Fig. 4.1
Schemes of the dorsal loop of the BG. (a) The BG circuitry in normal brain. (b) The BG with degeneration of DA neurons in the SNc and with reduced DA activity in the striatum, which finally leads to a decreased output of the thalamus to the cortex. (c) The BG circuitry in a Parkinsonian brain plus an ectopic graft of nigral DA cells in the striatum. Dashed lines indicate reduced activity (the more dashes, the less activity). Thick arrows show a hyperactivity of neurons (the thicker, the more activity). Abbreviations in brackets are derived from rodent nomenclature (Modified from Schmidt [161])
4.3 Therapeutic Strategies to Treat Parkinson’s Disease
DA replacement therapy by applying, e.g., the DA precursor L-dihydroxyphenylalanine (L-DOPA) is still the gold standard treating the DA deficiency, and there are currently no strategies that can stop the degenerative processes and fully restore impaired motor patterns. New approaches, however, have been made to counteract the Parkinsonian symptoms especially at later stages of the disease [75], including deep brain stimulation [3, 92, 120], continuous apomorphine infusion via mini pumps [112], and—most strikingly—the neurotransplantation of fetal DA cells [36, 38, 71].
4.4 Drug-Based Therapy
The substitution of DA by using L-DOPA was introduced in the 1960s [6, 28]. L-DOPA naturally occurs as a metabolite during the synthesis of catecholamines. A major handicap of the L-DOPA therapy is that during the treatment phase, which may last some years, some patients develop severely disabling side effects: abnormal involuntary movements (motor fluctuations, dyskinesias) and psychiatric disturbances [75]. The rationale behind the dyskinesias has not been fully understood, but it has been discussed that the intermittent application of L-DOPA may be correlated with the occurrence of these side effects [22]. As a consequence, the drug-based therapy nowadays starts with DA agonists and other drugs (e.g., inhibitors of the enzyme monoamine oxidase B or NMDA antagonists), and L-DOPA is administered in small amounts later during the course of PD [75, 115]. Yet, the unwanted side effect will occur sooner or later no matter how the disease is managed and treated and will eventually outweigh the functional benefits.
4.5 Neurotransplantation
The success of the clinical trials—see below—would not have been possible without (and still depends on) intensive research in animal models of neurodegenerative diseases and neurotransplantation. The quest for optimized cell sources and better cell survival and the search for less trauma-causing surgical techniques are still ongoing and are the basis for this fascinating field of research (for a detailed historical review, see [36, 38, 153]).
Advances in neural transplantation and its functional assessment of graft impact on the host (rodent or human) have gained momentum over the past 120 years. W. Thompson’s 1890 [142] report of grafting adult neural tissue is one of the first records of neurotransplantation ever published. Almost three decades later, Elizabeth Dunn [33] published results of the first embryonic tissue transplantation (into neonatal rats). During those early days of neural transplantation, the main focus of many researchers was on anatomical and developmental studies examining new methods of brain repair and trying to create an environment that would allow grafted tissue to survive. Much less attention was paid to the functional effects of those grafts as the neuroscience community struggled to provide decent graft survival at all; cell sources, donor age, transplantation sites, and the immune response (matching between donor and host) were large obstacles that had to be overcome first. It was only in the 1940s when the first rather descriptive and vague reports about behavioral effects on grafted animals were published: Sugar and Gerard [138] examined the impact of muscle and neural tissue bridge transplants on functional and structural improvement in a rat spinal cord injury model. Greene and Arnold [55] analyzed guinea pigs after the transplantation of human brain tissue into the pigs’ brain hoping to increase the pigs’ “intellect,” but—to their disappointment—only an increased libido and no changes in cognitive function could be detected. These two studies, though, were at the forefront of behavioral assessment of grafted animals.
The lack of standardized behavioral assessment and functional evaluation was one of the main limitations hindering a breakthrough of neurotransplantation as potential therapy; it took another thirty years of intense research before researchers could investigate the functional impact of grafted tissue on (motor and cognitive) behavior following transplantation. The symptomatology of PD has been modeled in various animals, mainly in rats and mice, but also in monkeys; ethical and experimental costs, though, limit the use of primates in transplantation research, and, consequently, much less studies examine the effects of neural grafts on motor performance in monkeys [36, 77, 147]. The arrival of better rodent model systems was a prerequisite for the success of brain repair. An important landmark in this context was the development of the 6-hydroxydopamine (6-OHDA) lesion model in rats for preclinical research (Fig. 4.2) allowing translational conclusions from the animal to human PD [64, 148]. Therefore, we focus on rodent studies in this chapter.
