Abstract
Dry eye, a multifactorial disease of the tears and ocular surface, leads to tear film instability, potentially resulting in tear film hyperosmolarity, ocular surface epithelial damage, and inflammation. Impaired visual-related function associated with dry eye disease poses a significant economic and humanistic burden worldwide. Current treatment options are mostly palliative in nature, intended to supplement tears and alleviate dry eye symptoms. Neuromodulation is an established therapeutic strategy utilizing the direct activation of neural pathways to correct organ dysfunction and manage disease symptoms. A novel intranasal tear neurostimulation device has been developed, designed to increase tear production and improve tear quality in patients with dry eye by activating the nasolacrimal neuronal pathway through electrical stimulation of sensory nerve endings in the nasal mucosa. In clinical studies, the intranasal tear neurostimulator has demonstrated ability to increase tear production and improve symptoms, showing promise as a treatment option for patients with dry eye.
Keywords
Dry eye disease, Electrical stimulation, Intranasal tear neurostimulator, Nasolacrimal pathway, Neuromodulation, Ocular surface, Signs and symptoms, Tear film, Tear production, Transcutaneous electrical nerve stimulation
Outline
Overview of Dry Eye Disease 1235
Signs, Symptoms, and Diagnosis of Dry Eye 1235
Epidemiology of Dry Eye Disease 1236
Physiology of the Ocular Surface 1236
Pathophysiology of Dry Eye Disease 1238
Role of Inflammation in Dry Eye 1239
Anatomy and Physiology of the Lacrimal Functional Unit 1239
Oculolacrimal Neural Pathway 1239
Nasolacrimal Reflex Pathway 1240
Pathophysiology of the Lacrimal Functional Unit 1240
The Intranasal Tear Neurostimulator 1241
Clinical Outcomes With the Intranasal Tear Neurostimulator in Dry Eye 1242
Effects on Aqueous Tear Production 1243
Effects on Tear Components, Goblet Cell and Meibomian Gland Activity 1244
Safety of the Intranasal Tear Neurostimulator 1244
Conclusions 1244
Acknowledgments [CR]
References 1245
Acknowledgments
Writing and editorial assistance was provided to the authors by Andrew Fitton, PhD, and Kakuri M. Omari, PhD, of Evidence Scientific Solutions, Philadelphia, PA, USA, and was funded by Allergan plc.
Overview of Dry Eye Disease
Signs, Symptoms, and Diagnosis of Dry Eye
Dry eye, also known as keratoconjunctivitis sicca, is a multifactorial disease of the tears and ocular surface that is caused by reduced tear production and/or excessive tear evaporation. Common symptoms of dry eye include conjunctival redness, burning/stinging, itching, foreign body sensation, irritation, ocular fatigue, blurred vision, contact lens intolerance, excessive eye watering, and photophobia ( ). In addition to symptoms of ocular discomfort and visual disturbance, dry eye is associated with a loss of homeostasis of the tear film, in which tear film instability and hyperosmolarity, ocular surface inflammation and damage, and neurosensory abnormalities play etiological roles ( ).
Dry eye is usually a symptomatic disorder, ranging in severity from mild ocular irritation or discomfort to significant vision loss ( ). In the majority of patients, the condition is characterized by episodic irritative symptoms that are often triggered or exacerbated by aggravating factors such as environmental conditions that increase evaporative tear loss (e.g., exposure to low humidity, air conditioning, or high winds) ( ). In other patients, dry eye disease may be chronic, marked by continuous symptoms of fluctuating severity and possible damage to the ocular surface. Inflammation associated with dry eye disease can lead to various ocular complications, including infection, ocular surface keratinization and vascularization, corneal ulceration and opacification, and conjunctival squamous metaplasia ( ).
The symptoms of dry eye disease can adversely affect the performance of everyday vision-related activities such as reading, driving, computer use, and watching TV ( ). Manifestations of ocular pain and irritation, and impairment of vision-related function associated with dry eye disease have significant negative impact on patients’ quality of life ( ). Dry eye disease is one of the leading causes of patient visits to ophthalmologists and optometrists ( ). There is currently no cure for dry eye. The most frequently used treatments are artificial tears and anti-inflammatory agents ( ). Artificial tears often contain polymers that lubricate the ocular surface, but lack the numerous biological factors found in natural tears that maintain ocular surface homeostasis ( ). In addition, preservatives in multidose formulations of artificial tears may irritate the ocular surface ( ). Thus, an opportunity exists for development of novel therapies with different mechanisms of action that can trigger production of natural tears in patients with dry eye.
