Chapter 22 Neuromuscular Junction
Skeletal Muscle
The most intensively studied effector endings are those that innervate muscle, particularly skeletal muscle. All neuromuscular (myoneural) junctions are axon terminals of somatic motor neurones. They are specialized for the release of neurotransmitter onto the sarcolemma of skeletal muscle fibres, causing a change in their electrical state that leads to contraction. Each axon branches near its terminal and subsequently innervates from several to hundreds of muscle fibres, depending on the precision of motor control required. The detailed structure of a motor terminal varies with the type of muscle innervated. Two major endings are recognized: those typical of extrafusal muscle fibres, and endings on the intrafusal fibres of neuromuscular spindles. In the former, each axon terminal usually ends midway along a muscle fibre in a discoidal motor end-plate (Figs 22.1–22.3). This type usually initiates action potentials, which are rapidly conducted to all parts of the muscle fibre. In the latter, the axon has numerous subsidiary branches that form a cluster of small expansions extending along the muscle fibre. In the absence of propagated muscle excitation, these excite the fibre at several points. Both types are associated with a specialized receptive region of the muscle fibre, the sole plate, where a number of muscle cell nuclei are grouped within the granular sarcoplasm.
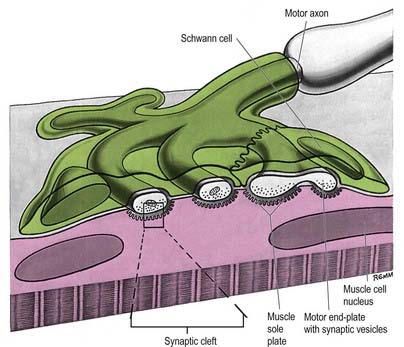
Fig. 22.2 Neuromuscular junction. Note the axonal motor end-plate and the deeply infolded sarcolemma.
The sole plate contains numerous mitochondria, endoplasmic reticulum and Golgi complexes (see Figs 22.2, 22.3). The neuronal terminal branches are plugged into shallow grooves in the surface of the sole plate (primary clefts), from which numerous pleats extend for a short distance into the underlying sarcoplasm (secondary clefts). The axon terminal contains mitochondria and many clear 60-nm spherical vesicles, similar to those in presynaptic boutons, clustered over the zone of membrane apposition. The motor terminal is ensheathed by Schwann cells whose cytoplasmic projections extend into the synaptic cleft. The plasma membranes of the nerve terminal and the muscle cell are separated by a 30- to 50-nm gap, with a basal lamina interposed. The basal lamina follows the surface folding of the sole plate membrane into the secondary clefts. It contains specialized components, including specific isoforms of type IV collagen and laminin and agrin, a heparan sulphate proteoglycan. Endings of fast and slow twitch muscle fibres differ in detail: the sarcolemmal grooves are deeper, and the presynaptic vesicles more numerous, in the fast fibres.
Conduction of the Nervous Impulse
All cells generate a steady electrochemical potential across their plasma membranes (a membrane potential) because of the different ionic concentrations inside and outside the cell (Fig. 22.4). Neurones use minute fluctuations in this potential to receive, conduct and transmit information across their surfaces.
