71 Optimizing Cerebral Perfusion Pressure in Acute Brain Injury
Pedro Kurtz 1, Neeraj Badjatia 2
1 Neurological Intensive Care Unit, Department of Neurology, Columbia University Medical Center, New York, USA
71.1 Introduction
The management of cerebral perfusion pressure (CPP) is an integral part of hemodynamic resuscitation of brain injured patients. CPP, the difference between mean arterial pressure (MAP) and intracranial pressure (ICP), plays a major role in defining blood flow to the injured brain. The clinician’s main task at the bedside is to ensure adequate delivery of oxygen and nutrients to match neuronal metabolic demands and avoid secondary brain injury. Since delivery is dependent on cerebral blood flow (CBF), finding the optimal CPP is crucial to limit secondary injury and improve outcomes.
The objective of this chapter is to discuss how to define and achieve optimal CPP goals based on individual patient profiles and different clinical situations.
71.2 Multimodal Monitoring
A comprehensive approach to goal-directed interventions requires that organ function is assessed to indicate the need and evaluate the response to specific treatments. The ideal monitoring tools in the ICU should be continuous, noninvasive and accurate measurements of the end-organ function of interest. In order to monitor cerebral function parameters there are no good substitutes to intracranial probes that measure intracranial pressure, brain tissue oxygen tension and aerobic metabolic activity. Our approach to multimodal monitoring focuses on systemic and cerebral parameters and the interrelation between them, as shown in Table 71.1.
Hemodynamic monitoring is a cornerstone of the management of critically ill neurological patients. We use either VigileoTM or PICCOTM devices to monitor mean arterial pressure and pulse-contour analysis of the arterial waveform to generate continuous cardiac output [1-5]. Both technologies also offer continuous measurement of stroke volume variation (SVV) which is used as an estimate of fluid responsiveness in mechanically ventilated patients. The PICCOTM further calculates extravascular lung water (EVLW) and global end-diastolic volumes based on a transpulmonary thermodilution curve [5]. Through a central venous line, preferably on the subclavian site, central venous pressure (CVP) and oxygen saturation are continuously monitored. Arterial lactate and arterial-venous delta CO2 (a-v ΔCO2) allow estimation of tissue hypoperfusion and inadequate systemic CO2 washout, an indication of inappropriate cardiac output [6-10].
Multimodal monitoring parameters of brain function are shown in Table 71.1. It is composed of intracranial measurements of ICP, partial pressure of brain tissue oxygen (PbtO2), microdialysis, brain tissue perfusion (HemedexTM) and cortical depth continuous electroencephalography (cEEG) [11,12]. In addition to the intracranial monitoring, surface cEEG and jugular bulb oxymetry complete the armamentarium of tool available. The intracranial modalities are usually used as a bundle, inserted into a multi-lumen bolt and/or tunneled in, as necessary. PbtO2 is a measure of tissue oxygen tension and is believed to reflect the balance between delivery, consumption and tissue diffusion of oxygen [13-16]. Microdialysis allows measurement of glucose, lactate and pyruvate in a small volume of tissue around the catheter. High lactate/pyruvate (L/P) ratios indicate anaerobic metabolism and if associated with low brain glucose, suggest tissue metabolic crisis [17,18]. Tissue perfusion around the area of the probe is estimated through a thermodilution method between two thermistors along the probe (HemedexTM) [12,19].
Modality | Purpose and general goals | Description and comments |
Systemic | ||
Continuous cardiac index (CI) | Above 2.5 l/min/m2 | BSA indexed cardiac output based on pulse contour analysis of arterial waveform |
Mean arterial pressure (MAP) | Above 70 mmHg | Invasive MAP through radial or femoral artery |
Stroke volume variation (SVV) | Below 10% | Ventilation induced variability of stroke volume |
Global end diastolic volume index (GEDI) | Above 600 ml/m2 | Estimated maximal volume of 4 heart chambers based on transpulmonary thermodilution curve |
Central venous oxygen saturation (scO2) | Above 70% | Blood oximetry measured by central venous catheter located at right atrium or superior vena cava |
Central venous pressure (CVP) | Above 5 mmHg | Intravascular pressure measured by central venous catheter located at right atrium or superior vena cava |
Extravascular lung water index (ELWI) | Below 10 ml/kg | Estimate of the intra-thoracic volume of water outside the blood vessels base on transpulmonary thermodilution |
Cerebral | ||
Continuous eletroencephalography (cEEG) | Seizure and ischemia detection | Surface continuous EEG with quantitative parameters |
Intracranial pressure (ICP) | Below 20 mmHg | Intracranial parenchymal or ventricular pressure |
Cerebral perfusion pressure (CPP) | Above 60 mmHg | MAP-ICP |
Brain tissue oxygen tension (PbtO2) | Above 15 mmHg | Partial pressure of oxygen measured at brain tissue level |
Cerebral microdialysis | Lactate/pyruvate ratio <40 and glucose >0.7 mmol/l | Lactate, pyruvate and glucose measured at brain tissue level |
Brain tissue perfusion (rCBF) | Above 20 ml/100 g/min | Regional brain tissue perfusion based on thermodilution method |
Depth continuous EEG | Seizure and ischemia detection | Subcortical continuous EEG |
Jugular venous bulb oximetry (sjO2) | Above 65% | Blood oximetry measured at jugular venous bulb |
Table 71.1. Multimodal monitoring. Systemic and cerebral parameters.
