16 Myelomeningocele Fetal surgery was developed to treat conditions such as congenital diaphragmatic hernia and sacrococcygeal teratoma that were otherwise fatal.1 An open neural tube defect, however, is compatible with a term gestation, and a child with such a defect in the modern era should expect a long life span, particularly if concurrent medical problems are managed appropriately.2 As with any surgical intervention, the procedural risks must be balanced against the potential benefit, which in this case is a reduction in the burden of disability for the affected child. A particular ethical consideration specific for this fetal procedure is the risk borne by the mother, and whether she is able to dispassionately balance the risk to herself against the benefit to her unborn child. Currently, the reported benefits of fetal surgery include a reduction in shunt insertion rates and an improvement in the hindbrain abnormality.3–5 Published reports of results from fetal myelomeningocele procedures are mostly retrospective case series compared with historical controls. To obtain more conclusive data, the potential benefit of fetal surgery for myelomeningocele is being examined in a clinical trial directly comparing patients randomized into preand postnatal treatment groups. The neurologic deficits associated with a myelomeningocele can be separated into two groups: primary and secondary. Primary neurologic deficits are those caused by the arrested development of the neural tube.6 Because neural tube closure occurs during the 3rd and 4th week of gestation, the spinal cord is very immature at the stage when a myelomeningocele develops. It is clear that the normal anatomy of the spinal cord is severely disrupted at the level of the placode.7 The functional neurologic level is either at the same level as the vertebral anomaly or actually higher than the vertebral level, resulting in worse neurologic function in 80% of patients with spina bifida aperta, but not with spina bifida occulta.8 There is little that can be done in the postnatal setting to reverse the primary developmental abnormality of the affected spinal cord. It is unknown whether the unclosed neural tube retains the capacity for further development. The appearance of a repaired myelomeningocele lesion on magnetic resonance imaging (MRI) invariably shows a dysplastic spinal cord terminating and adherent to the over-lying soft tissues at the site of the defect. The presence of the conus at the level of the repair site means that virtually all of these patients have a tethered spinal cord by radiologic criteria. A symptomatic tethered spinal cord can occur in childhood or many decades later. Patients develop secondary neurologic deficits, such as loss of motor function, paresthesias, and worsening bowel and bladder control. Orthopedic problems, such as progressive foot deformities and scoliosis, can also occur. It is not clear why some patients with a myelomeningocele remain free of symptoms related to a tethered spinal cord. One possibility is that these patients are likely to maintain normal viscoelasticity of the filum terminale, preventing the lumbosacral cord from unnecessary stretching.9 The theoretical benefit of an early fetal myelomeningocele repair is that the neural tube is covered and protected many months before the anticipated delivery date. The basis for expecting improved neurologic function is that restoration of the dysplastic neural placode within the spinal canal isolates it from the amniotic fluid and prevents ongoing injury.10,11 Evidence supporting this hypothesis was obtained from experiments performed on fetal sheep. Mueli et al surgically created a spinal cord lesion in fetal sheep at 75 days of gestation that simulated a spontaneous spina bifida lesion.12 After delivery at term, these animals were incontinent and had loss of sensation and motor function below the lesion level. The gross and microscopic appearance of the exposed spinal cord resembled a human myelomeningocele lesion. Animals with surgically created spina bifida lesions were then treated using a musculocutaneous flap at 100 days of gestation. These animals were then carried to full-term gestation and had near-normal motor function and normal bowel and bladder control. The results of these experiments suggest that early repair of an exposed spinal cord may preserve neurologic function and allow improvement through plasticity.13 Although provocative and interesting, these large animal experiments clearly rely on a model system that does not recapitulate all the features of the human disease. Fetal surgery for myelomeningoceles would be expected to have the best results if performed as early as possible. However, this is limited by the timing of diagnosis and the technical limitations of the actual surgical procedure. Ultrasonography allows detection of most fetuses with myelomeningoceles by the midportion of the second trimester.14 From a practical point of view, this means that a diagnosis of a myelomeningocele is usually made between 18 and 22 weeks of gestation. Taking into consideration