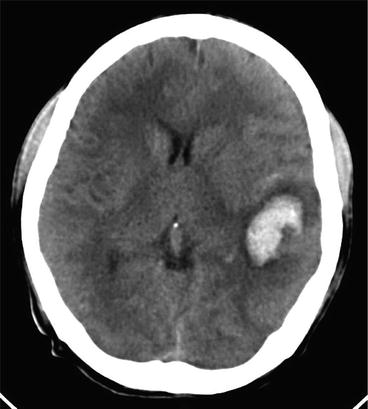
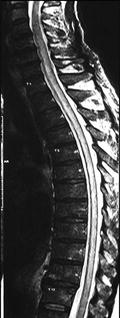
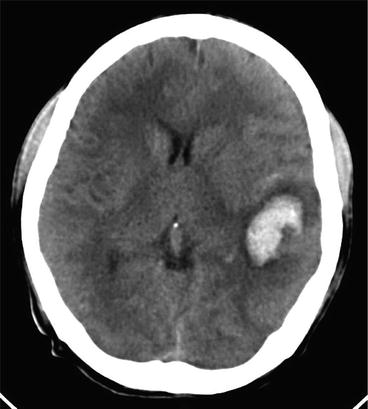
Fig. 8
CT brain image of cerebral gnathostomiasis. Axial non contrast CT image demonstrated intracerebral hemorrhage at the left temporal lobe (Kanpittaya et al. 2012)
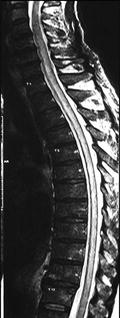
Fig. 9
MR image of spinal gnathostomiasis. Sagittal T2-weighted image showing a long segment of abnormal high intensity from C5 to T12 level (Kanpittaya et al. 2012)
Serological tests can be used as the supportive evidence for diagnosis. ELISA based detection of human IgG class antibody to Gnathostoma antigen has been widely reported (Suntharasamai et al. 1985; Dharmkrong-at et al. 1986; Diaz Camacho et al. 1998; Maleewong et al. 1988). By immunoblot, the 21 kDa and 24 kDa bands in the crude somatic third stage larvae of G. spinigerum antigen recognized by IgG antibody have good diagnostic value for neurognathostomiasis (Tapchaisri et al. 1991; Nopparatana et al. 1991; Wongkham et al. 2000; Anantaphruti et al. 2005; Laummaunwai et al. 2007; Intapan et al. 2010).
Randomized trials of antihelminthic therapies have been reported (Katchanov et al. 2011). Patients showed full recovery after treatment with albendazole (800 mg/day for 1 month, 800 mg/day for 3 weeks or 400 mg twice per day for 4 weeks) combined with prednisolone or dexamethasone (Górgolas et al. 2003; Elzi et al. 2004; Schmutzhard 2007). However, relapses after therapy with ivermectin and albedazole have been observed (Strady et al. 2009).
5 Other Nematodes
5.1 Strongyloides stercoralis
Strongyloidiasis is primarily an infection of the upper intestinal tract caused by the nematode genus Strongyloides. The parasites in this genus are widely distributed from the tropical to the climate zones, and are highly species-specific. In most instances, human strongyloidiasis is caused by infection with S. stercoralis, but infection with other species such as S. fullborni has also been reported (Freedman 1991; Grove 1996).
Strongyloides species have a complicated life cycle. Infective third stage larvae (L3) reside in the humid soil or muddy water and penetrate percutaneously into the hosts. After percutaneous invasion, larvae enter circulation, reach the lungs, and emerge into the alveoli. Then, they are coughed up, swallowed and parasitize in the duodenum to the upper jejunum where they mature into adult female worms. They lay embryonated eggs from which rhabditiform first stage larvae (L1) hatch and are usually excreted in feces. In the freshwater, the L1 develop to L3 that can infect human hosts to complete their parasitic cycle. Alternatively, L1 develop into free living rhabditiform male and female adults in water. Free living male and females mate and lay eggs from which L1 emerge and take one or other path in the life cycle, i.e., either free living or parasitic. In addition, some L1 in the human intestine molt to infective L3 stage while they are descending, and L3 penetrate either through the lower intestinal mucosa or perianal skin to re-enter circulation and complete their life cycle within the same host—“auto-infection”. Because of this recycling system, Strongyloides infection is life-long if not treated. Autoinfection is accelerated in immuno-compromised hosts resulting in a “hyperinfection syndrome” (also known as “overwhelming strongyloidiasis”). Strongyloidiasis hyperinfection syndrome was first seen in soldiers, who went to the endemic areas during the World War II and returned to their home in non-endemic areas (“War Strongyloidiasis”: Grove 1980).
