3 Neurophysiopathology
Giuliano Dolce and Leon Sazbon
Relationship with the Outer World
From a neurophysiological point of view, the brain structures and functional organization regulating conscious activity and allowing awake humans to express the contents of consciousness (that is to say, awareness of oneself and of the environment) only subsist when four specific conditions are met simultaneously:
• Presence of specific sensory inputs
• Presence of nonspecific, diffuse input from the ascending reticulothalamic activating systems diffusely projecting to the cortex
• Cortical activity with neuronal discharge in the medium–frequency range
• Activation of the primitive ipsilateral and contralateral motor systems, which stabilize spatial relationships and orient the system toward the source, thereby allowing for perception
These functional conditions must have a certain degree of interaction (the “theory of coherence”), and it is therefore insufficient for a certain cerebral area to be activated unless activation occurs to a degree that is appropriate to the activity of the interacting structures or functions. Information processing in the nervous system (which lacks a central pacemaker) becomes comprehensible only when the functional interaction between the activated cortical areas or brain systems is taken into account. This interaction is largely mediated through the thalamocortical system; another example elucidating the theory of coherence is given by the functional relationship between cortex, hippocampus and limbic system. Cortical information processing appears optimal when a theta rhythm of around 5 Hz is established in the hippocampus. This mechanism is considered to be the neurophysiological correlate for the phenomenon occurring (for instance during tests at school) when a strong emotional charge interferes with cortical information recall and causes a black–out of memory functions. It is therefore to no avail to activate a single cerebral structure in order to obtain improvement in performance. It would be necessary to determine the optimal coherence between the various structures to attain the best performance.
For many years, neurologists adhered to the concept that any given clinical picture would reflect the dysfunction (almost invariably a deficit, seldom hyperfunction or malfunction) of a particular structure or system. This induced Plum and Posner [1] to outline a number of different syndromes, characterized by their complex clinical picture and referred to as direct or indirect lesions of the encephalic brain stem, i.e. the syndrome of rostrocaudal deterioration responsible for coma.
The vegetative state, by contrast, results from a great variety of etiologies and pathophysiological mechanisms, in comparison with the relatively uniform clinical picture which, for instance, synthetically represents the “locked–out” syndrome [2]. Laminar encephalitis, selective damage to cortical neurons induced by insulin, several metabolic disturbances, anoxia, multiple or isolated lesions of the hemispheric structures, diencephalon, or brain stem, and lesions of the connections both between or within brain hemispheres, are all pathophysiological mechanisms capable of inducing a vegetative state.
The location, extent, and nature of the pathological anatomic lesions that determine the vegetative state do not correlate with the clinical progression. The fact that the deficit causing the vegetative state does not involve a single functional area alone, but depends on a reduction of the reciprocal connections among several brain structures, explains the lack of a definite anatomical correlate as a reference for recuperation. In our personal experience, we have often observed that patients with relatively inconspicuous morphological damage on brain computed tomography (CT) or magnetic resonance imaging (MRI) scans did not recover awareness, in contrast to unexpected recuperations in post-traumatic patients with multiple extensive lesions characterizing a severe postacute encephalopathy.
This discrepancy is most likely due to a single factor, represented by the degree of functional residual plasticity (very powerful at an age when maturation is incomplete, e.g. before 25 years), which allows a new postlesional organization to form (in general by the sixth month, but most often between the third and fourth months after brain injury), thereby establishing a certain degree of reciprocal activation among the major functional systems regulating the mechanisms needed for awareness to exist.
Following a certain functional hierarchy, the first of these major mechanisms is related to medium–frequency cortical neuronal discharge. Consciousness is not manifested at maximal discharge frequencies, during metabolic coma (e.g. in case of very high insulinemia), or when there are interfering physical factors such as low temperatures. Second, consciousness is almost completely lacking in the absence of specific input, as is seen for instance when sensory deprivation is induced experimentally. Also, conscious activity is not seen with reduced function of the ascending nonspecific activating systems (e. g. in barbiturate coma) or of the reticulothalamic system projecting diffusely to the cortex.
Although the functional anatomy and pathophysiology of these systems have been described and extensively covered in the literature, the motor component, which intervenes in mechanisms permitting contact with the outer world, has not. This contact is completely interrupted in the vegetative state.