current obstetrical practice, it is unlikely that the detection of fetal myelomeningoceles will occur any earlier unless new, more sensitive screening tests are discovered. Preoperative fetal imaging studies begin with a detailed ultrasound examination. The specific goals of the exam are to identify anomalies in ventricular shape and size, the position of the cerebellar tonsils, the level of the spinal defect, and the presence of lower-extremity deformities. In many cases, the dimensions of the anatomical defect can be accurately measured by ultrasound examination, although there can be difficulty determining the exact dysraphic level.14 Most patients being considered for fetal surgery will also undergo an MRI study. The preferred technique performed at the University of California, San Francisco is a single-shot, fast spin echo T2-weighted sequence. The parameters of this sequence are repetition time, 4000 msec; echo time, 90 msec; field of view, 24 cm; slice thickness, 3 mm; no skip; bandwidth, 25 kHz; matrix, 192 160; and number of excitations, 0.5. These images are acquired in the axial, sagittal, and coronal planes, although adequate image quality is sometimes difficult to obtain because of fetal motion.15 There is some evidence that MRI may improve the ability to detect coexisting spinal and brain anomalies that may not be apparent on ultrasound studies.16,17 Fetal surgery cannot be performed without the participation of a well-trained team. Successful completion of a procedure requires specialized maternal and fetal anesthesia, the ability to perform uterine opening (hysterotomy) and closure with control of uterine contractions, and continuous intraoperative fetal monitoring. These techniques are described elsewhere and will not be addressed further in this chapter.18 The steps involved in the fetal procedure are similar to the standard postnatal procedure. They include (1) identification of the neural placode, (2) separation of the placode from the surrounding epithelium, (3) closure of the dura and overlying soft tissues, and (4) closure of the skin. There are several differences, however, between a fetal procedure and that done upon a term infant. First, all tissues during dissection and closure must be handled with great care. The neural placode is extremely fragile, and even limited manipulation leads to loss of tissue integrity. Although the nerve roots are able to withstand some handling, excessive tension causes avulsion. The dura is often insubstantial, transparent when mobilized, and has the physical characteristics of arachnoid in older children. The skin is able to handle surgical dissection, but excessive tension leads to tearing. A second limitation is the inability to place the fetus in a neutral position at all times during the procedure. The location of the hysterotomy is determined in part by the position of the fetus but also by the location of the placenta (Fig. 16.1). The orientation of the fetus can be confirmed prior to hysterotomy with intraoperative ultrasound, but it can be difficult to maintain the lumbar spine in a horizontal position, which interferes with the visualization of the lesion. Additional assistants are sometimes required to stabilize the fetus. The fetal neural placode is more easily distinguished from the surrounding arachnoid and skin than in the term infant. The edges of the placode are contiguous with the arachnoid, which is extremely thin and translucent. If the myelomeningocele sac is intact, the placode will be lifted upward away from the surface of the back. The epithelium of the skin usually does not reach the edge of the placode. The clear identification of the intervening arachnoid usually allows the placode to be divided from its attachments with sharp dissection. Depending on the consistency of the placode, the neural tube can be retubularized; however, if the placode is particularly fragile, this step may not be possible. The dura is loosely attached to the underlying subcutaneous tissues just lateral to the spinal canal. After incising the dura at its lateral junction with the dermis, gentle instillation of saline into the epidural plane lifts the dura away from the underlying tissues, which minimizes the manipulation of the dura. Between 18 and 20 weeks of gestation, the dura can be very thin and difficult to handle. After 22 weeks of gestation, the dura becomes more substantial and can be handled more easily. Fig. 16.1 Fetal closure of a myelomeningocele lesion. (A) The fetal myelomeningocele is framed in the hysterotomy incision. The fetus is not removed from the uterus, and every effort is made to maintain the amniotic fluid volume. The actual sac consists of thin membranous tissue with a minimum of skin extending upward toward the placode. (B) The skin is closed as a single layer, in this case without a patch required. (C) After delivery at 36 weeks, the wound appears well closed, although the sutures, which are the monofilament absorbable type, are still visible. Once the dura is detached from the dermis and separated from the underlying lumbar fascia, it can be closed