Chapter 2
Laryngeal Sensory Receptors
The larynx subserves several important functions including airway protection, respiration, and phonation, which are derived from a complex interrelationship of diverse reflexive and involuntary actions. The laryngeal reflexes are initiated when chemical or mechanical stimuli contact receptors in the laryngeal mucosa, which trigger afferent neural activity. The reflexes include apnea, swallowing, coughing, bradycardia, hypertension, and changes in peripheral vascular resistance. The laryngeal chemoreflexes have clinical significance and can have fatal consequences as is seen by their association with sudden infant death syndrome.1 The structure, function, and physiologic reflexes produced by these sensory receptors will be discussed.
Morphology of Laryngeal Sensory Receptors
It is well established that the sensory innervation to the supraglottic larynx is derived from the internal branch of the superior laryngeal nerve; below the true vocal folds, sensation is mediated by the recurrent laryngeal nerve.2 Afferent impulses are delivered through the nodose ganglion to the brainstem tractus solitarius. The laryngeal mucosa is the source of afferent neural activity and has been the focus of countless research efforts to better understand the laryngeal sensory system. The most commonly described sensory structures in the laryngeal mucosa include free nerve endings, taste buds, muscle spindles, and glomerular and corpuscular receptors. Investigations are still underway to better define the morphologic and topographic characteristics of these sensory structures and to correlate with specific reflexes. Morphologic descriptions of the different receptor types present in the larynx widely vary because of the study of many different species, ranging from mouse to humans. In addition, it has been difficult to correlate which receptor causes a specific recorded afferent nerve discharge and reflex. Historically, Konig3 in 1881, Simanowsky4 in 1883, Retzius5 in 1892, and Ploschko6 in 1897, all described the sensory innervation of the laryngeal mucosa. Konig, using serial section and light microscopy, identified a fine network of neurofibrils in the subepithelium, approximately 150 to 250 μm for the epithelium, which disappeared when the stratified squamous epithelium of the glottis changed to columnar respiratory epithelium. He described sensory nerve corpuscles resembling retiform and fungiform as well as efferent neurofibrils that project toward the epithelial surfaces. These neural structures were predominantly present in the posterior portions of the larynx. In the anterior glottic region, only isolated neurofibrils and scant free epithelial nerve endings were documented. Some of the fibers become myelinated after they leave the epithelium and others remain unmyelinated. These findings matched those of Jabonero,7 who in 1958, noted subepithelial plexuses, intraepithelial nerve endings, and sensory corpuscles in the mucous membrane of the epiglottis.
More recently, using modern techniques including immunohistochemistry and immunoelectron microscopy, several authors have further clarified the morphology of various sensory structures in the laryngeal mucosa. In 1986 Shin et al8 performed morphologic investigations of sensory nerve endings in human and canine larynges with silver impregnation, immunohistochemistry, and electron microscopy. They discovered intraepithelial free nerve endings with simple and complex branching patterns, corpuscles endings with glomerular patterns, and taste bud-like structures. In the equine laryngeal mucosa, Yamamoto et al9 found the highest density of intraepithelial free nerve endings in the corniculate process of the arytenoid region and lowest in the vocal cord mucosa. Lima-Rodrigues et al10 described intraepithelial nerve fiber endings that protrude into the laryngeal lumen among the cilia of the apical epithelial cells, which may explain the mechanism of airborne airway irritants.
In summary, free nerve endings are found throughout the laryngeal mucosa, from the epiglottis to the subglottis, including in the vocal folds, and they have a wide array of morphology. Although they are located in the subepithelial plexuses, they send projections into the intercellular space of the epithelium, and new evidence points to intralumen projections. These nerve endings are often surrounded by polygonal stratified epithelial cells in the supraglottic region, and by ciliated cells in the subglottic region. Immunologic studies have identified substance P (SP), calcitonin gene-related peptide (CGRP), choline acetyltransferase (CHAT), and nitric oxide in some fibers.