The primitive motor systems are responsible for ipsilateral and contralateral movements, described in detail in the German literature [3,4]. We wish to describe these motor systems at this point, because the functional role they play in the pathophysiology of vegetative state is poorly known, although it is as relevant as that of the other systems described above.
Movements toward the right or left determine the spatial displacement of the body. Two distinct systems exist in each hemisphere to execute movements toward the same side (the ipsilateral system) or toward the opposite side (the contralateral system) (Fig. 3.1). The neurons of the ipsilateral system originate in several nuclei of the vestibular area, along the border of the floor of the fourth ventricle. The axons of these neurons rise, without crossing, to the mesencephalic periaqueductal reticular substance, where they terminate. The fibers of a second neuron rise, together with the uninterrupted fibers from the reticular substance, to the ventral intermediate nucleus (VIM) of the thalamus and reach cortical area 6aδ. A second system is represented by fibers from the central medial thalamic nucleus reaching the putamen. If this system is stimulated in the cat or monkey, the head and the body turn toward the stimulated side. A comparable stimulation effect has also been observed in humans. In wakefulness, this system is continuously active and pulls the body to that side when the opposite system is not operative. The erect body posture and straight backward movement known as backward locomotion are achieved in the wakeful state through a physiological level of excitation that is symmetrical in intensity on both sides of the brain stem.
The execution of the movements involved in contralateral turning of the body and eyes involves a far more complex functional arrangement than in the case of ipsilateral movements, the key anatomical structure of which is the “entopeduncular” nucleus of Montanalli Hassler, or internal pallidum. The contralateral thalamocortical system arises from several thalamic nuclei to reach different cortical areas. The known circuits are:
• From the anterior ventral nucleus of thalamus (receiving afferents from the internal part of the pallidum) to area 6aα
• From the posterior lateral nucleus of thalamus to area 8 and 6spl
• From the anterior nucleus to area 32, within the cingulum
All of these cortical areas project onto the other segments of the pallidum, along with fibers stemming from area 22.8 and probably also area 5. A part of these fibers crosses the internal pallidum, again reaching the anterior ventral nucleus and closing the pallidothalamocortical circuit.
The most important descending portion from the pallidum is the Q–bundle, which crosses the median line and terminates in a periaqueductal zone surrounded by the reticular formation, from which the dorsolateral tegmental tract descends. The effect on the movement of arms, legs and eyes takes place through the descending reticulobulbospinal and vestibulospinal fibers, a portion of which passes through the vestibular nuclei. The contralateral system in the frontal lobe (area 8) and parietal lobe (areas 5 b and 7) also provides fibers that descend directly through the internal capsule and cerebral peduncle to cross the median line at the mesencephalic level and terminate in the reticular formation. In humans, the selective stimulation of the internal pallidum and its efferent systems by stereotactic methods causes sideways movements of the body toward the opposite side and dilatation of the pupils. If the head is immobilized, vicarious movements toward the opposite side of the ocular bulbs can be observed. A weaker response is obtained with stimulation of the external pallidum.
Through GABAergic neurotransmission, the putamen inhibits both the internal and external pallida, with its stimulation provoking an ipsilateral sideways movement. Stimulation of the right putamen, for instance, provokes the same response as stimulation of the left pallidum – i. e., a sideways movement toward the right. These systems for sideways movements toward the opposite side are continuously active in the state of wakefulness. When activity is symmetrical and equivalent in both hemispheres, a tonic, erect position of the body directed frontward, not sideways, is achieved. A forward marching movement (forward locomotion) results when the symmetrical activation of ipsilateral systems is reduced simultaneously.
These subcortical and cortical mechanisms for sideways movement are also particularly important in the processes of apperception. In 1937, Kleist referred to an “optomotorisches System,” and also described auditory – motor and olfactory – gustatory – motor systems [5]. Even earlier, Sir Charles Sherrington (1857–1952) and Cécile Vogt (1875–1962) and Oskar Vogt had observed that when the cortical zone adjacent to the primary auditory area is stimulated, a gaze and head movement toward the opposite side appears. The same happens when cortical areas adjacent to the primary visual area are stimulated. Undoubtedly there are motor systems proximal to the primary sensory areas, the function of which is to turn the body toward the source of sensory inputs. Every sensory system, therefore, possesses its own regulatory motor apparatus within the cortex. The most obvious clinical example of the connection between perception and the opposite motor system is the pseudosyndrome of Balint (or congenital bilateral aplasia of frontal area 8), in which perception is not possible and objects are not seen, in the absence of any lesion of the visual system, when the head is immobilized and lateral eye movements are blocked. Clinical studies have also reported patients who are transiently unable to perceive their body and the contralateral hemispace after extended coagulation of the pallidum or thalamic nuclei receiving afferents. In addition, bilateral surgical ablation of the temporal poles (which probably interrupts the pallidal circuits) induces an akinetic mutism syndrome with prolonged loss of consciousness in epileptic patients [6] also in the absence of any lesions of the brainstem structures.