Diagnosis of dry eye disease is based on the presence of symptoms in combination with one or more objective clinical signs of reduced tear flow, tear film instability, and ocular surface damage ( ). Supplementary diagnostic procedures that are used where available include measurement of tear film osmolarity, tear lactoferrin levels, and impression/brush cytology, and other more sophisticated laboratory tests such as fluorescein tear clearance measurements, lacrimal gland function tests, meibography, and tear fluid protein immunoassay ( ). However, diagnosis of dry eye disease is complicated by the poor correlation between clinical signs and reported symptoms, the absence of a single definitive diagnostic test, and the lack of consensus on which combination of tests should be used ( ). Because of these limitations, dry eye disease is presumed to be frequently misdiagnosed and under-reported.
Epidemiology of Dry Eye Disease
Estimates of the prevalence of dry eye symptoms in the general population vary widely, ranging from 5% to 50%, depending on the population studied, the geographic location, and how dry eye is defined ( ). In the United States, the prevalence of self-reported dry eye in the Beaver Dam (Wisconsin) Eye Study (n = 3722) varied from 8.4% in subjects under 60 years of age to 19.0% in those over 80 years of age; the overall prevalence was 14.4% ( ). The Men’s Health Study (n = 25,444) indicated that the prevalence of dry eye (defined as a reported clinical diagnosis or either frequent or constant symptoms of dryness and irritation) was 3.9% in men in the 50–54-year age group, rising to 7.7% in those over 80 years of age ( ). Using the same definition of dry eye, the Women’s Health Study (n = 39,876) reported a prevalence of 5.7% in women under 50 years of age, rising to 9.8% in those over 75 years of age ( ). In the United States, it had been estimated that 3.2 million women and 1.7 million men over the age of 50 years have moderate to severe dry eye ( ), and tens of millions more experience other forms of dry eye ( ).
Established risk factors for dry eye include advanced age and female gender ( ), postmenopausal estrogen therapy ( ), androgen deficiency ( ), a diet low in omega-3 essential fatty acids or with a high omega-6 to omega-3 fatty acid ratio ( ), vitamin A deficiency ( ), contact lens use ( ), prolonged computer use ( ), low humidity environments (e.g., air-conditioned offices, aircraft cabins) ( ), refractive surgery ( ), bone marrow transplantation ( ), hepatitis C ( ), and certain classes of systemic medications, including antidepressants, anxiolytics, and antihistamines ( ). The prevalence of dry eye is likely to continue to grow with the increase of risk factors such as an aging population, a greater number of refractive laser surgeries, and more frequent use of contact lenses, computers, smart phones, and tablets.
Physiology of the Ocular Surface
Tears
The ocular surface, which comprises the cornea and the bulbar and tarsal conjunctiva up to the mucocutaneous junctions of the lid margin, is continuously bathed by tears. Components of the tear are secreted by the lacrimal and meibomian glands, with additional contributions from the conjunctiva ( ) ( Fig. 102.1 ). The lacrimal glands are exocrine glands located beneath the superior temporal lid and orbital rim (main lacrimal gland) and on the inner surface of the lids and conjunctival fornices (accessory lacrimal glands of Krause and Wolfring) that secrete aqueous fluid into the upper and outer conjunctival fornices ( ). The lacrimal secretion, comprising water, electrolytes and proteins, including glycoproteins such as mucins (MUC1, MUC4, MUC7, MUC5AC) from the acinar cells of the lacrimal glands or tear duct ( ), is responsible for the bulk of the tear volume and flow ( ). Conjunctival goblet cells also produce the gel mucin component (MUC5AC), which acts as an eyelid lubricant ( ).

The meibomian glands are tubuloacinar sebaceous glands lying in parallel rows in the tarsal plates of the upper and lower eyelids, with duct orifices opening onto the eyelid margins ( ). The meibomian secretion (meibum) in its healthy form is a clear oily product, consisting of a complex mixture of lipids, that is expressed into a shallow, eyelid margin reservoir, from where it spreads onto the surface of the tear film with each blink ( ).
