6 Movement and coordination
At the end of this chapter, you should be able to:
• describe the different types of movement, i.e. reflex and voluntary
• explain what is meant by the term spasticity and compare and contrast this with rigidity, hypertonia and contracture
• explain the role of the various brain areas, highlighted in the text, in movement
• describe the problems associated with cerebral palsy and the use of anti-spasmodic therapies to treat the motor symptoms of the condition.
Introduction
The contraction of the skeletal muscle involves the complex interaction of four specific proteins: actin, myosin, troponin and tropomyosin. Many individual skeletal muscle units (i.e. sarcomeres) fuse together to form the long fibres which have one end attached to the tendon, which is in turn attached to the skeleton. It is this connection that is responsible for muscle contraction resulting in skeletal movement. The structure of normal muscle can be seen in Figure 6.1.
Unlike cardiac and smooth muscle, which have regular contraction and relaxation patterns, skeletal muscle usually only contracts under the influence of neuronal stimulation. This stimulation occurs at the neuromuscular junction (Fig. 6.2), where the neurotransmitter involved is acetylcholine. The motor neurone releases acetylcholine which travels across the junction and interacts with nicotinic cholinergic receptors on the skeletal muscle fibres. This receptor stimulation results in the creation of a muscle action potential, brought about by the opening of voltage-gated sodium channels, which in turn causes the release of calcium from the sarcoplasmic reticulum (the intracellular store of calcium). Inside the skeletal muscle cell can be found a complex formed by two proteins, troponin and tropomyosin, which, on interaction, inhibits the interaction of the two contractile proteins, actin and myosin. The calcium which enters binds to the troponin and displaces the troponin–tropomyosin complex, thereby allowing the actin and myosin to interact. This leads to the contraction of the muscle via the sliding filament theory. As calcium levels fall, the troponin–tropomyosin complex is re-established, the actin–myosin interaction is again inhibited and the muscle relaxes (note that the muscle is only relaxed when neural transmission halts – it is unable to elongate (stretch) itself!).
Types of movement
Reflex movements
You will recall from Chapter 1 that reflex movements were first described by Charles Sherrington, the eminent British pathologist and neurophysiologist, at the turn of the 19th century. Reflex movements are those automatic movements that happen without us even thinking about them. Probably one of the best-known examples of a reflex movement is the knee jerk that follows a tap on the patellar tendon just below the knee. This is a relatively straightforward example of a reflex and comes about following the reactive contraction of the quadriceps femoris (thigh) muscle in response to the tap (which had caused it to stretch). A more complex example of a reflex movement can be seen when there is a coordinated muscle response, allowing us to maintain our balance following a large push, for example.
The basic structure of a reflex arc involves sensory input and motor output linked by small interneurones. The reflex movement initiated is very much dependent on the particular muscle mass stimulated. If we take the example of limb movements, contraction of the extensor muscles leads to extension of the limb, whereas contraction of the flexor muscles leads to withdrawal of the limb. It is the reflex withdrawal of our limbs and body that gives us a level of protection when we encounter potentially harmful stimuli, e.g. touching a hot surface. One other aspect of the reflex movement system is that there is also a level of variability of response within it. If your hand were to come into contact with a slight heat, producing a low level of pain, then withdrawal of the arm would be seen (Fig. 6.3). However, if the thermal stimulus was exceptionally hot, producing a high level of pain, then a withdrawal of the whole body would be seen. This variability in response can be achieved through so-called ‘triggered reactions’, with a latency of 80–120 ms. These reactions can result in complex responses, involving a number of muscle groups which may be relatively remote from the area stimulated originally. Note that we are still within the domain of reflexes; voluntary reaction time (the M3 response) takes longer with a latency of 120–180 ms, as various stages of information processing need to be dealt with (see Chapter 7).
In addition to this flexor reflex there is also the stretch reflex (Fig. 6.4), which occurs when, as the name implies, a muscle is stretched. The level of muscle activation observed can vary from individual to individual and can be altered under certain conditions, e.g. spinal injury, stroke, etc. The abnormal changes in muscle activation that are observed arise from a reduction in the level of neuronal impulses coming from the brain areas involved in movement. These efferent neuronal impulses are normally inhibitory and if they are removed, or reduced, a hyperexcitation of the α and γ motor neurones can be seen. This hyperexcitation may lead to muscle rigidity or spasticity.
Spasticity, rigidity, hypertonia and contracture
Spasticity is a common problem in neurological conditions and is considered as one component of the upper motor neurone (UMN) syndrome. The UMN syndrome is a constellation of symptoms that are traditionally divided into ‘positive’ and ‘negative’ (Barnes and Johnson 2001). Positive symptoms are associated with an exaggeration of motor activity, whereas negative symptoms arise from a lack of it (Table 6.1). A full discussion of positive symptoms of the UMN other than spasticity can be found in Sheean (1998, 2001).
Table 6.1 Examples of ‘positive’ and ‘negative’ symptoms of the upper motor neurone syndrome.