Fig. 3.1 Schematic outline of relevant pathways of the ipsilateral and contraversive primitive motor systems (adapted from Hassler [4])
Fig. 3.2 Metaphorical model of consciousness in three of its basic aspects: vigilance, conscious activity, and contents. The audience represents the outside world; the theater, the inside world; the actors, conscious activity; the stage lighting, light; and the text, contents. If the text does not exist, the performance cannot take place. If the actors also (representing the average frequency discharge of cortical neurons) talk too softly, the audience will not be able to understand them. If the light from the stage (vigilance) is too dim or blinding, the performance will not be visible. If the stage lighting is not directed toward the stage (ipsilateral and contralateral motor mechanisms), the actors will be unable to act. Only simultaneous functioning of all of these elements thus allows the performance (conscious state) to take place. A fourth aspect – consciousness of ourselves – is not suitable for inclusion in a neurological function model, as it concerns a specific condition, exclusive of human beings, and goes beyond physiological categories
In conclusion, it is evident that to maintain a state of consciousness that allows operative behavior, reciprocal interaction between several functional structures is required. A certain degree of functional coherence must exist among specific sensory afferents, nonspecific activating afferents from the major subcortical systems (mostly in the mesencephalon and diencephalon) projecting diffusely to the cortex, primitive (ipsilateral and contralateral) motor systems, and cortical neurons discharging at medium frequency. The absence of only one of these factors can induce loss of consciousness (Fig. 3.2).
This model allows for a better understanding of the different mechanisms and functional structures responsible for the onset of coma. The post-traumatic vegetative state, similar to a “locked–out” syndrome, is not a primary result of the absence of a single response, but depends on the impossibility of obtaining harmony with the outer world. This occurs mostly due to a lack of synchronous function among the motor components regulating the complex phenomena of perception, with the patient becoming unable to see because he cannot look, and unable to hear because he cannot listen.
From a clinical point of view, two conditions stand out: absolute akinesia (preceding every initiated activity of consciousness, which remains absent for the duration of the ongoing akinesia) and “orientation gaze” (Zuwendung, tracking). The latter involves a conjugate, source–oriented gaze caused by auditory or visual inputs recalling some memory (e. g., the face or voice of a particular person) and evoking displacement in the immediate surrounding space. This is almost always the first sign of recuperation, no matter how minimal. The relationship with the outer world, although interrupted during the vegetative state, remains or becomes possible as long as the major functional systems described above are sufficiently active to achieve a certain degree of reciprocal functional coherence. Humans can also use other systems, phylogenetically much older, that help establish contact with the “inner world” and can also maintain a residual function during the vegetative state, in the absence of wakefulness.
Relationship with the Inner World
Under physiological conditions, these two separate systems are very well integrated functionally, but can assume a certain degree of independence, particularly in the post–traumatic vegetative state.