Meibum secretion is achieved by the coordinated activity of Riolan’s muscle, a sphincter muscle at the orifice of the meibomian glands on the surface of the eyelid, and the orbicularis oculi muscle that runs alongside the eyelids and provides pressure to the meibomian glands when contracted ( ). When the eyelids are open, Riolan’s muscle is contracted, which closes the orifice of each meibomian gland, thereby minimizing environmental influences such as bacterial entry into the meibomian gland; at the same time, the orbicularis oculi muscle is relaxed and thus exerts minimal pressure on the meibomian glands. During blinking or when meibum needs to be expressed, orbicularis oculi muscle action increases the pressure and temperature inside the meibomian glands, while relaxation of Riolan’s muscle allows the meibum to exit the gland onto the eyelid margin. Subsequent blinking helps to mix and distribute the meibum with the tear film across the eye.
Tear Film
The preocular tear film is a vital layer of fluid approximately 3 μm thick that covers the entire ocular surface ( ). It consists of three identified but dynamically interacting layers: a superficial lipid layer, an intermediary aqueous layer, and a deep mucin layer on the epithelial surface ( ) ( Fig. 102.1 ). There appears to be no strict segregation of tear layer components, with the aqueous and mucin layers combining to form a hydrated mucin gel, and with lipid associating with proteins throughout the gel ( ). A healthy tear film requires that all components be present in appropriate concentrations, and deficits in any layer can disrupt the function of the tear film and cause dry eye symptoms ( ).
Lipid Layer
The lipid layer is derived from meibum that is spread from the eyelid margins with each blink. Its deepest aspect consists of polar lipids and long-chain fatty acids, which interface with the aqueous layer of the tear film, while its upper (and much thicker) aspect is formed mostly of highly nonpolar lipids, such as wax esters and cholesteryl esters ( ). Glycoproteins such as lipocalins, lysozyme, and mucin are believed to be intercalated with the lipid layer and enhance its stability ( ). This protective lipid layer plays a key role in stabilizing the tear film and slowing evaporation of the underlying aqueous layer ( ).
Aqueous Layer
The aqueous layer forms the bulk (∼90%) of the tear film and contains electrolytes and a large range of proteins, including growth factors (e.g., epidermal growth factor and hepatocyte growth factor) important for maintenance of the epithelium, antimicrobial peptides related to innate immunity (e.g., lysozyme, lactoferrin, surfactant protein-D, and trefoil peptide), and immunoglobulins involved in adaptive immunity (e.g., IgA) ( ). The aqueous layer hydrates the mucin layer, supplies oxygen and electrolytes to the conjunctival and corneal epithelium, provides antibacterial defense, maintains and renews the ocular surface, and washes away invading debris, such as dust, pollen and other air pollutants ( ).
Mucin Layer
The goblet cells of the conjunctival epithelium secrete into the tears a gel-forming mucin (MUC5AC), a highly glycosylated protein, which creates a smooth hydrophilic medium for wetting and protection of the ocular surface from dehydration ( ). Adhesion of the mucin layer to the ocular surface is achieved through interaction of mucin with minute membrane folds (microplicae) on the corneal and conjunctival epithelial surface. These membrane-tethered mucins form a layer (the glycocalyx) that protects the epithelium against microtrauma and infection ( ). The mucin layer lubricates and nourishes the ocular surface, and is critical in maintaining an optically smooth layering of the tear film for high-quality optical imaging ( ). Additionally, the mucin layer traps shed epithelial cells, inflammatory cells, and cellular debris to form a mucous thread within the lower conjunctival sac ( ) ( Table 102.1 ).
Tear Film | Source | Function |
---|---|---|
Lipid layer | Meibomian glands | Minimizes evaporation and maintains tear film stability |
Aqueous layer | Lacrimal glands | Lubricates the eye, washes away particles, and prevents infection |
Mucin layer | Epithelial goblet cells of the sclera and eyelid | Nourishes the cornea, maintains tear film adherence, and prevents microtrauma |
Tear Dynamics
Blinking plays a critical role in tear and tear film dynamics by mixing and spreading the tears and constantly refreshing the tear film. Although its key function is to prevent dehydration of the ocular surface, blinking also contributes to the mechanism of meibum delivery, tear drainage, and removal of debris from the ocular surface. Secreted tear fluid is distributed to and mixed with the tear film with each blink, and is subsequently lost through evaporation from the exposed tear film during the interblink interval and by drainage from the conjunctival sac via the nasolacrimal passage into the nasal cavity ( ).