Taste Buds
Taste buds are found mainly in the mucosa of the epiglottis, arytenoid cartilages, aryepiglottic fold, and the posterior glottic region. Morphologically, they resemble the taste buds present at the base of the tongue, although they are not contained in papillae and are not believed to play a role in gustation. Studies of various animals have shown abundant taste buds in the laryngeal surface of the epiglottis, whereas a variable number have been found in human epiglottis. The ultrastructure of the laryngeal taste buds was described by Idé and Munger in 1980.11 They found three cell types in the taste buds of monkeys: basal cells or stem cells; sustentacular cells, which are characterized by the presence of microvilli and dark granules near apices; and chemosensory cells, which project synaptic specializations and microvillar extensions into the surface. Sweazey et al12 compared the ultrastructural details of epiglottal taste buds with those in the oral cavity and concluded that epiglottal taste buds share many of the same structural characteristics as oral taste buds, having basal cells, which did not extend a process into the taste pore, as well as type I (dark) and type II (light) cells, which have synaptic connections with primary afferent fibers. Taste buds contain small clear and large dense-cored vesicles containing neurotransmitters such as SP, CGRP, 5-hydroxytryptamine (5-HT), galanin, and enkephalin (ENK). In some cases, the taste buds were arranged around the opening of the duct of the epiglottic glands. The taste buds function as chemoreceptors and their reflex actions include swallowing and associated apnea, which protect the airway against aspiration.
Other Sensory Receptors
The remaining sensory receptors are less well defined. Glomerular, corpuscular, and lamellar receptors are complex structures with surrounding sheaths, which have been frequently described in the laryngeal mucosa. Their sizes vary and some are found inside the squamous cell epithelium. Their functions have not been well established, but by analogy to the skin, it is likely that they respond to pressure, touch, and cold.13 Sbarbati et al14 in 2004 discovered a new type of chemoreceptor organ organized in plates of a modified, highly permeable mucosa that they coined specific laryngeal sensory epithelium (SLSE). The SLSE contains arrays of solitary chemoreceptor cells (SCCs), which are single epithelial cells contacted by nerves, and are located primarily in highly reflexogenic regions such as the posterior commissure. These chemoreceptor cells had previously been described only in aquatic vertebrates, and their recent discovery in rat laryngeal epithelium encourages further investigations as to their role in the human larynx.
Chemoreceptors
Neurophysiology
As early as 1876, Schofield15 discovered the presence of taste bud–like structures in the laryngeal of the dog and cat. In the first quantitative investigation of epiglottal taste bud–like structures, Lalonde and Eglitis16 counted approximately 1000 receptors in a human newborn. Whether or not these receptors functioned for taste or other stimuli was debated. Bradley et al,17 studying sheep of different ages, noted six stages in the development of epiglottal taste buds identifiable by their changing cellular morphology. The buds of all six morphologic stages were never present in a single epiglottis at any of the ages examined. As the animals matured, the morphologic features of the buds changed until a single population of similar structure was noted, indicating that the stages are distinct landmarks in the development of these sensory structures.
The maturational changes noted early on by Bradley et al,17 along with the large number of these sensory structures, seem to imply an important physiologic role. Wilson,18 reviewing the early literature in 1905, thought the epiglottal taste bud–like structures to be either a phylogenetic residue with no functional role, or to serve as chemoreceptors. He used a laryngeal mirror to apply chemical stimuli to his larynx and noted the ability to discern the four primary tastes, although quantitatively inferior to the tongue. It was postulated that the sensory buds were important multichemical receptors and part of the reflex mechanism protecting the airway. Supporting such a role is the fact that the buds maintain a constant density throughout the life span of an organism. Storey,19 using cat larynges in an electrophysiologic study, demonstrated the response patterns of chemosensitive fibers in the nerve supply of the epiglottis, including specificity. He dissected free the superior laryngeal nerve and severed it near the nodose ganglion. Action potentials were recorded and photographed from single-sensory units consisting of a receptor and afferent fiber using a cathode ray oscilloscope. Receptor units were found by systematically applying a range of chemical stimuli to the exposed laryngeal mucosa. Test solutions included various concentrations of sodium chloride, sodium acetate, calcium chloride in water, sucrose in water, sucrose in Ringer’s solution, as well as acid and alkaline solutions. By dripping these test solutions onto the larynx, together with puffs of air and tactile stimulation, he was able to demonstrate proprioceptive, pressure, tactile, water-sensitive, and hybrid-receptive units responding to multiple chemicals. No receptor was specifically responsive to sodium chloride, sucrose, or acid. He noted that only stimulation of tactile and water-sensitive units were adequate to induce swallowing and coughing, concluding that these were the receptors initiating such reflexes. These receptor units were also noted to have the shortest response latencies and were sensitive to topical anesthetics, indicating their proximity to the mucosal surface. The chemoreceptor discharge patterns associated with reflex swallowing and coughing were noted to be different from those recorded from lingual taste buds. Free nerve endings were therefore thought to be the primary receptors of these two laryngeal chemoreflexes.