71.3 Goal Directed Systemic Resuscitation
Systemic hemodynamic resuscitation should always precede brain targeted interventions. Comatose patients with severe brain injury are likely to be mechanically ventilated and should be monitored with an invasive arterial line, a central venous catheter and an intracranial pressure probe. Semi-invasive continuous monitoring of cardiac output and stroke volume variation are possible through pulse contour analysis of the arterial waveform. GEDVi is used as a volumetric static measure of preload and extra-vascular lung water measurements as an indicator of pulmonary edema. Central venous pressure and central venous oxygen saturation (scvO2) complement this comprehensive list of hemodynamic monitoring parameters.
Markers of end organ hypoperfusion, such as high lactate and low central venous oxygen saturation, indicate inadequate oxygen delivery and should prompt interventions in order to achieve optimal mean arterial pressure and cardiac output [9,10,20]. Assessment of fluid responsiveness should follow based on GEDV and stroke volume variation (SVV). SVV greater than 10% and GEDVi less the 600 ml/m2 generally indicate that the patient will respond to a fluid challenge with either 500 ml of crystalloid or 250 ml of colloid [21]. An increase in cardiac output confirms the response to the fluid challenge.
After optimal preload is achieved, MAP should be maintained above 70 mmHg with norepinephrine and cardiac index kept above 2.5 l/min/m2 with dobutamine or milrinone, if necessary. After hemodynamic stabilization, end organ perfusion parameters should be reassessed. Urine output >0.5 ml/kg/h, clearance of arterial lactate, scvO2 >70% and delta a-v ΔCO2 <6 are good indicators of effective systemic resuscitation.
71.4 Multimodal Monitoring and Goal Directed Cerebral Resuscitation
The goal of advanced neuromonitoring in patients with severe brain injury is to allow early detection of complications and ensure adequate delivery of oxygen and nutrients to the brain in order to avoid permanent damage. After the primary event, a number of processes can lead to secondary brain injury. Nonconvulsive seizures after traumatic brain injury, vasospasm after subarachnoid hemorrhage, expansion of the hematoma after intracerebral hemorrhage and increased ICP after cardiac arrest are examples of detectable complications that progress in the ICU and can be captured by comprehensive monitoring of brain function [22-31]. Early detection and prompt intervention can potentially prevent irreversible damage.
Continuous multimodality neuromonitoring includes ICP, PbtO2, microdialysis, continuous EEG (surface and depth) and tissue perfusion. These probes are introduced at the bedside through a multi lumen bolt and/or tunneled in subcutaneously. All the data is stored and continuously displayed at the bedside along with systemic monitoring parameters.
An integrative approach to brain oxygenation, metabolism, electrical activity and perfusion allows the clinician to understand the pathophysiology of events and to individualize clinical therapy. Small elevations in ICP below traditional thresholds may compromise perfusion and lead to brain tissue hypoxia and metabolic crisis. Early treatment to optimize perfusion may reverse these alterations and avoid a vasodilatory cascade that leads to refractory intracranial hypertension [32]. Similarly, a reduction in regional blood flow to ischemic levels may cause reduced alpha/delta ratios, elevated lactate/pyruvate ratios and low PbtO2 [14,18,33]. Early CPP optimization and balloon angioplasty may also reverse ischemia and avoid permanent deficits.
CPP is the primary determinant of cerebral blood flow and oxygen delivery to the brain [34-36]. It is thus a powerful and practical tool at the bedside to achieve adequate balance between oxygen and nutrient delivery and the brain’s metabolic demand. Instead of relying on arbitrary thresholds to target CPP, functional assessment of the brain permits goal directed management of cerebral hemodynamics and individualized targets of optimal CPP.
The main goals when optimizing CPP are to maintain PbtO2 >15 mmHg, sjO2 >65%, L/P ratio <40 and regional perfusion >20 ml/100 g/min [18,37]. The first step is usually to optimize cardiac preload with fluids in patients who are fluid responsive. Once adequate preload and an SVV<10% are achieved, MAP and cardiac output can be improved with vasopressors and inotropes, respectively. We initially keep cardiac index above 2.5 and CPP above 60 mmHg.
CPP and cardiac output, though critically important, are only 2 pieces of the homeostatic puzzle where factors such as blood rheology, serum osmotic pressure, glucose and arterial pO2 influence ongoing neuronal injury. Taking into account the complexity and interactions between these variables, efforts are undertaken to adjust sedation, serum osmolarity, blood glucose control and exclude surgical complications through neuroimaging while hemodynamic is optimized.