Normally, tear dynamics are tightly regulated to maintain a stable tear film. The blink interval is an important determinant of evaporative water loss from the ocular surface, and hence of tear osmolarity ( ), which is normally maintained within narrow limits (mean 302 ± 9.7 mOsm/L) ( ). In healthy eyes, the tear film remains intact for 10 s or longer after each blink (as measured by the tear break-up time) ( ), which generally exceeds the normal blink interval (∼6 s under standard conditions of temperature and humidity) ( ), indicating that the eye is protected from dehydration during the blink cycle ( ). However, environmental stress from wind, low ambient humidity, and decreased blinking that occurs in tasks requiring visual concentration such as reading and computer work, increases tear evaporation and may potentially lead to loss of the tears’ homeostatic balance. Variation in tear film thickness and tear film break-up may give rise to visual disturbances, including blurred and fluctuating vision, decreased contrast sensitivity, and increased forward scatter and glare ( ).
Pathophysiology of Dry Eye Disease
Dry eye may develop from an abnormality in any of the three tear film layers, or from ocular inflammation, unequal distribution of the tear film caused by corneal scarring or surface irregularities, or from blinking abnormalities ( ). Dry eye may also be caused by damage to or destruction of the lacrimal tissues or obstruction of the secretion ducts, and may develop as a side effect of corneal or cataract surgery ( ). Generally, all the intrinsic and extrinsic factors that trigger or exacerbate dry eye cause disruption of the structure or function of one or more of layers of the tear film, resulting in tear film instability. The most common causal mechanism of tear film disruption is reduction of the aqueous layer, either through decreased tear production by the lacrimal glands or increased evaporative loss as a consequence of dysfunction of the mucin and lipid layers. Inflammation is also thought to contribute to tear film disruption by disrupting the function of one or more of the tissues that contribute to the tear film.
Reduced lacrimal tear flow can occur as a consequence of normal aging, inflammatory lacrimal damage, autoimmune disorders such as Sjögren’s syndrome, conjunctival scarring, or the use of certain medications, such as antihistamines and antimuscarinic agents. Increased tear film evaporation may be triggered by adverse environmental conditions such as low humidity and high air flow, and, in particular, by meibomian gland dysfunction, which leads to an unstable tear film lipid layer ( ).
Role of Inflammation in Dry Eye
In addition to aqueous tear deficiency and excessive evaporation, ocular surface inflammation is a major factor contributing to the pathogenesis of dry eye and ocular surface damage ( ). Exposure of ocular surface epithelial cells to the hyperosmolar tear environment promotes epithelial inflammation and damage through activation of intracellular mitogen-activated protein kinase (MAPK) signaling pathways in the ocular surface epithelium ( ). Osmotic stress, acting through the MAPK pathways, specifically stimulates production of inflammatory cytokines (e.g., interleukin [IL]-1α and 1β, IL-6 and tissue necrosis factor [TNF]-α), chemokines (e.g., CXC chemokine IL-8) and matrix metalloproteinases (MMPs; mainly MMP-9) by the corneal, conjunctival and glandular epithelial cells ( ). These proinflammatory mediators in turn activate antigen-presenting cells, leading to the recruitment to the ocular surface of autoreactive CD4+ helper T cells expressing immune activation and adhesion markers (e.g., HLA-DR, CD11a, and ICAM-1) ( ).
The inflammatory process is amplified through additional secretion of proinflammatory cytokines and MMPs by infiltrating CD4+ T cells, and may develop into a self-sustaining cycle of inflammation and ocular surface damage ( ). Infiltration of pathogenic CD4+ T cells (subtypes T H 1 and T H 17) triggers, via the secretion of interferon (IFN)-α and IL-17, apoptosis of surface epithelial cells, including goblet cells, and conjunctival epithelial metaplasia ( ). In addition, enzymatic degradation by MMPs of the corneal epithelial basement membrane and tight junction proteins undermines corneal epithelial barrier function ( ). The increased osmotic gradient between the tear film and the ocular surface draws water across conjunctival and corneal epithelial cells, causing epithelial desquamation, denudation of surface glycoproteins, and destabilization of the ocular surface–tear film interface.