In strongyloidiasis hyperinfection syndrome, CNS involvement is primarily represented by meningitis or meningoencephalitis due to overwhelming co-infections with enterobacteria. In rare cases, there is aseptic meningoencephalitis, assumed to be caused by Strongyloides L3 stage (Foucan et al. 1997; Vishwanath et al. 1982).
As the CNS involvement in strongyloidiasis is mainly due to overwhelming co-infections with enterobacteria, both anti-parasitic and anti-bacterial treatment should be done in parallel. For S. stercoralis, a single dose of ivermectin 200 μg/kg for 2 weeks interval is used a standard protocol. For the cases of bacterial meningitis/menigoencephalitis, antibiotics treatment should be initiated after identification and drug resistance of bacteria. In cases in whom oral or rectal administration of drugs is impossible, or there is poor drug absorption due persistent severe diarrhea, hematochezia and vomiting, subcutaneously injectable ivermectin for veterinary use should be considered (Pacanowski et al. 2005; Takashima et al. 2008).
5.2 Trichinella Species
Trichinellosis is one of the major food-borne parasitic nematodiasis emerging and re-emerging in various parts of the world. Autochthonous trichinellosis occurs in 55 countries where the annual incidence of clinical trichinellosis is estimated to be 10,000 cases with a death rate of 0.2 % (Pozio 2007). In a recent literature survey of 6 international databases for 1986–2009, about 66,000 cases with 42 deaths were reported from 41 countries, the great majority being in the EU, especially in Romania during 1990–1999 (Murrell and Pozio 2011). Trichinellosis affects primarily adults without significant gender difference. Infection mainly occurs by ingesting raw or undercooked pork meat, but the meat of other domestic animals or wild game sources has also been reported.
Until recently trichinellosis was thought to be caused solely by T. spiralis. However, a molecular phylogenetic study identified at least 12 Trichinella species/genotypes (Pozio and Murrell 2006). Some of those species such as T. pseudospiralis, T. papuae and T. murrelli were previously considered as non-human pathogen, but confirmed human cases of infection with those species have been reported repeatedly. Thus, all except one (T. zimbabwensis) species/genotypes are zoonotic pathogens for humans (Pozio and Murrell 2006). Since trichinellosis outbreaks around the world are caused by different Trichinella species with different clinical manifestations, a more comprehensive approach is required for appropriate diagnosis and treatment (Bruschi and Murrell 2002; Dupouy-Camet and Murell 2007).
The life cycle of Trichinella species is maintained by predator/prey relationship of a variety of omnivorous and carnivorous mammals. When humans ingest animal meats containing infective stage of Trichinella larvae (muscle larvae), the larvae are released in the upper small intestine and develop into the adult stage within 48 h p.i. Then male and female adult worms mate and female worm releases newborn larvae in the gut lumen 5 days later [intestinal (enteric) phase]. The larvae immediately penetrate into lymphatic vessels, disseminate systemically via bloodstream, and penetrate into skeletal muscles where they become an infective stage and reside there for weeks to years [muscle phase (parenteral phase)].
Clinical manifestations of trichinellosis largely depend on the worm burden. In low-density infections, patients may remain asymptomatic, while infections with over a few hundred larvae may cause acute diarrhea and abdominal pain at around 2 days post-infection. However, intestinal pathology caused by adult worms and/or by penetration of newborn larvae is not serious compared with the subsequent systemic muscular damages due to migration of disseminated larvae. Although CNS is not a selective or specific target organ of this parasite, involvement can be seen in severely affected patients as part of systemic dissemination of larvae (Neghina et al. 2011). By gadolinium-enhanced MRI, multiple small subacute cortical infarcts may be observed in trichinellosis (Feydy et al. 1996).