When brain physiological targets are not yet achieved, further efforts to increment CPP and cardiac output are undertaken. Supranormal levels are defined as optimal if they correlate with improvements in the cerebral oxygenation and metabolic profile.
71.5 Specific Situations
71.5.1 Subarachnoid Hemorrhage (SAH)
Patients with aneurysmal SAH are at increased risk for rebleeding, hydrocephalus and vasospasm. The highest rates of rebleeding occur in the first 3 days after SAH and surgical clipping or endovascular coiling of the ruptured aneurysm should be pursued as soon as possible after admission. While the aneurysm is unsecure, systemic hypertension should be avoided but hemodynamic stability is crucial to avoid cerebral hypoperfusion, acute ictal infarcts and cerebral circulatory arrest [38].
Liberal fluid resuscitation with crystalloids is commonly necessary in poor grade SAH patients before securing the aneurysm. Although frequently hypertensive, patients are admitted with relative intravascular volume depletion – due to natriuresis and systemic inflammatory response – and 2 l of normal saline are acutely administered to maintain organ perfusion. If a MAP goal of 70 mmHg is not achieved, norepinephrine is initiated. If, instead, the MAP is higher than 130 mmHg or systolic blood pressure is above 180 mmHg, continuous infusion of nicardipine is started to avoid unsafe blood pressure levels.
Comatose patients with suspected hydrocephalus should emergently have an extraventricular drain (EVD) placed and ICP monitored [38]. Once the aneurysm is treated and multimodality monitoring data is available, optimal CPP should be pursued as described previously.
Vasospasm is a major concern after SAH, especially between days 4 and 14 after the initial hemorrhage. Up to 50% of patients will develop symptomatic vasospasm after SAH. Especially those who present with diffuse and thick cisternal blood are at increased risk for delayed infarcts due to vasospasm. There is increasing evidence that multimodality monitoring allows detection of cerebral ischemia due to vasospasm before clinical signs develop [14,15,18,27,33]. Integrating electrical activity monitoring through quantitative EEG, oxygenation with PbtO2 and oxidative metabolism measured by microdialysis may create a window of opportunity for intervention before clinical signs appear and permanent deficits take place. Recent evidence suggests this window may vary from hours to a few days [17,39].
Dynamic changes in alpha/delta ratio, a relative reduction in PbtO2 or elevation in lactate/pyruvate ratio should alert the bedside nurse or clinician of potential ongoing ischemia. Repeated clinical and transcranial Doppler examinations usually follow. If vasospasm is suspected, especially in a comatose or sedated patient, a perfusion CT scan is indicated to evaluate the extent of the perfusion deficit. At the same time a trial of increased CPP and cardiac output is undertaken with reversal of the altered parameter as the goal. A positive response to improved cerebral blood flow is seen within minutes for the continuously measured PbtO2 and qEEG parameters and is reflected in the next 1 to 2 hours of microdialysis measured lactate and pyruvate. If a positive response is achieved, these supranormal levels of cardiac output and CPP are defined as optimal. Caution is advised in patients with stunned myocardium for excessive MAP and CPP may result in increased left ventricular afterload and lead to reduced cardiac output and pulmonary congestion [40]. Angiography and definite treatment with intra-arterial vasodilators and balloon angioplasty are often necessary for refractory symptomatic vasospasm [41].
71.5.2 Traumatic Brain Injury (TBI)
A recent update of the Brain Trauma Foundation Guidelines for the management of patients with severe TBI addresses the evidence available on CPP management, including the role of brain tissue oxygenation and metabolism [37]. It suggests there is a clinical threshold of CPP, between 50 and 60 mmHg, below which cerebral blood flow is compromised and poor outcome is more likely. Studies have shown that CPP values below 60 mmHg are associated with low PbtO2 and jugular venous oxygen saturation and that these findings are related to poor outcome. Microdialysis studies have also suggested that ischemia, as measured by altered L/P ratios, is more frequent when CPP trends below 50 mmHg.
The guidelines’ update also emphasizes recent evidence from a randomized clinical trial comparing treatment based on CPP and ICP goals. CPP was kept above 70 mmHg in one group while the other group was treated to maintain ICP below 20-25 mmHg and avoid CPP <50 mmHg. There was no difference in outcome between the 2 groups and the CPP-based management group had a five-fold increased incidence of acute respiratory distress syndrome (ARDS) [42].
Similar results were found in a retrospective analysis of a RCT where CPP-targeted therapy was associated with ARDS and this complication was strongly related to vasopressor administration [43].