Ocular surface inflammation can also be initiated by chronic irritative stress (e.g., contact lens use) and by systemic inflammatory or autoimmune disease (e.g., rheumatoid arthritis) ( ). These inflammatory, osmotic, and mechanical stresses ultimately lead to tear film instability, which in turn further exacerbates ocular surface hyperosmolarity ( ).
Anatomy and Physiology of the Lacrimal Functional Unit
The ocular surface (cornea and conjunctiva), lacrimal glands, meibomian glands, eyelids, nasolacrimal duct, and interconnecting afferent sensory and efferent autonomic and motor nerves as well as muscles of the eyelid comprise the lacrimal functional unit ( Fig. 102.2 ), an integrated homeostatic mechanism that maintains a healthy ocular surface environment ( ). Through this integrated functional unit, environmental, mechanical, chemical, or pathogenic stress to the ocular surface initiates reflex neural signaling that results in aqueous tear secretion and, possibly, stimulation of mucin and lipid secretion ( ).

Oculolacrimal Neural Pathway
The ocular surface is densely innervated, with the cornea, the main sensory epithelial surface of the lacrimal functional unit, having approximately 7000 nerve endings per square millimeter ( ). Corneal sensitivity is extremely acute and interpreted predominantly as irritation and pain ( ; ), whereas the conjunctiva is less densely innervated and less sensitive ( ). Sensory (afferent) fibers arising from the cornea and conjunctiva run along the ophthalmic branch of the trigeminal nerve (cranial nerve CN V) via the trigeminal ganglion and spinal trigeminal nucleus (located in the brainstem) to the superior salivatory nucleus in the midbrain (pons), where the signal is integrated with cortical and other neural inputs. The efferent loop descends via the facial nerve (CN VII) to the pterygopalatine ganglion, located next to the orbit, from where unmyelinated postganglionic parasympathetic fibers extend to the lacrimal gland, nasopharynx, and blood vessels of the orbit ( Fig. 102.2 ).
Parasympathetic fibers innervating the meibomian glands and other structures within the eyelid margin as well as conjunctival goblet cells, are also believed to follow this route ( ). Efferent innervation to goblet cells of the conjunctiva is provided by the oculomotor nerve (CN III), while efferent drive to the eyelid goblet cells is provided by CN VII. Likewise, Riolan’s muscle and the orbicularis oculi muscle receive efferent input through the facial nerve. The blink reflex utilizes the same neural pathways via trigeminal afferents and the somatic efferent motor fibers of the facial nerve ( ). Additionally, sympathetic fibers extend along the paravertebral sympathetic tract via the superior cervical ganglion to innervate the epithelia and vasculature of the ocular surface, lacrimal glands, meibomian glands, and nasolacrimal duct. This innervation pattern offers the possibility of coordinated secretion of the various tear film components.
An important role of the lacrimal functional unit is maintenance of basal (resting) tear flow. Basal tear flow is believed to result from continuous background stimulation of the corneal surface in response to environmental factors ( ). Various sensory stimuli, such as pain, microbial, environmental insult, emotion, and foreign body sensation can stimulate the lacrimal reflex to generate additional tear flow ( ). In contrast, inhibition of afferent sensory input with general anesthesia disrupts the lacrimal functional unit and blocks normal tear production ( ).
Nasolacrimal Reflex Pathway
A functionally related neural pathway linking the nasal mucosa to the lacrimal glands, the nasolacrimal reflex pathway, is an important contributor to basal and stimulated tear production ( ). The nasolacrimal reflex pathway comprises afferent nerve fibers running from the nasal cavity via the ophthalmic branch of the trigeminal nerve to the superior salivary nucleus, and linking with efferent parasympathetic fibers running, via the facial nerve and geniculate and pterygopalatine ganglia, to the lacrimal glands ( ) ( Fig. 102.3 ).