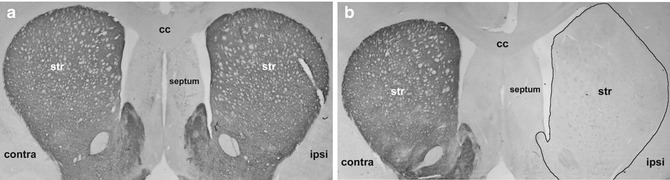
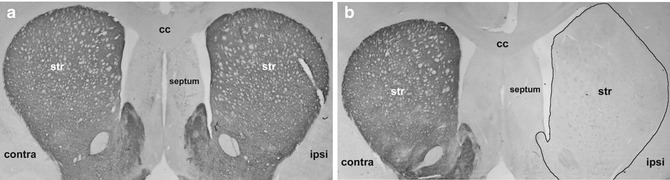
Fig. 4.2
TH staining for control rats and unilaterally 6-OHDA-lesioned rats. Both pictures show a brain section at +1.0 from bregma: (a) control brain with no surgery at all. Both hemispheres are clearly stained for TH in the striatum. (b) Unilateral medial forebrain bundle 6-OHDA lesion: no TH staining and complete DA depletion on the ipsilateral (right) hemisphere. Contra contralateral, ipsi ipsilateral, str striatum, cc corpus callosum (Modified from Klein et al. [73])
4.6 Neurotransplantation in the Unilateral Rat Model of Parkinson’s Disease
On the basis of the hemiparkinsonian rat model and the assessments of the motor deficits after 6-OHDA lesions, the two trend-setting publications, which developed the idea of “brain repair” raising hope that functional improvement after cell replacement therapy was feasible, were published by Björklund and Stenevi [11] and by Perlow et al. [109]. These studies gave a big impetus to the use of grafting cells in models of neurodegenerative diseases [75]. The early studies of functional restoration after 6-OHDA lesions focused on graft integration and survival and on transplantation techniques demonstrating that the DA grafts can partially reinnervate the striatum, that they release DA controlled by cortical afferences, and that they connect downstream to striatal (host) neurons via new synapses [23, 25, 32, 46, 57, 94, 118, 119, 135, 152]. On the molecular level, the expressions of the upregulated enkephalin, D2-receptor, and GAD67 mRNA within the reinnervated areas of fetal striatal DA grafts are nearly normalized [75]. Other changes such as the lesion-induced downregulation of substance P are less affected [19, 20, 24, 156]. Also, transplantation into DA-depleted rats restored DA levels [9, 45, 127] and resulted in almost normal electrophysiological firing profiles of grafted neurons [54, 124, 159] and the formation of synaptic connections to host neurons [46]. Specific training and enriched environment have also been proven to promote neurogenesis in adult mammals and to be beneficial to the development of the grafts in the host brain [13, 30, 31, 113]. Interestingly, most of the abovementioned effects were clearly dependent on the degree of DA reinnervation in the host striatum after transplantation. Therefore, a complete reinnervation of the diseased striatum remains the main objective in PD research.
Many behavioral tests have been carried out to monitor graft-related changes of motor behavior [75]. Drug-induced rotation has been the first successful behavioral (simple motor) assessments of graft function [11, 109, 149]. Drug-induced rotation, e.g., by injecting amphetamine, evaluates the rotational bias after unilateral 6-OHDA lesion in rats. Full recovery or even overcompensation was observed in grafted animals in this test, i.e., transplanted rats had no rotational bias anymore or even rotated to the other side. Surgical removal of the graft abolished any improvement seen after grafting clearly indicating the beneficial impact of the transplants on rotational bias [10, 35]. Other simple motor tests also underlined the positive effects of DA grafts in DA-depleted rats, e.g., contralateral neglect, adjusting step test, vibrissae-evoked hand-placing test, Rotarod, akinesia, cylinder, or catalepsy (for a review, see [36]). The functional restoration of more complex behaviors such as skilled forelimb use is more difficult to achieve (for a review, see [77]). The first studies did not show any improvement of motor pattern of the forepaws—despite the obvious improvement in, e.g., drug-induced rotation [1, 34, 90, 97, 104]. With the introduction of the microtransplantation approach [101], the injection of multiple deposits of DA cells could partially restore the complex grasping behavior of PD rats [27, 73, 107, 121, 122]. These results clearly underline the demand for a complete and specific restoration of the degenerated nigrostriatal pathway to achieve functional recovery.