Pierre Broca (1824–80) defined the limbic lobe as including the formations that constitute the cortical gyri, situated in a ring shape around the brain stem and representing the phylogenetically oldest part of the cortex. The limbic lobe includes diverse formations, such as the parahippocampal gyrus, the cingulate gyrus, the subcallosal gyrus, and the lower (and morphologically simple) cortex of the hippocampal formation. The hippocampal formation includes the hippocampus, dentate gyrus, and subiculum. In 1937, James Papez (1883–1958) outlined the way in which these structures are organized into a neuronal functional circuit and first suggested the “Papez circuit” as the anatomic substrate of emotional behavior: as emotions reach the conscious level and influence thought, cognitive functions in turn influence emotions. The concept of a limbic system was subsequently broadened, and direct and indirect relationships with other, especially neocortical structures, were better defined. This system integrates and coordinates the behavioral expression of emotional states or changes and represents the functional structure intended for the normal relationship of the individual with his or her own internal world. The vegetative and endocrine integrated hypothalamic responses to unpleasant or pleasant stimuli prepare the organism for flight, attack, sexual activity, and different behavioral patterns involving adaptation. These internal reactions are relatively simple and do not require conscious control, unlike those that are needed for the individual to adapt to modifications in the outer world. These are much more complex and less predictable and provide a far greater variety in quality and quantity of responses to stimuli. To relate to the outer world, responses are also devised to carry on peculiar projects and strategies. Perhaps this is the condition that led to the notable evolution of consciousness itself in humans, enabling them to meet the enormous complexity of the external environment [7]. The functions that regulate the relationship with the outer world are regulated, as we have seen above, by the major thalamocortical functional systems and through the sensory and motor systems.
Working Hypothesis on the Brain’s Functional Organization in the Vegetative State
Higher primates and humans rely on two major (anatomical and functional) arrangements of the brain to maintain contact with the inner world and the surrounding environment.
The main structures that are at the basis of these arrangements (which are interconnected, though to some extent independent) are outlined in Chapter 3, figs. 11–15. The neocortex, thalamus, basal ganglia, and ascending reticular activating system (ARAS) mediate higher brain functions such as perception, awareness, speech, motor skills, and motor planning. The limbic system (also including the hypothalamus) regulates instead the brain functions relating to the inner world of emotions, emotional reactions, mood, rage, fear, etc. that also can occur in the absence of vigilance, as is the case during sleep. Brain functions do not appear to develop from a large number of independent and partly interacting modules (the theory of localized functions), nor do they apparently depend on dynamic connections (the theory of equipotentiality). According to the coherence model, brain function appears instead to depend on the interplay between distinct areas/structures of the brain.
For this interplay to occur, efficient functional connection among active structures is necessary, and is normally provided by the brain’s white matter. We can now imagine that the axonal damage following brain injury varies (depending on the dynamics related to rotation, translation, linear or angular acceleration it sets into motion) and may exert different effects depending on the gray matter involved and the length of the axons. In addition to the lower mass compared to the other system, the limbic system is structurally arranged in space to form a “ring” that is located deep inside the brain and protected by the hemispheres and cerebrospinal fluid. Axonal connections are shorter compared to the long axons connecting the neocortex to the thalamus and brain stem, for example. Conversely, any secondary damage due for instance to hypoxia, ischemia, acidosis, intracranial increased pressure, or reduced perfusion should develop without significant topographic differences in the two major systems we have taken into consideration. A different distribution and entity of primary neuronal damage (especially in relation to diffuse axonal injury) is at the basis of and supports the practicability of our hypothesis.
The brain functions that allow contact with the outer world are still partly operative after several months in the vegetative state, and communication can also occur in the absence of vigilance –with vegetative responses (such as tears) being observed to external inputs with relevant emotional content (such as being called by a familiar voice). By contrast, vigilance remains a functional prerequisite for any contact, no matter how feeble, with the surrounding environment.
The limbic system is connected through the Gudden fasciculum (mamillary tegment) to other ascending sensitive (especially taste), reticular, spinal, and autonomic systems. This arrangement helps us understand the way in which the perception and recognition of the voice of a specific person, for example, may be possible via some functional bypassing of a damaged thalamocortical specific sensory system.
All this suggests that the axonal damage immediately following head injury may be less salient than the damage selectively affecting the interconnections among the brain stem, thalamus, and neocortex. Notably, widespread axonal damage of this type should affect long pathways dedicated to the primitive lateral motor systems as well as those mediating in voluntary motility. The akinesia, often generalized, complete, and persistent that is observed in the vegetative state is the clinical result of this pathophysiological arrangement. In agreement with this hypothesis, the reduced extension of the anatomical damage affecting the limbic system and its connections would justify the residual function of brain structures regulating contact with the inner world.
It therefore seems a practicable hypothesis that the pathophysiological conditions causing development into a vegetative state may already come into operation on brain injury, although they are eventually concealed by the concurrent coma. The system regulating vigilance, namely the ARAS, also suffers sudden functional blocking. This blocking, however, is not irreversible, and a discrepancy may underlie another condition peculiar to the vegetative state – i. e., the permanence of vigilance in the absence of any apparent contents. When this condition lasts for a long time (a month or more), a peculiar condition characterized by the lack of any relationship with the surrounding environment in association with a relatively spared emotional life and contact with the inner world (through responses mediated by flushing, mimicry, changes in heart rate, tears, etc.) can develop.