Boushey et al20 described the site, method of excitation, and the resulting reflexes produced by chemical irritation of the larynx in cats. By stroking the laryngeal epithelium with a single linen thread containing an applied chemical stimulus, they were able to detect the exact site from which a reflex reaction was initiated. They concluded that the receptors involved were of a hybrid nature, being sensitive to both mechanical stimulation as well as chemical irritants. Two groups of such receptors were established based on their spontaneous electrical discharges: group 1 fibers had little or no spontaneous activity, and group 2 fibers had regular spontaneous discharges. Group 1 units were stimulated by cigarette smoke, NH3, SO2, CO2, and distilled water. The adaptation time was variable, and the units were mechanosensitive. Group 2 units were stimulated by NH3 and distilled water, but inhibited by cigarette smoke, CO2, and had slow adaptation times. This study also confirmed data showing that the superior laryngeal nerve fibers have receptive fields in the supraglottic larynx.
By 1975, the chemoreflexes reported to be associated with chemical stimulation of the larynx included coughing, apnea, bronchoconstriction, laryngeal constriction, increased blood pressure, and swallowing.19–22 However, the specific chemoreceptor units could not yet be matched to the various known reflexes produced. Prolonged and sometimes fatal apnea could be initiated reflexively in newborn lambs breathing via a tracheotomy by the introduction into the larynx of water but not isotonic saline. Preliminary investigation of afferent units in superior laryngeal nerve fibers by Storey and Johnson23 revealed the presence of watersensitive receptors with short response latencies, slow rates of adaptation, and insensitivity to isotonic saline. They considered these to be the chemoreceptor units responsible for the apnea reflex in lambs. Harding et al24 extended the work of Storey and Johnson by recording the action potentials generated in single afferent units of the superior laryngeal nerve with various chemical stimuli in neonatal and adult sheep, cats, and monkeys. Two types of receptors sensitive to water were detected to be present in each species from birth. The most common unit responded after a latency period of less than 1 second, discharged maximally in the first 1 to 3 seconds, and became inactive when the stimulus was withdrawn. The other receptor responded after only several seconds, with the discharge frequency gradually increasing and continuing after removal of the stimulus. Reproducible responses were elicited by tactile stimuli to the laryngeal mucosa over the receptive field of each of the long latency units. The short latency units responded to milk, gastric contents, saliva, and isotonic solutions of sugar. Harding et al believed that the water-sensitive chemoreceptors represented the unspecified epithelial endings previously described by Koizumi.25 It is apparent from these electrophysiologic data that distinct groups of chemoreceptors exist that initiate various chemoreflexes in response to the same or different stimuli.
Bradley et al26 used various concentrations of KCl, NH4Cl, NaCl LiCl, HCl, citric acid, and distilled water to stimulate sheep larynges to better define the chemical specificity of laryngeal chemoreceptors. All the chemicals were dissolved in a solution of NaCl of very low concentration, which elicited minimal activity. The responses recorded in the superior laryngeal nerve fibers varied among fibers and within a single fiber depending on the chemical stimulus. In lambs and ewes, KCl was the most effective chemical stimulus followed by NH4Cl, with NaCl and LiCl being much less effective. HCl was a more effective acidic stimulant than citric acid. The difference in response frequency between each chemical and a solution of 0.5 molar KCl (used as a standard) was calculated for the entire stimulation period. It was evident that plotting the peripheral neural response pattern over time enabled discrimination among salts, between some salts and acids, and between some salts and water during stimulation of the epiglottis. This demonstrated that various chemicals produced characteristic discharge patterns, when decoded by central nervous system processing centers, and initiated an appropriate laryngeal chemoreceptor reflex. Sucrose, glucose, HCl, quinine, and water all initiated reflex apnea and swallowing. Shingai and Shimada27 elicited similar results in rabbits.