Interestingly and importantly, success or failure in restoring motor behavior as measured by the aforementioned tests depends on the graft placement in the striatum highlighting that the corticostriatal circuits and the striatum—similar to the cortical motor map—are topographically structured [70, 73, 96, 140] demanding site-specific transplantation coordinates that are critical for each individual behavior investigated by the different tests.
It is important to bear in mind that most transplantation approaches in PD are based on an ectopic cell transplantation concept (see Fig. 4.1 and Winkler et al. [157]). That is, DA cells are “misplaced” into the adult striatum (their target area where the DA terminals end, but actually no DA neuron cell bodies are naturally located) [75]. Hence, if transplanted homotopically, newly generated axonal fibers of the injected neurons only insufficiently find their targets (the distance between the substantia nigra (SN) and the striatum is too long and the appropriate growth factors are not expressed); some groups, however, recently showed some considerable yet not fully functional reconstruction of the nigrostriatal pathway after intranigral transplantation of DA neurons [136, 137, 141]. In line with these findings, if cells are transplanted into the neonatal SN or if so-called bridge grafts are used, a modest reinnervation of the nigrostriatal pathway is also possible [99, 100, 155, 157]. Several attempts to create an axonal outgrowth-friendly environment in the adult brain are currently under investigation: (i) applying neuronal growth factors (via injections or by gene therapy [4, 12, 144]), (ii) blocking surface proteins which are repellent to neuronal growth cones [61, 130], and (iii) transplanting cells in two steps, firstly to create a neonatal environment and secondly to rewire neuronal circuits [145].
The stereotactic transplantation technique of injecting cells into the brain has also changed over the years [75]: at the beginning, monoamine-rich solid pieces of the VM were grafted into the striatum, the lateral ventricle, or cortical cavities [11, 109]. Poor functional restoration and surgery-related traumata were the driving force to develop new neurotransplantation techniques. Bjorklund et al. [8] established the cell suspension technique which has become the “hallmark protocol” of cell preparation and implantation. Nikkhah et al. [101] further optimized this protocol and introduced glass capillaries for the infusion of single cell suspensions (Fig. 4.3) [102, 134]. This technique caused fewer traumata than the use of a metal cannula. The so-called microtransplantation approach resulted in better functional integration, graft survival, and enhanced reliability of survival of the graft (fewer differences in the survival rates of grafted cells). The new technique also offered the option of injecting multiple deposits of DA cells into the entire DA-depleted striatum and/or SN [42, 52, 60, 73, 76, 103, 116, 121]. Together with improvements not only in basic sensory and motor behavior but also in more complex processes such as skilled reaching or cognitive tasks (for a detailed review, see [36, 77, 86, 153]), brain repair with primary fetal cells has been the most successful type of brain repair so far.