using a running suture. If the amount of dura is insufficient, then a patch is used to close the opening. The use of acellular human dermis to repair the dura may contribute to the formation of intracellular dermoid cysts.19 For this reason, a synthetic collagen matrix (DuraGen, Integra Life Sciences, New Jersey) can be used to create a dural barrier. Following dural closure, the skin is closed as a single layer incorporating the superficial and deeper tissues. In general, dissection of the underlying muscle and fascia is not attempted because excessive fetal blood loss must be avoided and the duration of the procedure minimized. Elevation of the skin and separation from the underlying subcutaneous tissues are relatively easy, although increased tension on the skin inevitably leads to tearing. Small openings in the skin caused by handling with forceps or tension from suture points generally close rapidly. If the skin can be brought together, the final postnatal appearance is often excellent (Fig. 16.1). For situations where insufficient skin is available to close the lesion, skin flaps, relaxing incisions, or synthetic material can be used. There are substantial complications associated with open hysterotomy. These include placental separation, blood loss, premature labor, and delayed uterine rupture.20,21 All of these reasons have led to most fetal procedures evolving toward endoscopic techniques. The treatment goals for myelomeningocele can be achieved by using endoscopic or open procedures, but the quality of the repair is substantially better with an open procedure.22,23 Because endoscopic procedures are associated with fewer complications, new techniques may allow this approach to be reevaluated in the future. Data from centers performing fetal surgery for myelomeningocele have indicated that the benefits of surgery are a reduction in the rate of cerebrospinal fluid (CSF) shunt insertion and improvement in the appearance of the Chiari II malformation (Fig. 16.2) on imaging studies.3,24 It is unknown whether the structural improvement in the hindbrain abnormality results in an improvement of clinical signs and symptoms caused by the Chiari II malformation. The shunt rate in a cohort of 116 children treated with fetal surgery and followed in the postnatal period for at least 12 months was 54%.25 The strongest predictor for postnatal shunt placement was the upper level of the spinal lesion, with those above L3 showing the highest rates of shunt insertion. This trend is similar to a historical series where lesion level affected shunt rates.8 The overall percentage of patients requiring shunt placement, based on retrospective series, is usually in the range of 80 to 95%. By this measure, the reduction in shunt insertion rates reported in the fetal surgery group is encouraging. However, it is possible that selection bias alone may account for this benefit. Experimental evidence suggested that early closure of myelomeningoceles should improve neurologic function by preventing secondary injury to the exposed nervous tissue.10,12 Early clinical results from fetal repair of human myelomeningoceles have been disappointing. Tubbs et al examined a cohort of patients (n 37) who had undergone fetal repair between 20 and 28 weeks of gestation and compared their neurologic function to a cohort (n 40) of patients who underwent postnatal procedures.26 No statistical difference was observed in lower-extremity function between the two groups. Although this study, along with others, has limitations inherent with any retrospective analysis, the lack of clear improvement in neurologic function with fetal surgery suggests that the animal models used to study this disorder do not adequately recapitulate the human disease. The incidence of delayed signs and symptoms such as lower extremity weakness, worsening of bladder and bowel control, and pain in patients who have had fetal surgery is unknown. Based on anecdotal cases, reexploration in patients who have had previous fetal repair appears to be more difficult because tissue planes in the area of the placode are poorly defined. Postnatal imaging studies of the distal spinal cord in patients who have had fetal repair, however, do not show an obvious anatomical difference compared with patients who have had postnatal repair. Urodynamics performed on a small group of children who had undergone fetal surgery showed clear abnormalities such as vesicoureteral reflux and a significant postvoid residual urine volume. These results were indistinguishable from those of patients who had undergone postnatal repair.27 This is not unexpected, as urological function should be strongly related to sacral spinal cord function. Fig. 16.2 Effect of fetal closure on hindbrain appearance. (A) Fetal magnetic resonance imaging (MRI) sagittal image showing a tight posterior fossa with tonsillar descent into the upper cervical spine. (B) MRI (T1-weighted sagittal sequence) of the same patient after delivery showing the near normal appearance of the cerebellum with normal-appearing brainstem and the presence of a cisterna magna.