Kovar et al28 in 1978 compared the swallowing and apnea reflexes in response to 194 chemical stimuli in lambs. They found that the reflexes did not follow an all-or-none principle but showed a graded relationship between the strength of the stimulus and the respiratory response. They also noted that salts and acids produced different respiratory responses for various stimuli. Data using sugars as stimuli supported the belief that laryngeal taste bud–like structures function as chemoreflex receptors. Lambs reacted with increasing apnea to progressively more concentrated glucose solutions. Potassium gymnetate, known to suppress taste sensitivity to sweet substances by binding to the sweet receptor site of lingual taste buds, markedly reduced this response to glucose. However, the response to saline or water was not altered. Accordingly, there appears to be some contribution by laryngeal taste bud–like structures to specific stimuli-induced chemoreflexes.
The question now arose as to what common features did the various stimulating substances possess that induced excitation of the chemoreceptors. Harding et al24 noted that hypotonicity was not a requirement because receptors were excited by isotonic solutions of sugars, milk, saliva, and gastric juice. They concluded that it was a lack of Na+ or Cl– ions that was responsible for the excitatory effect of the many isotonic solutions tested. Boggs and Bartlett29 used puppies to elucidate further the common features of chemoreceptor stimulants initiating reflex apnea. The laryngeal lumen was perfused with various substances while ventilation through a tracheal cannula was recorded. Water consistently elicited apnea, which could be terminated by a NaCl solution. Sucrose and urea solutions also consistently produced apnea, supporting data indicating that osmolarity is not a critical factor. Phosphate buffer solutions of various concentrations and pH did not produce apnea. Cation substitutions in chloride salts also did not elicit apnea with the exception of potassium. Large anion salts, sucrose, urea, and milk ceased to be effective stimulants in the presence of chloride ion in concentrations of 80 mEq/L or more. This clearly indicated that the common characteristic of apnea-inducing substances is a chloride concentration below that which is normally present in extracellular fluid. This suggests the existence of a receptor normally held in a low ionic conductance state by the presence of chloride ions in the extracellular fluid. The receptor membrane may have specific chloride or other small anion binding sites. Removal of chloride must somehow increase the conductance of nerve membranes to other ions, notably Na+, resulting in depolarization.
It is now widely accepted that the taste buds of the larynx respond to several chemical stimuli and to water. They tend to be stimulated by the pH and the tonicity of the solution, and do not respond to NaCl solutions close to physiologic concentrations (0.154 M), but do respond at both lower and higher concentrations. In addition, stimulation of the larynx with taste stimuli does not give rise to taste sensation. These results reveal a fundamental difference between the chemoreceptors of the oral cavity and the larynx, and lead to the conclusion that chemoreceptors of the larynx do not play a role in gestation but are adapted to detect nonsaline chemicals.
There appear to be species and age-dependent differences in laryngeal chemoreflexes. When adult dogs were tested with known apnea-inducing solutions, little or no effect on breathing was noted, but reflex swallowing or coughing did occur.29 A clear decrease in the magnitude of the chemoreflex-induced apnea occurs after 2 weeks of life, which gradually decreased to an inconsequential level when the puppies reached 7 weeks of age. The maturational component of laryngeal chemoreflex-induced apnea observed in puppies has also been noted in piglets30 and kittens.31,32 It was suggested that the absence of the apneic reflex in mature animals was the result of a maturation of central respiratory control mechanisms.
Age-related laryngeal neural dysfunction is evident by the high incidence of dysphonia and dysphagia encountered in the elderly. Mortelliti et al33 reported an age-related loss of small myelinated nerve fibers in the human superior laryngeal nerve. Rosenberg et al34 also noted age-related changes in the cytoplasm of Schwann cells from the superior laryngeal nerve of Wistar rats, although the number of axons was preserved with age. This suggested that the changes began at the terminal portion of the neurons. In an attempt to correlate changes in laryngeal neural structures with laryngeal dysfunction in elderly cats, Yamamoto et al35 compared the density, distribution, and morphology of various types of sensory nerve endings in laryngeal neurons tissue of rats of different ages. They discovered a significant decrease in the number of taste buds as well as degeneration of nerve endings with age. A variable amount of taste buds were found in postmortem human adult epiglottis, but no comparison was made to that of children.