The body of evidence available suggests that finding an optimal CPP is crucial to the management of patients with TBI [37]. The optimal level should be individualized after assessing cerebral autoregulation, oxygenation, metabolic and electrical profiles and their responses to changes in CPP. We assess autoregulation in the acute phase after severe TBI through the moving correlations between MAP and ICP (pressure reactivity index) [44,45] and CPP and PbtO2 (oxygen reactivity index) [46]. Impaired autoregulation indicates that cerebral blood flow is dependent on CPP and that unnecessary high CPP levels may lead to increased cerebral blood volume and intracranial pressure. Initially we avoid CPP levels below 60 mmHg. Intracranial pressure levels above 25 mmHg are treated with sedatives/analgesics and osmolar therapy followed by hypothermia and barbiturates in refractory cases [37]. Concomitantly, optimal CPP is pursued.
Cardiac preload assessment always precedes efforts to increase systemic vascular resistance with vasopressors and myocardial contractility with inotropes. The goal is to avoid CVP <5 mmHg, keep GEDVi >600 ml/m2and maintain stroke volume or pulse pressure variation below 10%. Extreme caution to avoid unnecessary fluid overloading is warranted during the course of fluid resuscitation. We prefer using fluid boluses of crystalloid as needed instead of continuous infusion of large volumes of fluid. After every fluid challenge cardiac output improvement is reassessed. Ineffective fluid challenges will fail to increase cardiac output and thus cerebral blood flow, and contribute to pulmonary edema. Although we do not consider high extravascular lung water measurements as a contraindication to fluid administration, special caution is warranted in patients with values above 10 to 15 ml/kg [5,47].
Vasopressors and inotropes should be initiated for patients who fail to maintain minimum values of CPP (>60 mmHg) and cardiac output (>2.5 l/min/m2) after fluid resuscitation. The next step is to titrate CPP and CI to meet individual needs based on physiological information from multimodality monitoring. In patients that present with reduced PbtO2 and elevated L/P – less than 15 mmHg and above 40, respectively – despite hemodynamic stability, a trial of increased CPP and/or cardiac output is attempted [48,49]. Dynamic improvement of oxygenation and metabolism suggest that the supranormal values achieved are necessary to maintain brain homeostasis and avoid secondary injury.
71.5.3 Intracerebral Hemorrhage (ICH)
The presence and severity of acute hypertension may affect outcome after intracerebral hemorrhage (ICH). There is reasonable evidence that elevated admission BP is associated with increased risk of deterioration, death or dependency after ICH [22,29]. Though, the target BP to pursue and the drug of choice to achieve it are still a matter of debate. Recent guidelines suggest maintaining SBP below 160 mmHg and MAP below 110 mmHg in patients at risk for deterioration and high ICP during the acute phase after ICH [50]. We usually start a continuous infusion of nicardipine in all ICH patients with SBP greater than 160 mmHg or MAP greater than 110 mmHg.
Comatose patients with intracranial mass effect associated with the hematoma or perihematomal edema regularly undergo multimodal monitoring. The main objective is to achieve adequate BP control without compromising cerebral perfusion and metabolism. Some authors suggest there is a penumbra region around the area of the hematoma with impaired autoregulation and at risk for ischemia [51]. The monitoring probes are usually directed to the ipsilateral hemisphere in the perilesional region. This approach to probe placement may potentially detect regional hypopefusion and diagnose expansion of perihematomal edema.
Initial CPP is targeted between 60 mmHg and 80 mmHg and finer adjustments are made based on PbtO2 and microdialysis values. If CPP levels of 60 to 70 mmHg are tolerated without evidence of brain tissue hypoxia or metabolic distress MAP is titrated to achieve this goal with either vasopressors such as norepinephrine or vasodilators such as nicardipine. Although the relationship between hematoma growth and hypertension is still controversial, CPP levels above 80 mmHg are usually avoided in the first 72 hours post ICH and, when deemed necessary, titrated with extreme caution.
71.5.4 Post Cardiopulmonary Resuscitation
There is little evidence on CPP management in patients that survived a
cardiac arrest, especially after hypothermia was instituted as an evidenced-based treatment by the International Liaison Committee on Resuscitation (ILCOR) [52-54].
Furthermore there is negligible data and experience with intracranial multimodal monitoring of these patients [31].
Cardiac arrest causes a global ischemic injury to the brain followed, after return to spontaneous circulation (ROSC), by a potentially harmful reperfusion injury. Patients that survived cardiac arrest used to have devastating outcomes, especially those with delayed time to ROSC and non ventricular fibrillation rhythms [53,55]. Since hypothermia was incorporated to the armamentarium of treatment for these patients, improved outcomes have been consistently shown in clinical trials and real world case series, even for severely ill patients with cardiogenic shock and time to ROSC of up to 30 minutes [53].