Fetal Repair
Neurologic Deficits and Myelomeningocele
Rationale for Fetal Repair of Myelomeningocele
Timing for Fetal Surgery
Preoperative Imaging Studies
Surgical Technique
Results
Conclusion
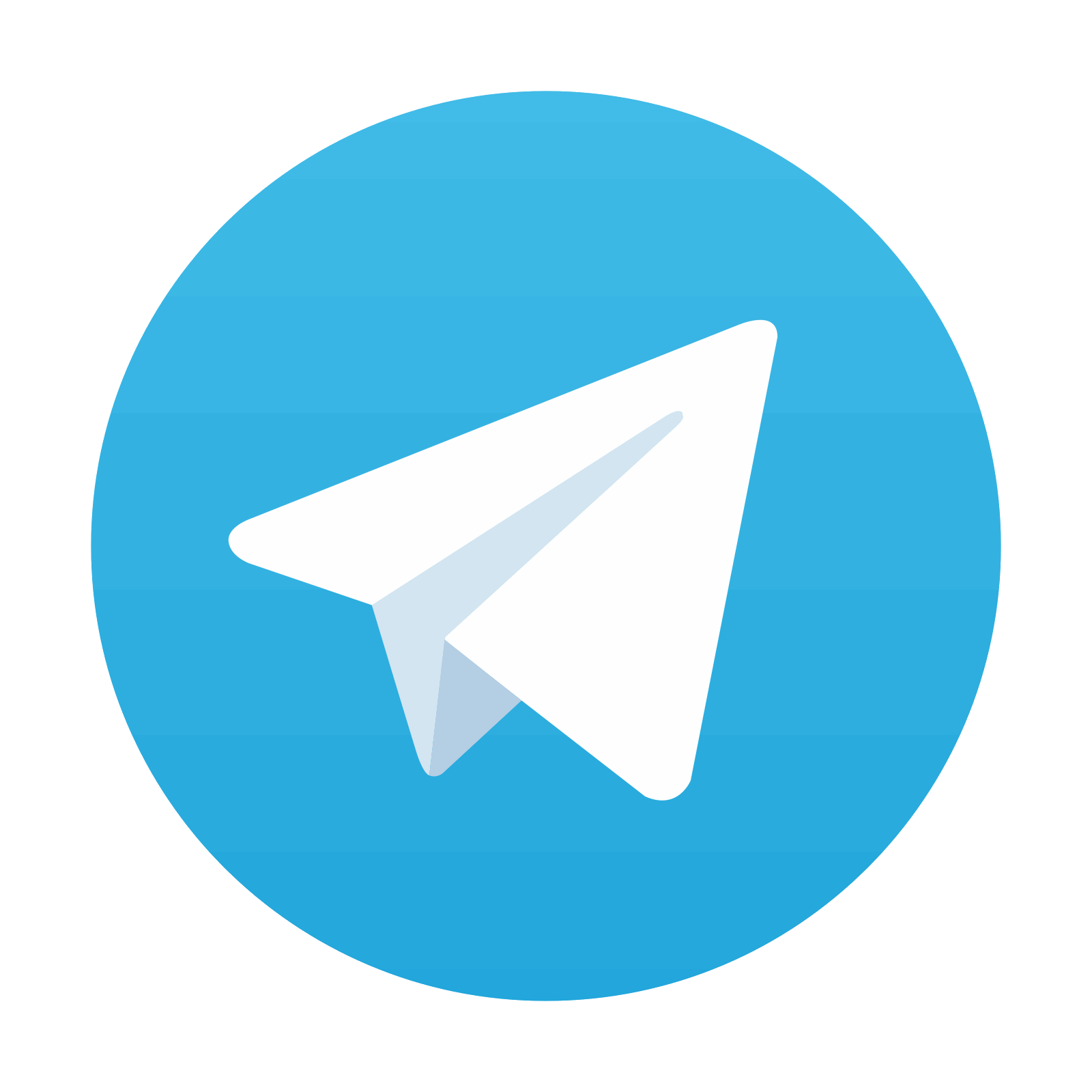
Stay updated, free articles. Join our Telegram channel

Full access? Get Clinical Tree
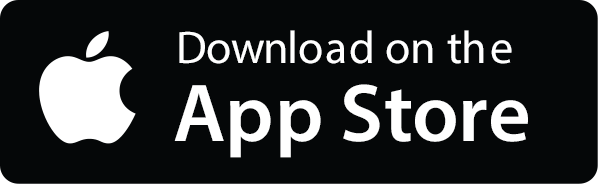
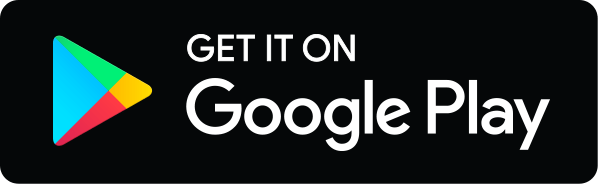