The clinical diagnosis of trichinellosis is difficult because there are no specific signs or symptoms for trichinellosis. Epidemiological data such as eating undercooked pork or boar meat in the community are of great importance. Trichinellosis usually begins with a sensation of general discomfort and headache, increasing fever, chills and sometimes diarrhoea and/or abdominal pain. Pyrexia, facial and/or eyelid oedema and myalgia represent the principal syndrome of the acute stage. In severe cases, signs of myocarditis, thromboembolic disease and encephalitis can be observed. By laboratory examinations, leucocytosis (>10,000/mm3) with marked eosinophilia (>1,000/mm3) is observed. Because of the systemic damage of muscles by invading larvae, creatine phosphokinase and lactate dehydrogenase in plasma are increased. A muscle biopsy to detect larvae and the detection of specific antibodies will confirm the diagnosis (Dupouy-Camet and Murell 2007; Gottstein et al. 2009).
The combined treatment of anti-helmintics (mebendazole or albendazole) and glucocorticosteroids is recommended. Mebendazole is usually administered at a daily dose of 5 mg/kg but higher doses (up to 20–25 mg/kg/day) are recommended in some countries. Albendazole is used at 800 mg/day (15 mg/kg/day) administered in two doses. These drugs are usually taken for 10–15 days. The use of mebendazole or albendazole is contraindicated during pregnancy and not recommended in children aged <2 years. The most commonly used steroid is prednisolone at a dose of 30–60 mg/day for 10–15 days, which may alleviate the general symptoms.
5.3 Filaria
Filariasis is a vector-borne disease mainly endemic in tropical and subtropical regions and more than 100 million people are affected (WHO data). There are two types of filariasis: one is a lymphatic filariasis caused by Wuchereria bancrofti and Brugia malayi transmitted by mosquitos; the other type of filariasis is a subcutaneous filariasis caused by Loa loa (see Bisoffi et al. 2014), of which the vector is a gadfly (Chrysops species), and Onchocerca volvulus, of which vector is a blackfly (Simulium species). Onchocerciasis and neurological involvement is reviewed in a companion chapter of this book (Njamnshi et al. 2014). In addition, Dirofilaria immitis, a dog heart worm, causes VLM in humans.
In lymphatic filariasis, peripheral neuropathy due to elephantiasis is common but CNS involvement is extremely rare (Dumas and Girard 1978). Microfilariae of W. bancrofti were found in the cyst fluid of brain tumors (Aron et al. 2002), but its pathogenicity or relation to the clinical symptoms remains unclear. CNS involvement in subcutaneous filariasis is also extremely rare. Encephalopathy (Kamgno et al. 2000; 2008) and meningoencephalitis (Moliner et al. 2011) with the appearance of microfilariae in CSF were observed after ivermectine treatment for loiasis. Similarly, neurological complications with the appearance of microfilariae in the CSF were reported during treatment of onchocerciasis with diethylcarbamazine (Duke et al. 1976). Apart from brain lesions secondary to direct filarial infection, allergic reaction secondary to filarial worm/microfilaria seems to cause recurrent Guillain-Barré syndrome (Bhatia and Misra 1993), meningoencephalitis (Poppert et al. 2009) or acute disseminated encephalomyelitis (ADEM) (Paliwal et al. 2012).