Although hypothermia can potentially ameliorate the effects of a global hypoperfusion injury to the brain, we believe it should be part of a comprehensive approach to avoid secondary injury that includes systemic and cerebral hemodynamic management guided by multimodal monitoring. Continuous EEG and jugular bulb oxymetry monitoring have been shown to be associated with outcome after cardiopulmonary resuscitation [56-58]. Studies conducted before the hypothermia era suggest that the presence of non-convulsive status epilepticus (NCSE) and elevated jugular bulb saturation indicate poor prognosis. More extensive monitoring of cerebral function including intracranial pressure, tissue oxygenation, metabolism and perfusion may help titrate systemic drivers of cerebral blood flow such as CPP and cardiac output. All patients treated with hypothermia after cardiac arrest in our ICU undergo invasive arterial pressure, semi-invasive cardiac output and continuous EEG monitoring. Initial hemodynamic goals include euvolemia – optimized preload – with MAP above 80 mmHg and CI above 2.5. If post resuscitation myocardial stunning is present, patients may require vasopressor and inotropic support to achieve these goals and care should be taken not to overload the patient and cause impaired lung gas exchange. Once multimodal monitoring data is available hemodynamic variables are titrated to keep CPP >60 mmHg, ICP <20 mmHg, CBF >20 ml/100 g/min, PbtO2 >15 mmHg and L/P ratios <40. These levels are derived from other scenarios of severe brain injury and need to be tested in prospective trials of patients after cardiopulmonary resuscitation.
With increasing numbers of patients undergoing hypothermia treatment in the post resuscitation phase and more comprehensive monitoring of cerebral function, the pathophysiology of the ischemia-reperfusion injury caused by cardiac arrest will be better understood. This can potentially lead to specific approaches that avoid secondary injury during the hypothermia, rewarming and post rewarming phases. We believe that better understanding and targeted treatment can further improve outcomes of cardiac arrest survivors.
71.6 Conclusions
CPP management after severe brain injury is part of a comprehensive approach that includes goal-directed systemic and cerebral hemodynamic management. The first step should always be to achieve optimal preload and adequate volemic status through targeted fluid resuscitation. Vasopressor and inotropic support may be necessary at this early resuscitation phase to guarantee systemic end-organ perfusion.
Optimal CPP is then pursued. In general, the goal of achieving optimal CPP is to guarantee adequate delivery to meet the brain’s metabolic demands. CPP and cardiac output are major determinants of CBF. Thus, oxygen and nutrient delivery to the tissue are directly related to CPP and cardiac output. The purpose of multimodal monitoring is to allow goal-directed management of cardiac output and CPP guided by measures of brain tissue perfusion, oxygenation and metabolic function. This approach allows not only early detection of harmful complications but also guides treatment towards a healthier profile of brain function, potentially preventing secondary brain injury and improving patients’ outcomes.
References
1. Ostergaard M, Nielsen J, Rasmussen JP, et al. Cardiac output–pulse contour analysis vs. pulmonary artery thermodilution. Acta Anaesthesiol Scand 2006; 50:1044-9
2. de Waal EE, Kalkman CJ, Rex S, et al. Validation of a new arterial pulse contour-based cardiac output device. Crit Care Med 2007; 35: 1904-1909
3. Mayer J, Boldt J, Schollhorn T, et al. Semi-invasive monitoring of cardiac output by a new device using arterial pressure waveform analysis: a comparison with intermittent pulmonary artery thermodilution in patients undergoing cardiac surgery. Br J Anaesth 2007; 98: 176-82
4. Mayer J, Boldt J, Wolf MW, et al. Cardiac output derived from arterial pressure waveform analysis in patients undergoing cardiac surgery: validity of a second generation device. Anesth Analg 2008; 106: 867-72
5. Berkowitz DM, Danai PA, Eaton S, et al. Accurate characterization of extravascular lung water in acute respiratory distress syndrome. Crit Care Med 2008; 36: 1803-9
6. Vallee F, Vallet B, Mathe O, et al. Central venous-to-arterial carbon dioxide difference: an additional target for goal-directed therapy in septic shock? Intensive Care Med 2008; 34: 2218-25
7. Bakker J, Vincent JL, Gris P, et al. Veno-arterial carbon dioxide gradient in human septic shock. Chest 1992; 101: 509-15
8. Cuschieri J, Rivers EP, Donnino MW, et al. Central venous-arterial carbon dioxide difference as an indicator of cardiac index. Intensive Care Med 2005; 31: 818-22
9. Otero RM, Nguyen HB, Huang DT, et al. Early goal-directed therapy in severe sepsis and septic shock revisited: concepts, controversies, and contemporary findings. Chest 2006; 130: 1579-95
10. Rivers E, Nguyen B, Havstad S, et al. Early goal-directed therapy in the treatment of severe sepsis and septic shock. N Engl J Med 2001; 345: 1368-77
11. Waziri A AH, Oddo M, et al. Early experience with a cortical depth electrode for ICU neurophysiological moniroting. Epilepsia 2007; 48(Suppl 6): 208-9