Upper motor neurone syndrome | |
---|---|
Positive features | Negative features |
Spasticity Rigidity Spastic dystonia Associated reaction Clasp-knife phenomenon Clonus Spasm Co-contraction Increased tendon reflexes Abnormal reflexes (e.g. Babinski) | Hypotonia Paresis Loss of dexterity Fatigueability |
(Based on Barnes & Johnson, 2001)
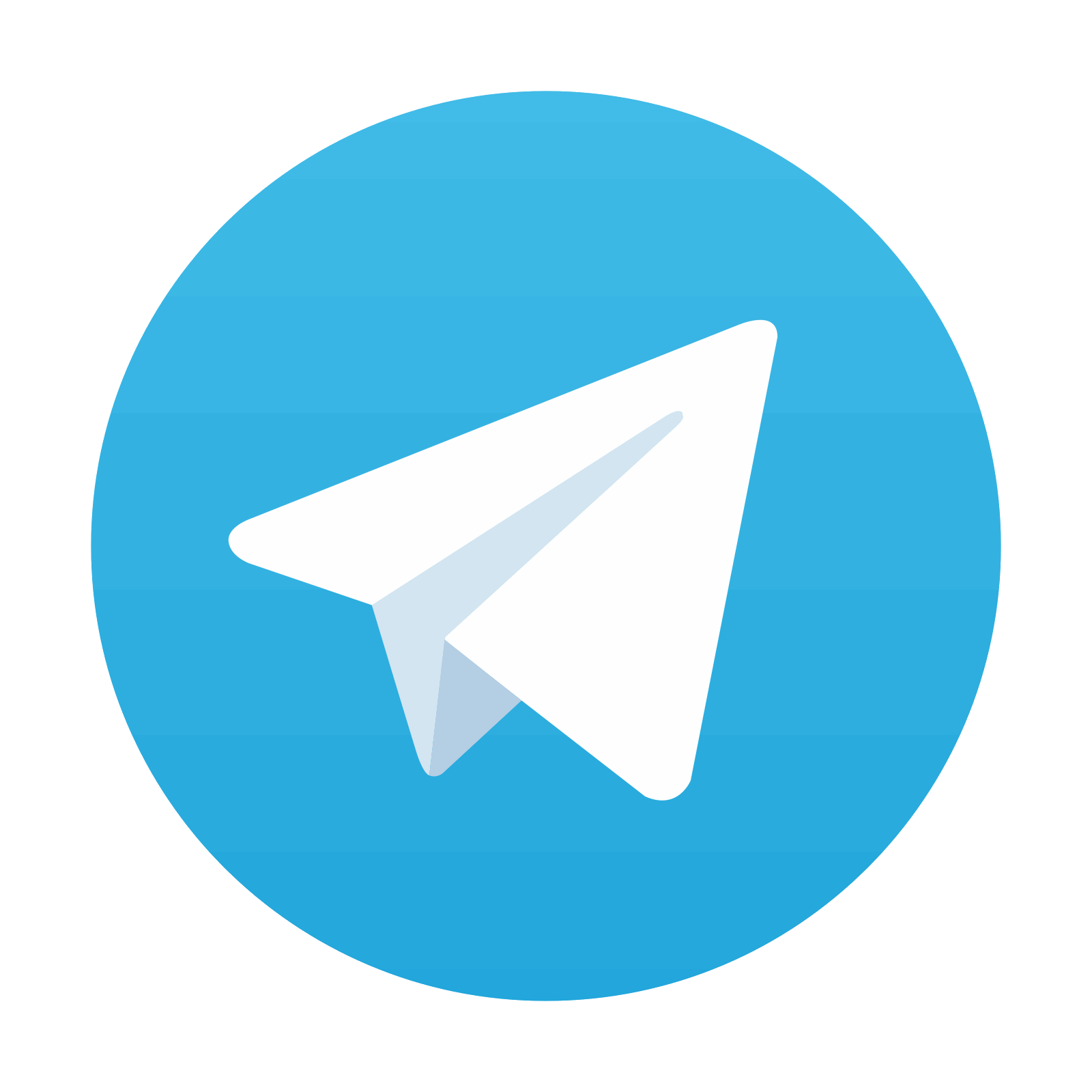
Stay updated, free articles. Join our Telegram channel

Full access? Get Clinical Tree
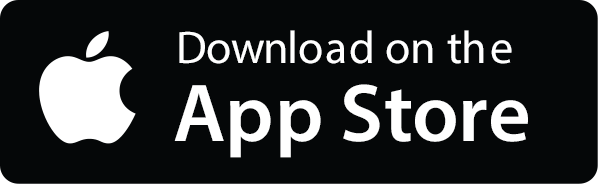
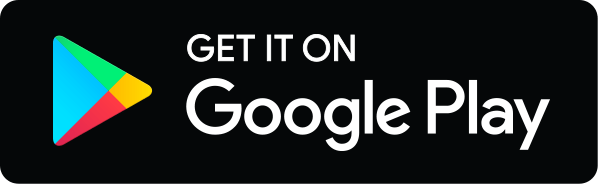