References
1. Plum F, Posner JB. Diagnosis of stupor and coma. Philadelphia: Davis, 1973.
< div class='tao-gold-member'>
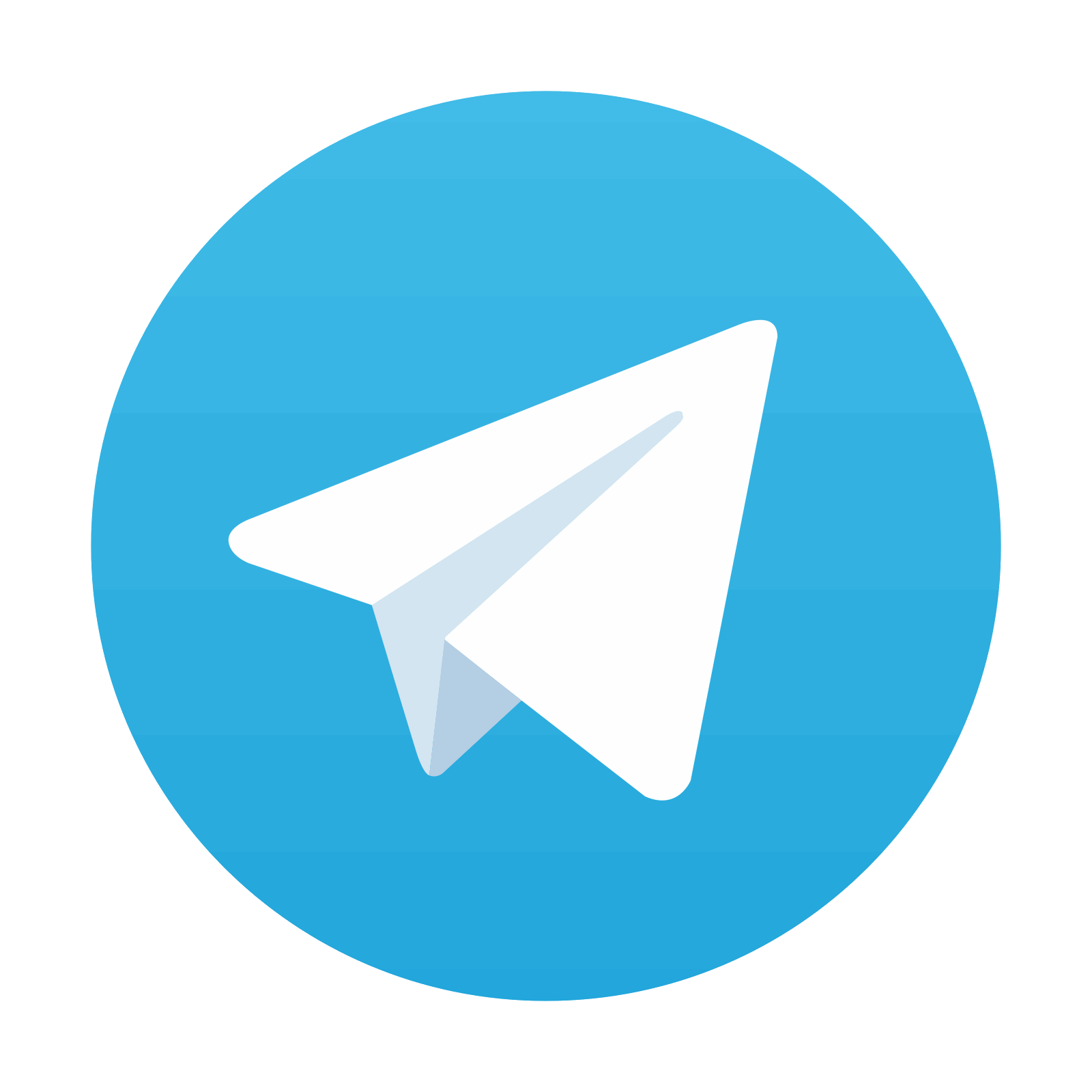