12. Jaeger M, Soehle M, Schuhmann MU, et al. Correlation of continuously monitored regional cerebral blood flow and brain tissue oxygen. Acta Neurochir (Wien) 2005; 147: 51-6
13. Stewart C, Haitsma I, Zador Z, et al. The new Licox combined brain tissue oxygen and brain temperature monitor: assessment of in vitro accuracy and clinical experience in severe traumatic brain injury. Neurosurgery 2008; 63: 1159-64
14. Rose JC, Neill TA, Hemphill JC, 3rd. Continuous monitoring of the microcirculation in neurocritical care: an update on brain tissue oxygenation. Curr Opin Crit Care 2006; 12: 97-102
15. Rosenthal G, Hemphill JC, 3rd, Sorani M, et al. Brain tissue oxygen tension is more indicative of oxygen diffusion than oxygen delivery and metabolism in patients with traumatic brain injury. Crit Care Med 2008; 36: 1917-24
16. Rosenthal G, Hemphill JC, Sorani M, et al. The role of lung function in brain tissue oxygenation following traumatic brain injury. J Neurosurg 2008; 108: 59-65
17. Hillered L, Vespa PM, Hovda DA. Translational neurochemical research in acute human brain injury: the current status and potential future for cerebral microdialysis. J Neurotrauma 2005; 22: 3-41
18. Bellander BM, Cantais E, Enblad P, et al. Consensus meeting on microdialysis in neurointensive care. Intensive Care Med 2004; 30: 2166-9
19. Barth M, Capelle HH, Munch E, et al. Effects of the selective endothelin A (ET(A)) receptor antagonist Clazosentan on cerebral perfusion and cerebral oxygenation following severe subarachnoid hemorrhage – preliminary results from a randomized clinical series. Acta Neurochir (Wien) 2007; 149: 911-918
20. Nguyen HB, Rivers EP, Knoblich BP, et al. Early lactate clearance is associated with improved outcome in severe sepsis and septic shock. Crit Care Med 2004; 32: 1637-42
21. Michard F, Teboul JL. Predicting fluid responsiveness in ICU patients: a critical analysis of the evidence. Chest 2002; 121: 2000-8
22. Davis SM, Broderick J, Hennerici M, et al. Hematoma growth is a determinant of mortality and poor outcome after intracerebral hemorrhage. Neurology 2006; 66: 1175-81
23. Vespa PM, Miller C, McArthur D, et al. Nonconvulsive electrographic seizures after traumatic brain injury result in a delayed, prolonged increase in intracranial pressure and metabolic crisis. Crit Care Med 2007; 35: 2830-6
24. Vespa P. Continuous EEG monitoring for the detection of seizures in traumatic brain injury, infarction, and intracerebral hemorrhage: “to detect and protect”. J Clin Neurophysiol 2005; 22: 99-106
25. Claassen J, Jette N, Chum F, et al. Electrographic seizures and periodic discharges after intracerebral hemorrhage. Neurology 2007; 69: 1356-65
26. Claassen J, Hirsch LJ, Frontera JA, et al. Prognostic significance of continuous EEG monitoring in patients with poor-grade subarachnoid hemorrhage. Neurocrit Care 2006; 4: 103-12
27. Claassen J, Mayer SA, Hirsch LJ. Continuous EEG monitoring in patients with subarachnoid hemorrhage. J Clin Neurophysiol 2005; 22: 92-8
28. Claassen J, Peery S, Kreiter KT, et al. Predictors and clinical impact of epilepsy after subarachnoid hemorrhage. Neurology 2003; 60: 208-14
29. Broderick JP, Diringer MN, Hill MD, et al. Determinants of intracerebral hemorrhage growth: an exploratory analysis. Stroke 2007; 38: 1072-5
30. Wright WL, Geocadin RG. Postresuscitative intensive care: neuroprotective strategies after cardiac arrest. Semin Neurol 2006; 26: 396-402
31. Nordmark J, Rubertsson S, Mortberg E, et al. Intracerebral monitoring in comatose patients treated with hypothermia after a cardiac arrest. Acta Anaesthesiol Scand 2009; 53: 289-98
32. Lescot T, Abdennour L, Boch AL, et al. Treatment of intracranial hypertension. Curr Opin Crit Care 2008; 14: 129-34
33. Claassen J, Hirsch LJ, Kreiter KT, et al. Quantitative continuous EEG for detecting delayed cerebral ischemia in patients with poor-grade subarachnoid hemorrhage. Clin Neurophysiol 2004; 115: 2699-710
34. Johnston AJ, Steiner LA, Coles JP, et al. Effect of cerebral perfusion pressure augmentation on regional oxygenation and metabolism after head injury. Crit Care Med 2005; 33: 189-95
35. White H, Venkatesh B. Cerebral perfusion pressure in neurotrauma: a review. Anesth Analg 2008;107: 979-88
36. Diringer MN, Axelrod Y. Hemodynamic manipulation in the neuro-intensive care unit: cerebral perfusion pressure therapy in head injury and hemodynamic augmentation for cerebral vasospasm. Curr Opin Crit Care 2007; 13: 156-62
37. Bratton SL, Chestnut RM, Ghajar J, et al. Guidelines for the management of severe traumatic brain injury. X. Brain oxygen monitoring and thresholds. J Neurotrauma 2007; 24(Suppl 1): S65-70
38. Bederson JB, Connolly ES Jr, Batjer HH, et al. Guidelines for the management of aneurysmal subarachnoid hemorrhage: a statement for healthcare professionals from a special writing group of the Stroke Council, American Heart Association. Stroke 2009; 40: 994-1025
39. Belli A, Sen J, Petzold A, et al. Metabolic failure precedes intracranial pressure rises in traumatic brain injury: a microdialysis study. Acta Neurochir (Wien) 2008; 150: 461-9
40. Naidech AM, Kreiter KT, Janjua N, et al. Cardiac troponin elevation, cardiovascular morbidity, and outcome after subarachnoid hemorrhage. Circulation 2005; 112: 2851-6
