6 Neurons and neuroglia
Overview
Neurons
In the central nervous system (CNS), almost all neurons are multipolar, their cell bodies or somas having multiple poles or angular points. At every pole but one, a dendrite emerges and divides repeatedly (Figure 6.1). On some neurons, the shafts of the dendrites are smooth. On others, the shafts show numerous short spines (Figure 6.2). The dendrites receive synaptic contacts from other neurons, from some on the spines and from others on the shafts.
The remaining pole of the soma gives rise to the axon, which conducts nerve impulses. Most axons give off collateral branches (Figure 6.3). Terminal branches synapse on target neurons.
Internal structure of neurons
All parts of neurons are permeated by microtubules and neurofilaments (Figure 6.4). The soma contains the nucleus and the cytoplasm or perikaryon (Gr.’around the nucleus’). The perikaryon contains clumps of granular endoplasmic reticulum known as Nissl bodies (Figure 6.5), also Golgi complexes, free ribosomes, mitochondria, and smooth endoplasmic reticulum (Figure 6.4).
Transport mechanisms
Microtubules are the supporting structures for neuronal transport. Microtubule-binding proteins, in the form of ATPases, propel organelles and molecules along the outer surface of the microtubules. Distinct ATPases are used for anterograde and retrograde work. Retrograde transport of signaling endosomes is performed by the dynein ATPase. Failure of dynein performance has been linked to motor neuron disease, described in Chapter 16.
Some points of clinical relevance are highlighted in Clinical Panel 6.1.
Clinical Panel 6.1 Clinical relevance of neuronal transport
Tetanus
Wounds contaminated by soil or street dust may contain Clostridium tetani. The toxin produced by this organism binds to the plasma membrane of nerve endings, is taken up by endocytosis, and is carried to the spinal cord by retrograde transport. Other neurons upstream take in the toxin by endocytosis – notably Renshaw cells (Ch. 15), which normally exert a braking action on motor neurons through the release of an inhibitory transmitter substance, glycine. Tetanus toxin prevents the release of glycine. As a result, motor neurons go out of control, particularly those supplying the muscles of the face, jaws, and spine. These muscles exhibit prolonged, agonizing spasms. About half of the patients who show these classic signs of tetanus die of exhaustion within a few days. Tetanus is entirely preventable by appropriate and timely immunization.
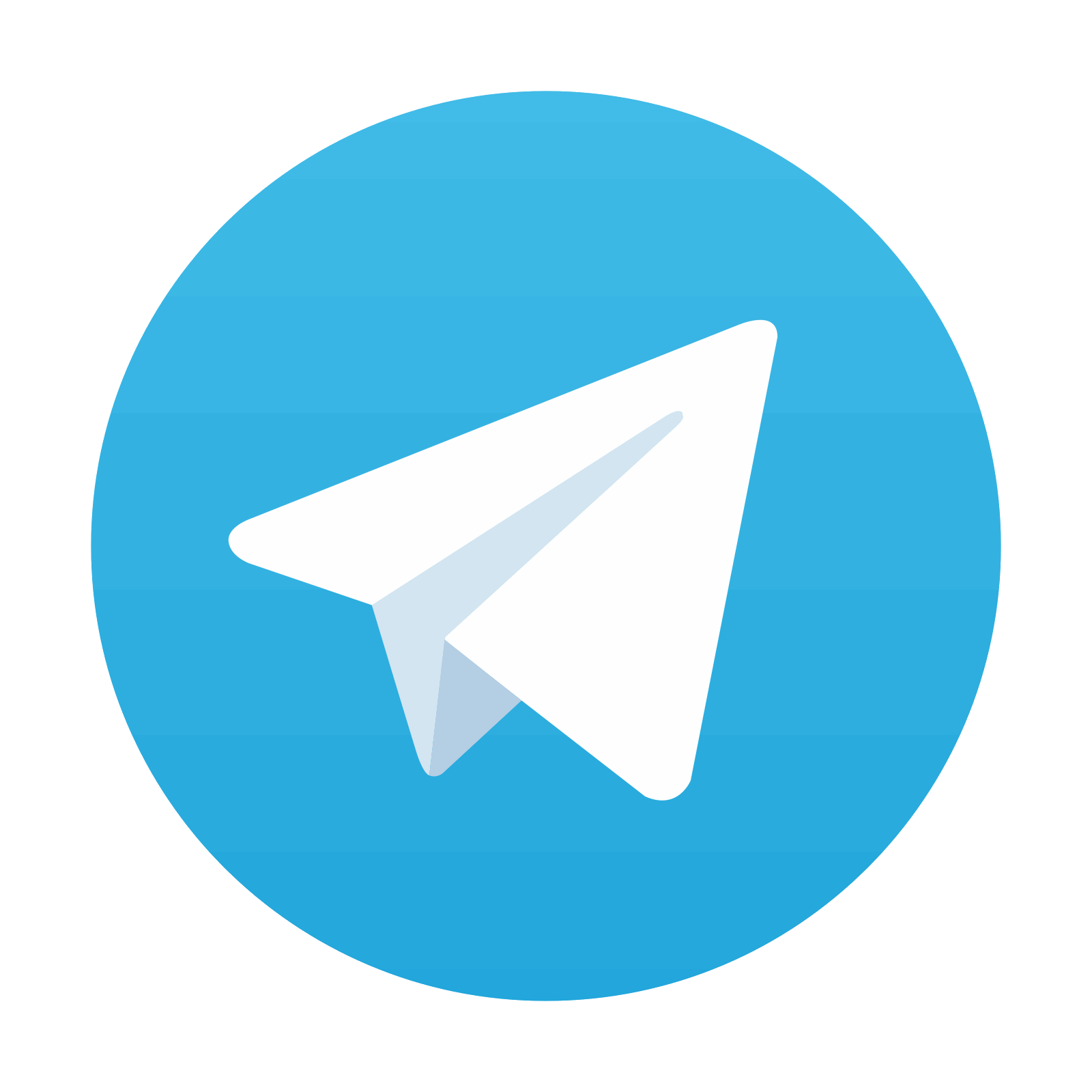
Stay updated, free articles. Join our Telegram channel

Full access? Get Clinical Tree
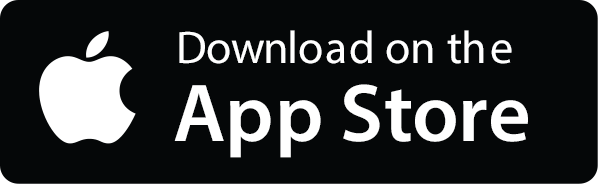
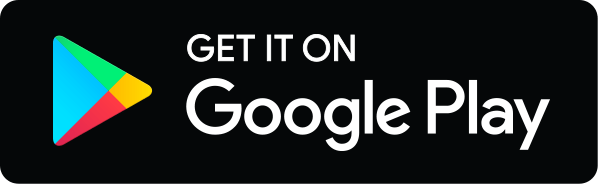