Chapter 126 Pain and Sleep
Pain and Sleep
As defined by the International Association for the Study of Pain,1 pain is an “unpleasant sensory and emotional experience associated with actual or potential tissue damage or described in terms of such damage.” The emotional dimension is concomitant with both acute pain (e.g., postoperative) and chronic pain (e.g., arthritic pain, herpetic neuralgia, diabetic neuropathy). In the presence of acute pain, such as a toothache, relief is predictable with appropriate dental care, and the consequences for quality of life and health are usually short term. When pain becomes chronic—that is, if it lasts more than several weeks or months—the patient may report poor quality of life and poor daytime performance at work or familial tasks. For some patients, day-to-day life with intense pain is so unbearable that a suicide attempt is possible if the distress associated with suffering is not managed.2 The sudden reappearance of a pain episode can be a sign of the recurrence of a potentially fatal disease (e.g., cancer pain), with its attendant effects on the patient’s well-being, anxiety, and sleep quality.
Interest in the interaction between pain and sleep emerged in the 1960s, with descriptions of the processing of tactile cutaneous sensory inputs at the level of the brainstem mesencephalic–reticular formation and the trigeminal sensory nucleus during wakefulness and sleep.3 Clinical interest began in the late 1960s with a series of publications suggesting that patients with chronic pain could demonstrate disturbed sleep homeostasis. At that time, the emerging concept of electroencephalographic (EEG) instability—referred to as alpha intrusions during non–rapid eye movement (NREM) deep sleep—was associated with somatic pain.4
Clinically, both linear and circular relationships exist between pain and sleep, and pain can precede the poor sleep complaints, or vice versa (Fig. 126-1). With acute pain, the poor sleep complaints usually do not continue after pain resolution. However, the initial linear sequence (i.e., pain precedes poor sleep) can be transformed, in some patients, into a vicious-cycle pattern when pain lasts too long (i.e., a day with intense pain followed by a night with poor sleep quality, and a night with poor sleep followed by a higher degree of pain perception or pain reports on the next day).5 The linear relationship was reported by 53% to 89% of patients with various types of musculoskeletal pain.6,7 The vicious cycle is reported for patients with chronic pain such as fibromyalgia or severe skin burns.8–10 Recent epidemiologic data have shown a reciprocal relationship between sleep duration and frequency of next-day pain in the general population. Specifically, transitioning from a night of relatively normal sleep duration (6 to 9 hours) to a night of less than 6 hours or greater than 9 hours was linked to a substantial increase in next-day pain frequency. Pain during the day also predicted alterations in sleep duration at night.11
Pain
Pain is a protective response, involving neuronal, glial, and immune cellular activities (e.g., gene expression, ionic channel and receptor activation), to integrated behavior such as the fight-or-flight reaction, with the activation of several limbic structures such as the hypothalamus, amygdala, cingulate, and frontal cortical areas.3,3a,12–14 Chronic pain may also be considered a disorder of the nervous system; when it is persistent, there are reversible or permanent changes associated with neuronal plasticity, or there are modifications at the cellular or network level (both neuronal and glial cells with immune responses). Pain is associated with direct nerve activation (e.g., trigeminal neuralgia), with inflammation (e.g., arthritis), with changes in nerve environment (e.g., neuropathic pain), or with changes at the cortical sensory representation areas (enlargement of the sensory cortical territory, a reverberating loop that potentiates sensory nonpain inputs to a frequency that is interpreted as pain). In summary, with most somatic pain, there is a usual sequence of activation from nociception to pain perception that involves the following: (1) activation of nerve endings, (2) transmission through sensory A delta and C sensory fibers, (3) a first relay at spinal cord neurons, (4) a second relay at thalamic levels and interactions with brainstem cells, (5) cortical neuronal network activation, and (6) the final reaction to pain, such as withdrawal or escape behaviors with or without emotional reactions such as tearing, crying, or anxiety.
Epidemiology and Risk Factors
The costs of pain and of poor sleep are in the billions of dollars in North America, but no specific cost assessment has been done for the cumulative impact of pain and poor sleep together.15 Comorbidities are a dominant feature of the pain and sleep interaction. A survey done in a primary care family practice network revealed that chronic pain, including arthritis and joint pain, was associated with complaints of being sleepy during daytime activities, of symptoms suggestive of restless legs syndrome, and of loud snoring and cessation of breathing during sleep (risk odds ratio [OR], 1.7 to 2.7).16 Clinicians involved in the diagnosis and management of complaints of pain and poor sleep quality appreciate the relevance of the interaction between the two conditions. In a general population survey, it was noted that the frequency of a day’s pain symptoms can be predicted by whether an individual sleeps less than 6 hours or more than 9 hours.11 Short or long sleep duration (either end of a U-shaped distribution) was also reported to be associated with health risks.17
The prevalence of chronic pain in the adult population is estimated to be 11%, but figures as high as 29% have been reported depending on the questions asked to the target population.18 Between 50% and 70% of adults and children with chronic pain reported poor sleep in relation to their pain conditions, which include arthritis, cervical pain, fibromyalgia, orofacial joint and muscle pain (temporomandibular pain), and low back pain.6,7,19–21 Other medical conditions with concomitant pain that are also reported to interfere with sleep quality are headaches, irritable bowel syndrome, spinal cord injury, traumatic brain injury, and metastatic breast cancer.4,22–26
A risk factor that contributes to the perpetuation of poor sleep is chronic pain, with a low OR of approximately 1.5 (i.e., 44% of patients with chronic pain report insomnia, versus 19% of subjects without pain).4 Concomitant conditions such as anxiety, fatigue, mood disturbances, depression, and poor physical fitness also affect the interaction between pain and sleep.