Chemoreceptors and Reflexes
The initiation and magnitude of chemoreceptors response require significant central nervous system input as well as a modulation from the peripheral system. Although apnea is the chemoreflex most commonly measured in the study of laryngeal receptor function, major cardiovascular reflexes may be initiated by laryngeal chemostimulation. These cardiovascular reflexes may also be duplicated by electrical stimulation of the superior laryngeal nerves and can be abolished by their sectioning, indicating their relationship to the laryngeal chemoreceptors.36,37 The receptors respond to substances that are chemically different from the normal secretions present in the larynx, and therefore have a protective function to prevent aspiration of foreign substance into the airway.
Lee et al38 studied the cardiorespiratory responses to chemoreceptor stimulation in piglets varying in age from 1 to 79 days. The introduction of water or milk into the piglet larynx previously was noted to produce persistent apnea and asphyxial death in a high proportion of animals. Upon the introduction of saline, only transient alterations of blood pressure and respiration were noted. Replacement of saline with water or KCl solutions resulted in reflex apnea and gasping. Accompanying this respiratory response was a rise in arterial blood pressure and fall in heart rate. Electrical stimulation of the superior laryngeal nerves in animals older than 1 month, as compared with younger piglets, did not produce an apneic reflex, with only a mild reduction in respiratory rate and depth noted. No significant hypertension or bradycardia was recorded either. These findings support the concept that important changes in the respiratory control system occur as the organism matures. This has important clinical implications in the pathophysiology of sudden infant death syndrome (SIDS), which will be discussed later.
Grogaard et al39 studied the cardiovascular reflexes produced by chemoreceptor stimulation in unanesthetized lambs to examine the influence of central mechanisms on them. Marked bradycardia, hypertension, increased systemic vascular resistance, and blood flow redistribution were noted with water infusion into the larynx. The associated apneic reflex was known to be associated with hypoxia and a decreased arterial oxygen tension, which should stimulate arterial chemoreceptors primarily in the carotid body. A decrease in the reflex cardiovascular response to laryngeal chemoreceptor stimulation during hyperoxia was demonstrated. This suggested that carotid body receptors operating through a central mechanism were modulators of the laryngeal chemoreflex response. Other workers demonstrated in newborn lambs a postnatal maturation effect on the carotid body–mediated hypoxic ventilatory response during the first 10 days after birth.40,41 It appears that the age-dependent magnitude of the chemoreflex response is related to the maturation of the modulating carotid bodies, which initiate the secondary central nervous system reflexes. The carotid bodies were shown to modify the respiratory and cardiovascular responses to laryngeal chemostimulation in 2- to 4-week-old lambs. Postnatal maturation of the carotid-body central respiratory reflex is required, as the reduced hypoxic ventilatory response noted in the first postnatal week of life was associated with an increased apneic reflex response. This observation was not altered by carotid body denervation.