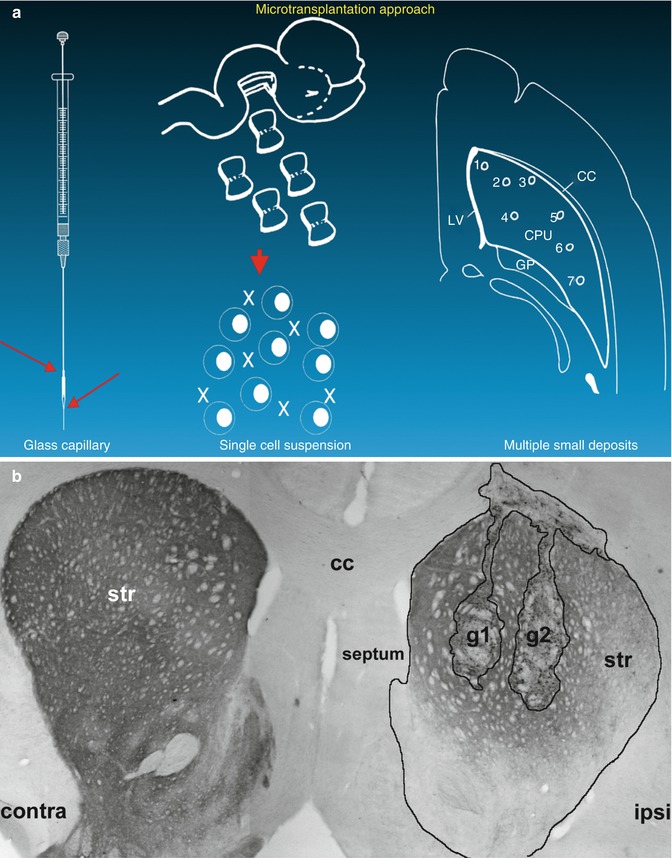
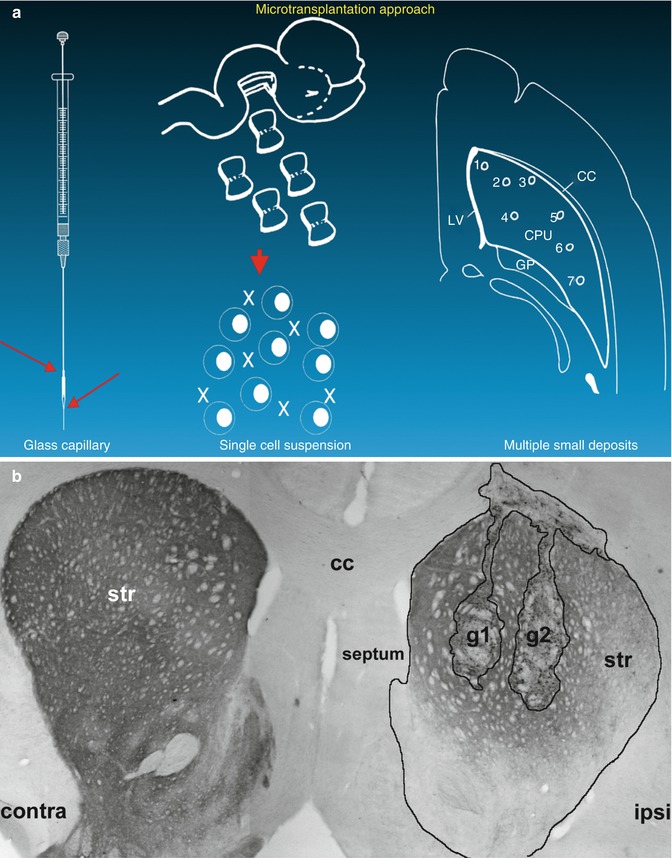
Fig. 4.3
Schematic description (a) of the microtransplantation approach as published by Nikkhah et al. [101]. Tyrosine hydroxylase (rate-limiting enzyme within the metabolism of DA; often used to visualize lesion and graft effects) staining (b) in unilateral lesioned rats with two grafts of E14 VM-derived cells (g1 and g2). Contra contralateral, ipsi ipsilateral, str striatum, cc corpus callosum (Modified from Klein et al. [73])
Despite these promising results, using primary DA cells still bears the burden of major ethical concerns including the handling of legally aborted fetuses, health and infectious disease issues, and logistics and availability [38, 47]. Hence, new cell sources—other than fetal tissue—must be made available [75]. Many studies (for a review, see Trueman et al. [147]) have made use of ESCs [41, 91, 143], neural stem cells [117, 132], stem cells from umbilical cord blood [5, 56, 160], adult stem cells [2, 50, 125], or cells that are genetically engineered in vitro overexpressing, e.g., growth factors or drugs [12, 16, 17, 18, 26, 40]. More recently, iPSCs [98, 139] and iNCs [150, 151] have become a major research area in brain repair (for a review, see Trueman et al. [147]). But not only is the cell source a concern but also the ability to turn those cells into DA phenotype has been a major challenge. There are morphological and anatomical differences between the DA cells of the SN pars compacta (A9) and the ventral tegmental area (A10). Recent attempts to proliferate and differentiate DA neurons in vitro were successful in providing not only a large number of DA neurons but also a relatively high number of region-specific DA A9 neurons [114, 133].