41. Diringer MN. Management of aneurysmal subarachnoid hemorrhage. Crit Care Med 2009.
42. Robertson CS, Valadka AB, Hannay HJ, et al. Prevention of secondary ischemic insults after severe head injury. Crit Care Med 1999; 27: 2086-95
43. Contant CF, Valadka AB, Gopinath SP, et al. Adult respiratory distress syndrome: a complication of induced hypertension after severe head injury. J Neurosurg 2001; 95: 560-8
44. Steiner LA, Coles JP, Johnston AJ, et al. Assessment of cerebrovascular autoregulation in head-injured patients: a validation study. Stroke 2003; 34: 2404-9
45. Steiner LA, Coles JP, Czosnyka M, et al. Cerebrovascular pressure reactivity is related to global cerebral oxygen metabolism after head injury. J Neurol Neurosurg Psychiatry 2003; 74: 765-70
46. Jaeger M, Schuhmann MU, Soehle M, et al. Continuous assessment of cerebrovascular autoregulation after traumatic brain injury using brain tissue oxygen pressure reactivity. Crit Care Med 2006; 34: 1783-8
47. Huber W, Umgelter A, Reindl W, et al. Volume assessment in patients with necrotizing pancreatitis: a comparison of intrathoracic blood volume index, central venous pressure, and hematocrit, and their correlation to cardiac index and extravascular lung water index. Crit Care Med 2008; 36: 2348-54
48. Joseph M, Ziadi S, Nates J, et al. Increases in cardiac output can reverse flow deficits from vasospasm independent of blood pressure: a study using xenon computed tomographic measurement of cerebral blood flow. Neurosurgery 2003; 53:1044-51
49. Muench E, Horn P, Bauhuf C, et al. Effects of hypervolemia and hypertension on regional cerebral blood flow, intracranial pressure, and brain tissue oxygenation after subarachnoid hemorrhage. Crit Care Med 2007; 35: 1844-51
50. Broderick J, Connolly S, Feldmann E, et al. Guidelines for the management of spontaneous intracerebral hemorrhage in adults: 2007 update: a guideline from the American Heart Association/American Stroke Association Stroke Council, High Blood Pressure Research Council, and the Quality of Care and Outcomes in Research Interdisciplinary Working Group. Circulation 2007; 116: e391-413