Clinically, the laryngeal chemoreceptor reflexes discussed evolved as a protective mechanism to preserve vital functions when the organism was not breathing. The laryngeal chemoreflex has been implicated in the apnea associated with gastroesophageal reflux in infants42,43 and SIDS.30 As discussed, a striking feature of laryngeal chemoreflex apnea is its disappearance with age. In no species has a fatal or sustained apneic response been elicited in an adult animal or even an immature animal after a certain developmental period.30,44
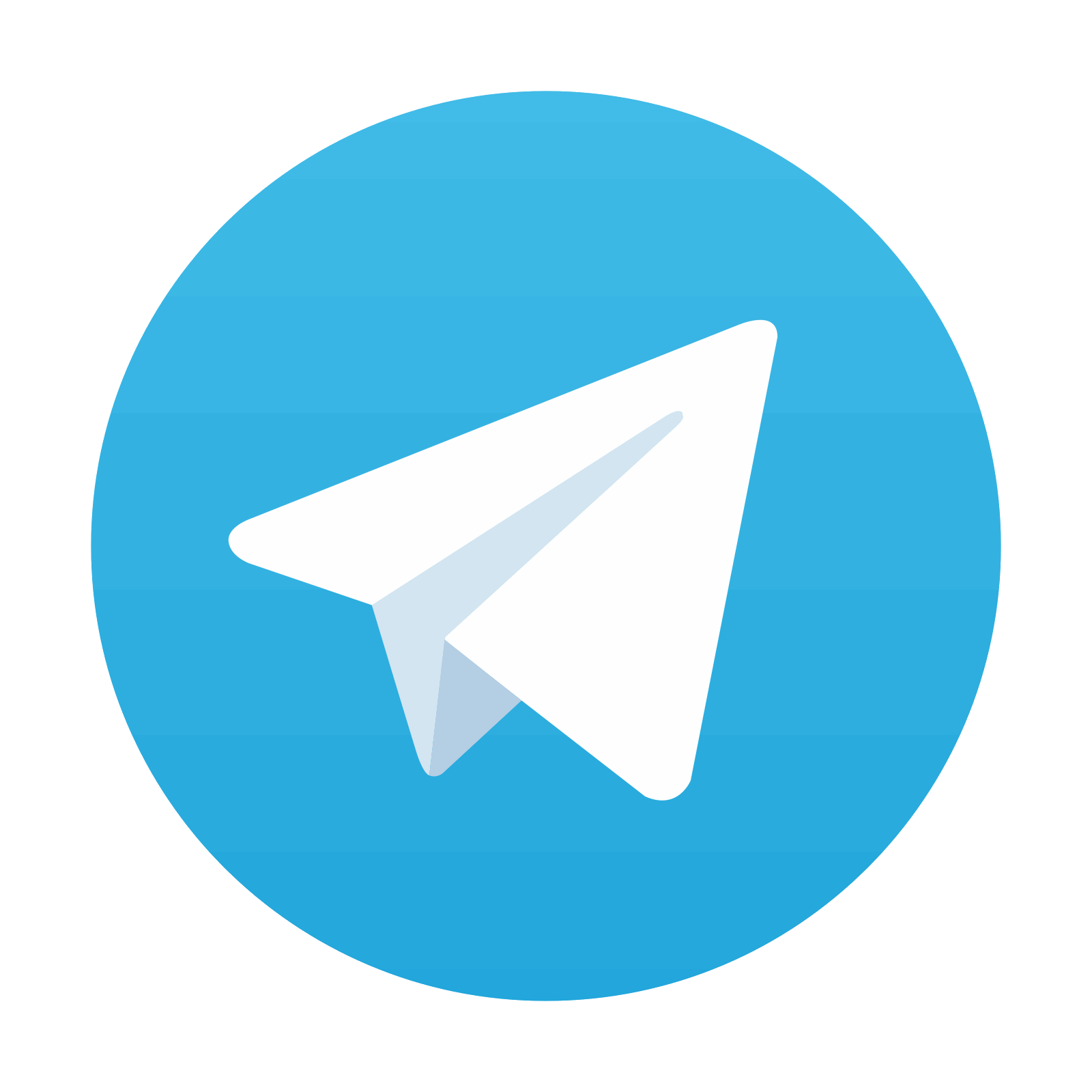
Stay updated, free articles. Join our Telegram channel

Full access? Get Clinical Tree
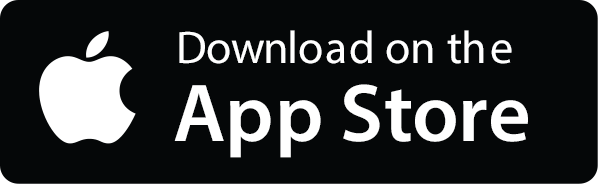
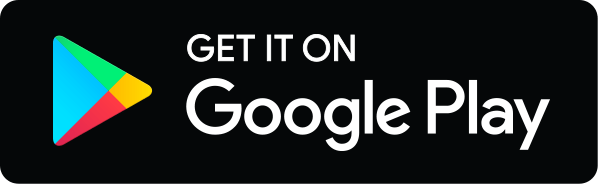