The use of ESCs has been discussed very controversially during the last years; moreover, using cells from human blastocysts is as ethically controversial as using fetal cells. Much success has been achieved in cultivating and proliferating those cells [71, 85, 131]. Turning undifferentiated pluripotent ESCs into a DA phenotype to a considerable and sufficient amount, however, is difficult and scientists are still in the process of finding optimal cell culture conditions. Another major concern of the use of ESCs is the potential tumor growth. When ESCs are transplanted in vivo, they continue proliferating resulting in severe tumor and teratoma growth. In contrast, it has never been reported that fetal grafts trigger any tumorlike tissue. In order to better control ESCs in vivo, pre-differentiation protocols have been established avoiding the transplantation of immature cells. In vivo experiments showed beneficial effects in an animal model of PD [72], but others [123] suggest that those results should be interpreted more carefully, and it reports a still ongoing proliferative activity of cells in the graft core.
To overcome ethical concerns, immunological mismatch, and the tumorigenic properties of ESCs, the use of autologous and/or adult stem cells has been developed over the years [39]. This includes still residing cells derived from brains of adult donors or cells derived from nonneural sources, e.g., skin fibroblast. This exciting research area is still relatively young and is its early phase of development. Functional improvement after iPSC transplantation has already been shown [15, 62, 154], but differentiation ratios into the desired phenotypes are still relatively low and—in case of iPSCs—these cells turn back into pluripotent state during the cultivation process meaning that they reactivate tumorigenic properties again [154]. iN cells, in contrast, omit these stages and much effort is currently put into developing protocols that could be eventually translated into a clinical setting where patients donate their own skin biopsy for in vitro cultivation, DA neurons will be harvested from those cell cultures, and a cell suspension from those cultures will be reinjected into the patient [151].
4.7 Clinical Neurotransplantation
Since the late 1980s, several open-label clinical trials and two placebo-controlled studies have been performed, and in total, more than 250 PD patients have received transplants of human fetal DA tissue worldwide [36, 38, 75, 87].
The results of the open-label trials were very promising: the grafts survived and integrated well into the host striatum, and the transplanted cells expressed a DA phenotype. Furthermore, the grafted patients showed a significant improvement in motor function as measured by the unified PD rating scale (UPDRS) and the reduction of their daily drug intake [59, 80, 81, 88, 93, 94, 95, 110, 111, 158]. These effects could be observed in some patients over a period of more than 10 years. Cognitive function was less affected; some patients did not improve at all [126, 146].
Open-label clinical trials included the placebo effects in patients, researchers, and clinicians [48, 49, 129]. Therefore, placebo-controlled clinical trials were carried out by Freed et al. [45] and by Olanow et al. [106]. Surprisingly, the outcome of these first double-blinded studies was disappointing: the functional improvement as seen in the first open-label studies could not be repeated, and, even worse, several patients (15–57 %) developed severe side effects such as abnormal involuntary movements (dyskinesias; for a review, see Lane et al. [83]). Remarkably, a retrospective analysis of patients from the earlier open-label trials also revealed mild dyskinesia in some of the patients [58]. Interestingly, the younger patients (< 60 years) showed a significant improvement in UPDRS scores, but there was no effect on the overall performance including all patients in the Freed et al. study [44]. Winkler et al. [158] comprehensively analyzed and compared the results of both study designs (open-label vs. placebo-controlled). The authors described fundamental methodological differences between these two trials and earlier open-label trials, which made it difficult to compare the results of those studies. These discrepancies in methodologies performing the clinical studies may have been the central key point for the disappointing outcome of the placebo-controlled trials. For example, patients of the placebo-controlled studies were not immunosuppressed at all or only for a short period of time, and, consequently, postmortem data suggested that there was a strong immune response, i.e., graft rejection, ongoing. Patients from the early open-label trials were immunosuppressed for life, and no graft rejection was documented when patients were compliant to their immunosuppressive therapy. Winkler et al. [158] concluded that the double-blinded clinical studies “provide the first observations of graft-induced improvements that are distinguishable from placebo effect” and that the transplantation procedure itself, the tissue handling and storage, the immunosuppressive treatment, and the selection of patients must be improved and standardized. Taking these suggestions into consideration, Winkler et al. [158] claim that “cell transplantation can be developed into a safe and efficacious restorative therapy for advanced, although not too seriously affected, PD patients.”