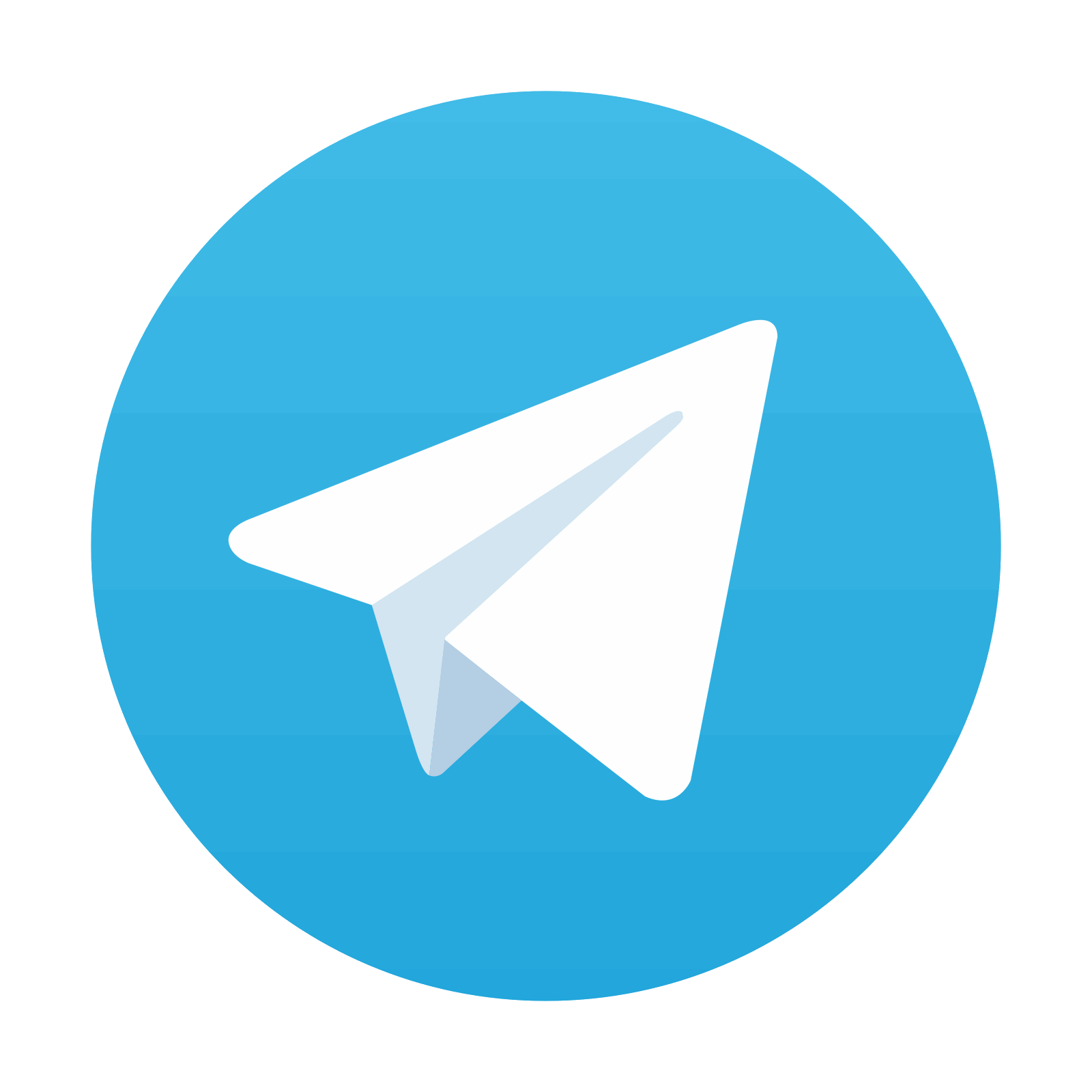
Stay updated, free articles. Join our Telegram channel

Full access? Get Clinical Tree
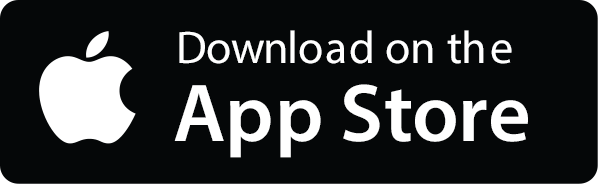
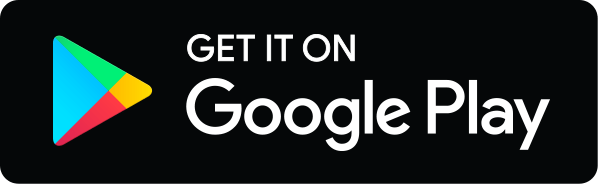