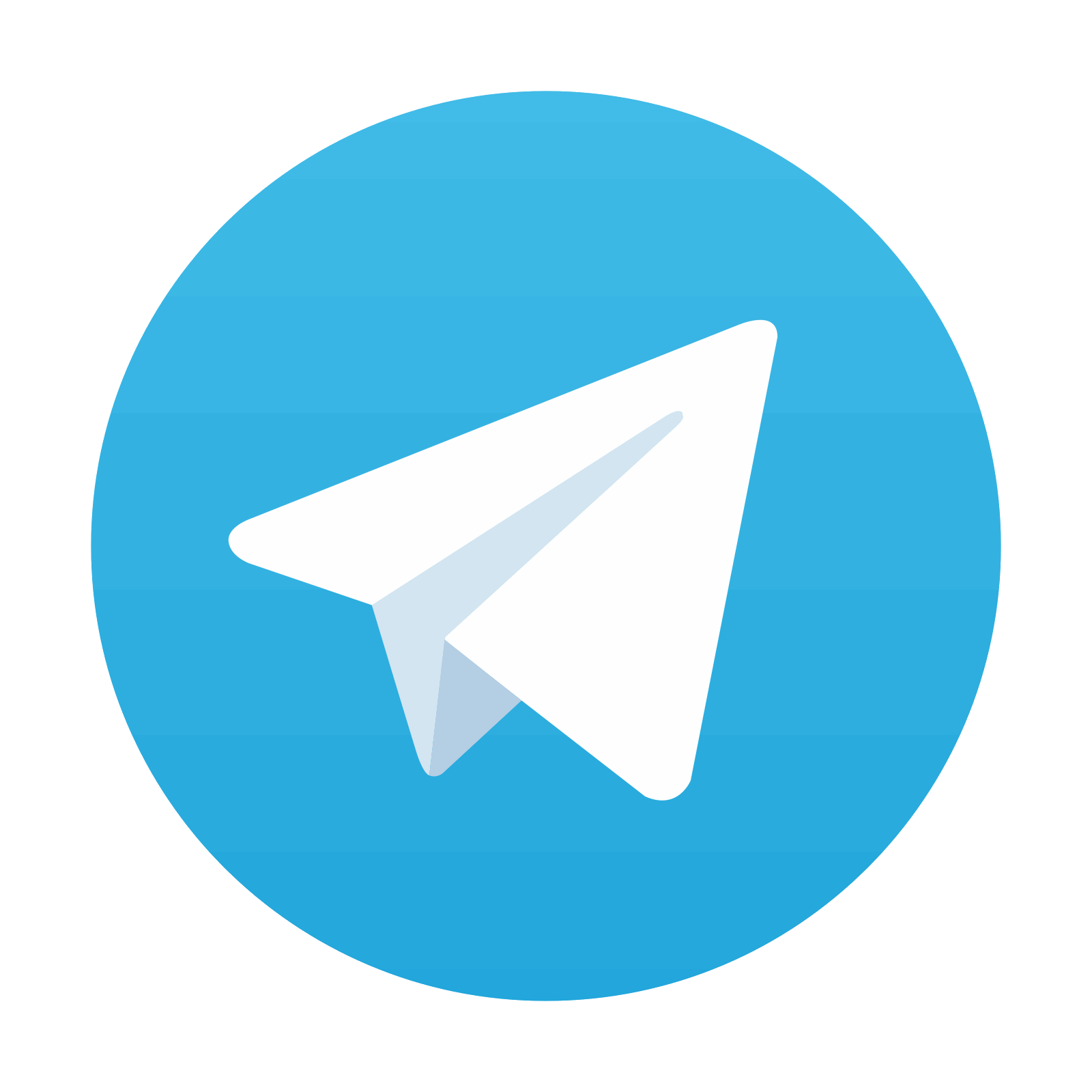
Stay updated, free articles. Join our Telegram channel

Full access? Get Clinical Tree
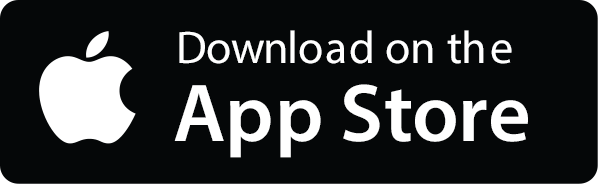
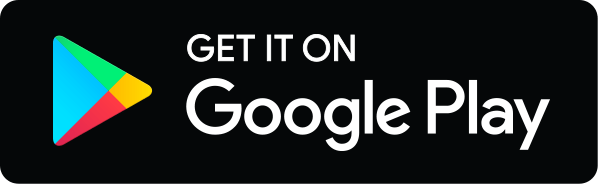