The results of the Freed and Olanow studies, however, let all clinical trials come to a halt worldwide and triggered intense investigation of unwanted graft-related side effects [16, 21, 51, 52, 82, 84]. Now, about a decade later, researchers and clinicians feel confident enough that graft-induced dyskinesia has been well investigated and some key factors such as selection of younger patients, responsiveness to L-DOPA, the impact of serotonin on the development of dyskinesia, and transplantation coordinates have been identified. Based on this research, a European multicenter consortium has been founded with the support from the European Union (TransEuro within the FP7 framework). Patients from the United Kingdom, France, Germany, and Sweden will be transplanted with fetal VM-derived cells beginning in summer 2013. The results of this trial will have groundbreaking implications on the future of neurotransplantation in PD.
4.8 Summary
Neurotransplantation is a promising therapeutic tool to restore sensorimotor function in PD. Many additional factors such as physiotherapy, supporting drug therapy, different cell sources, pretreatment of cells, and/or neuroprotection have to be taken into consideration [75]. The combination of all will most probably help to find the optimal therapy and disease management in PD and, potentially, also for other neurodegenerative diseases. Intensive experimental preclinical research is still required to optimize current protocols, and the ethical issues which arise from the use of fetal tissues and ESCs must be solved. Thus, finding alternative cell sources and a sufficient in vitro propagation and differentiation of (stem) cells will remain an important challenge for the future. In summary, neurotransplantation will continue being a restorative methodology, and more research is needed to identify the mechanisms that could stop the ongoing neurodegeneration during the course of PD and to detect the underlying pathology of PD so that the disease can be stopped at its core.
References
1.
Abrous DN, Torres EM, Dunnett SB (1993) Dopaminergic grafts implanted into the neonatal or adult striatum: comparative effects on rotation and paw reaching deficits induced by subsequent unilateral nigrostriatal lesions in adulthood. Neuroscience 54:657–668PubMed
2.
Altman J, Das GD (1965) Autoradiographic and histological evidence of postnatal hippocampal neurogenesis in rats. J Comp Neurol 124(3):319–335PubMed
3.
Ardouin C et al (1999) Bilateral subthalamic or pallidal stimulation for Parkinson’s disease affects neither memory nor executive functions: a consecutive series of 62 patients. Ann Neurol 46:217–223PubMed
4.
Behrstock S et al (2006) Human neural progenitors deliver glial cell line-derived neurotrophic factor to parkinsonian rodents and aged primates. Gene Ther 13:379–388PubMed
5.
Bicknese AR et al (2002) Human umbilical cord blood cells can be induced to express markers for neurons and glia. Cell Transplant 11:261–264PubMed
6.
Birkmayer W, Hornykiewicz O (2001) The effect of 1-3,4-dihydroxyphenylalanine (L-DOPA) on akinesia in parkinsonism (1961). Wien Klin Wochenschr 15(113(22)):851–854
7.
Birkmayer W, Riederer P (1985) Die Parkinson-Krankheit: Biochemie, Klinik, Therapie. Springer, Wien/New York
8.
Bjorklund A et al (1983) Intracerebral grafting of neuronal cell suspensions. II. Survival and growth of nigral cell suspensions implanted in different brain sites. Acta Physiol Scand Suppl 522:9–18PubMed
9.
Bjorklund A, Stenevi U (1985) Intracerebral neural grafting: a historical perspective. In: Bjorklund A, Stenevi U (eds) Neural grafting in the mammalian CNS. Elsevier, Amsterdam, pp 3–14
10.
Björklund A et al (1980) Reinnervation of the denervated striatum by substantia nigra transplants: functional consequences as revealed by pharmacological and sensorimotor testing. Brain Res 199(2):307–333PubMed
11.
Björklund A, Stenevi U (1979) Reconstruction of the nigrostriatal dopamine pathway by intracerebral nigral transplants. Brain Res 177(3):555–560PubMed
12.
Björklund T, Cederfjäll EA, Kirik D (2010) Gene therapy for dopamine replacement. In: Björklund A, Cenci MA (eds) Progress in Brain Research, 184th edn. Elsevier B.V., Amsterdam