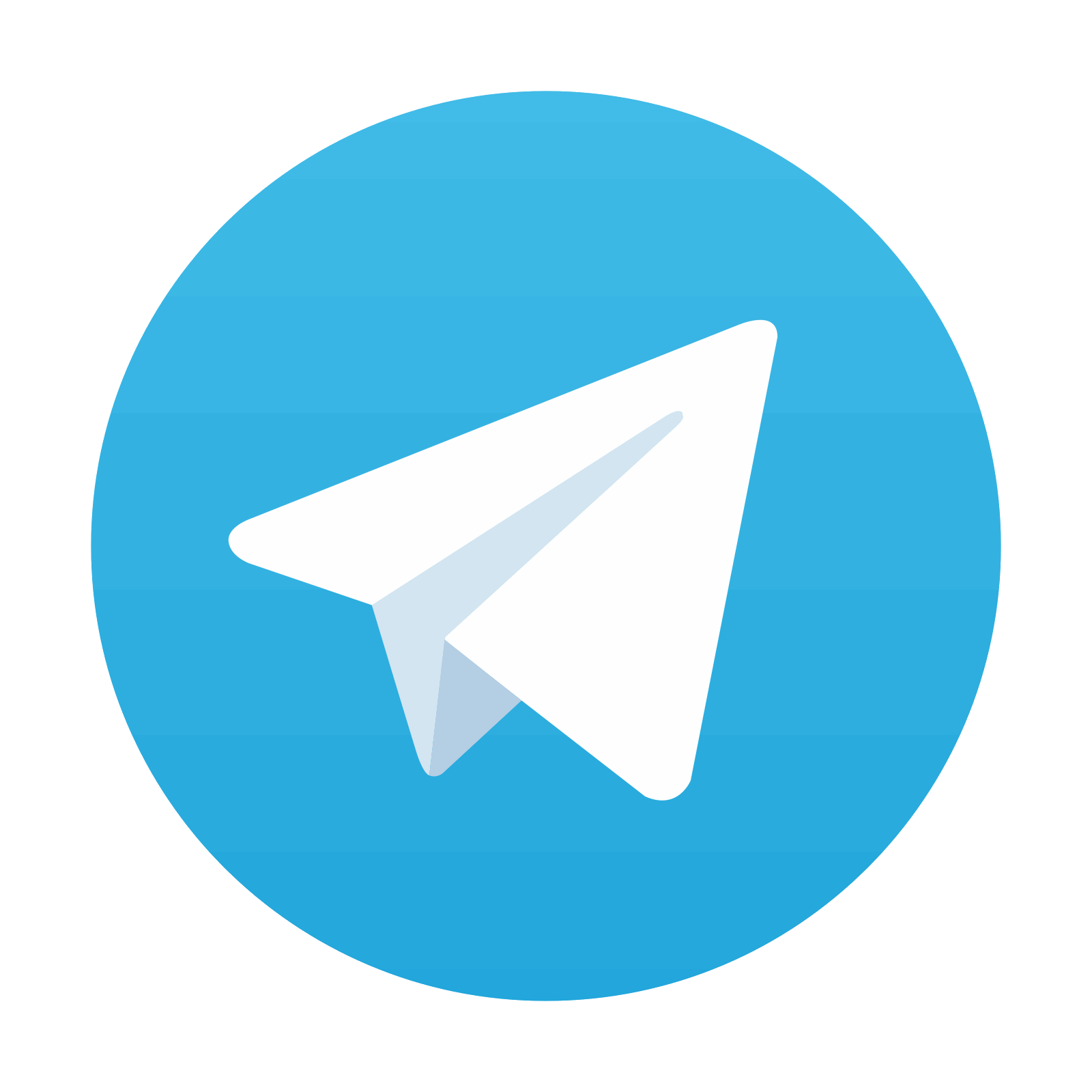
Stay updated, free articles. Join our Telegram channel

Full access? Get Clinical Tree
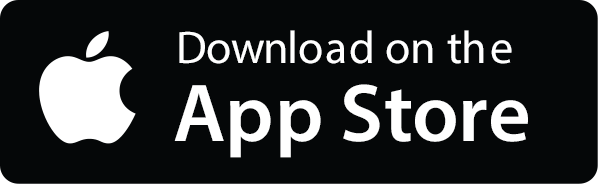
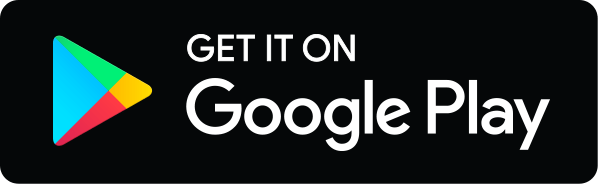