51. Kim-Han JS, Kopp SJ, Dugan LL, et al. Perihematomal mitochondrial dysfunction after intracerebral hemorrhage. Stroke 2006; 37: 2457-62
52. Bernard SA, Gray TW, Buist MD, et al. Treatment of comatose survivors of out-of-hospital cardiac arrest with induced hypothermia. N Engl J Med 2002; 346: 557-63
53. Oddo M, Ribordy V, Feihl F, et al. Early predictors of outcome in comatose survivors of ventricular fibrillation and non-ventricular fibrillation cardiac arrest treated with hypothermia: a prospective study. Crit Care Med 2008; 36: 2296-301
54. Jacobs I, Nadkarni V, Bahr J, et al. Cardiac arrest and cardiopulmonary resuscitation outcome reports: update and simplification of the Utstein templates for resuscitation registries. A statement for healthcare professionals from a task force of the international liaison committee on resuscitation (American Heart Association, European Resuscitation Council, Australian Resuscitation Council, New Zealand Resuscitation Council, Heart and Stroke Foundation of Canada, InterAmerican Heart Foundation, Resuscitation Council of Southern Africa). Resuscitation 2004; 63: 233-49
55. Oddo M, Schaller MD, Feihl F, et al. From evidence to clinical practice: effective implementation of therapeutic hypothermia to improve patient outcome after cardiac arrest. Crit Care Med 2006; 34: 1865-73
56. Rossetti AO, Logroscino G, Liaudet L, et al. Status epilepticus: an independent outcome predictor after cerebral anoxia. Neurology 2007; 69: 255-60
57. Buunk G, van der Hoeven JG, Meinders AE. Prognostic significance of the difference between mixed venous and jugular bulb oxygen saturation in comatose patients resuscitated from a cardiac arrest. Resuscitation 1999; 41: 257-62
58. Wijdicks EF, Hijdra A, Young GB, et al. Practice parameter: prediction of outcome in comatose survivors after cardiopulmonary resuscitation (an evidence-based review): report of the Quality Standards Subcommittee of the American Academy of Neurology. Neurology 2006; 67: 203-10
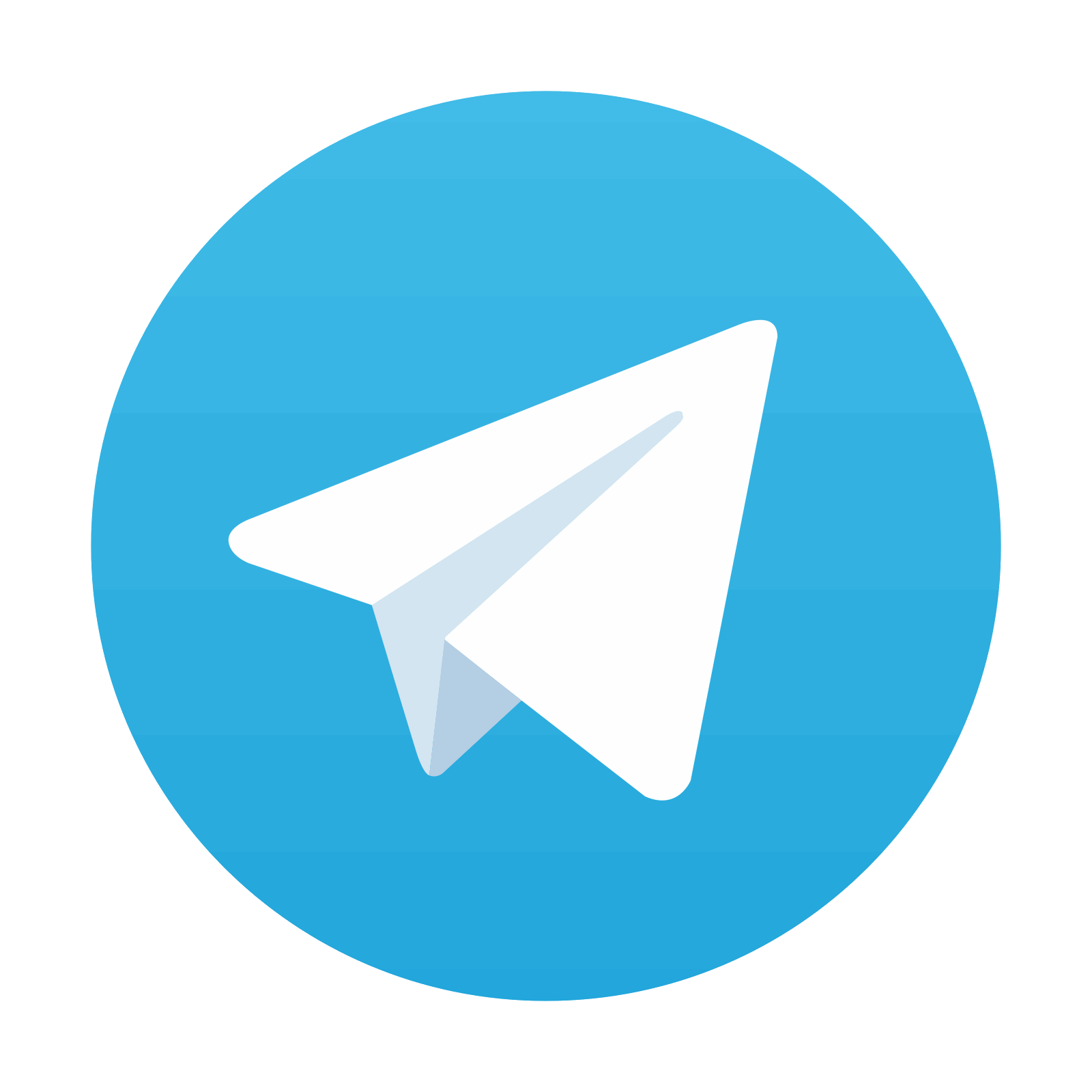
Stay updated, free articles. Join our Telegram channel

Full access? Get Clinical Tree
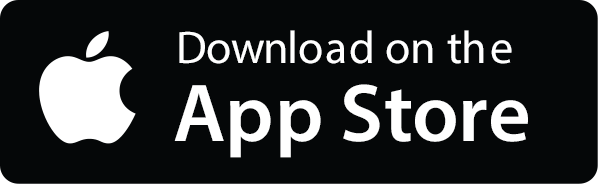
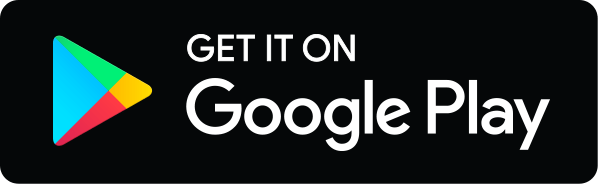