Sleep and pain interactions are influenced by multiple factors. To a large extent, the data are derived from surveys (e.g., simple questions or questionnaires on sleep, mood, pain) of general populations or of patient cohorts, in which pain intensity and sleep quality reports are subjective. About 30% of the interactions between pain and sleep (i.e., from poor sleep quality to insomnia) can be explained by the presence of concomitant fatigue, depression or mood alteration, poor physical condition, and anxiety.7,21,22,27,28 A recent comparison between women suffering from fatigue in the presence of osteoarthritis, rheumatoid arthritis, or fibromyalgia revealed that in the women with rheumatoid arthritis or fibromyalgia, there was a relationship between daily pain and fatigue.29 Insomnia complaints also tended to be related to lower scores on physical and emotional pain and to vitality domains when the 36-item Short-Form Health Survey (SF-36) was administered to older patients.30 Gender and aging are also concomitant variables. Chronic pain is more frequently reported by women, at approximately double the rate in men. With age, pain becomes more prevalent (e.g., in 25% to 50% of patients older than 55 years) and lasts longer, although older patients seem to cope better with pain and tend to be more stoic.30,31
PLM during sleep and sleep breathing disorders are other important variables to control for when studying the effect of age (see Chapters 90, Chapter 100 to Chapter 105). We recently observed that patients with chronic widespread pain have four times more PLM per hour of sleep than matched controls, and the presence of sleep breathing conditions (snoring, upper airway resistance, apnea–hypopnea syndrome) was also reported to be a common finding in patients with chronic pain.16,32–34 This suggests that the interaction between aging, pain, and poor sleep complaints depends on several variables.30
Pathogenesis
The interaction between pain and poor sleep could be partially explained by sleep fragmentation (higher frequency of arousals or sleep stage shifts) and by a lower percentage of slow-wave sleep (SWS) (i.e., stages 3 and 4, or deep or restorative sleep). Indeed, most patients with chronic pain report daytime fatigue and sleepiness, reduced cognitive function (e.g., memory impairment), and poor quality of life. So far, no simple mechanism explains the pathophysiology of the interaction between pain and poor sleep quality, or the presence of the vicious-cycle pattern found in some patients with chronic pain (see Fig. 126-1). Pain perception and modulation are altered in relation to sleep state. In general, sensory information is filtered during sleep, but relevant inputs threatening body homeostasis may trigger a rapid return of consciousness, with the patient reporting pain on awakening.
Neurobiological Basis of Sensory-Pain Perception in Sleep
Recent animal studies have confirmed that in the presence of brief sensory or pain stimulations during sleep, the spinoreticular and trigeminothalamic tract neurons (part of the ascending sensory and pain pathways) behave as a gated control mechanism to trigger or inhibit an awakening response. Such activity is not homogeneous across sleep stages (quiet NREM or active REM sleep) and seems to depend on brainstem raphe magnus cell activity (serotonergic or not).35,36 Moreover, the latency of the pain-related sensorimotor reflex, the tail flick, allows a sensitive assessment of spinal nociception. Compared with in wakefulness, the reflex latency in cats is increased threefold in NREM and fivefold in REM sleep.37 Based on animal and human findings, it is now accepted that a sleeping brain is not completely isolated from the external milieu, and that neuronal sensorimotor networks related to vigilance and body protection remain active in sleep.35,38
In humans, the perception of pain during sleep depends on the type of sensory stimulation. Differing stimuli—brief sensory nerve, midduration thermal, or long chemical or mechanical stimulations—produce very different outcomes, from spinal reflex to integrated cortical response and autonomic sleep arousal-awakening, all the way up to conscious awareness of stimulation.39–41 The spinal nociceptive reflex is a short-loop spinal reflex triggered by intense and brief electrical stimulation of the sural nerve area. Compared with wakefulness, the latency of this reflex is longer in deep NREM sleep and REM sleep, with its threshold significantly increased by 60% in NREM and by 200% in REM sleep.39 Laser stimulations in the milliseconds range, sufficient to evoke a brief cortical potential during the waking state, produce an observable cortical response during sleep stage 2 and REM.40 Midduration (6- to 12-second) thermal pain stimulations, causing a pain sensation with an intensity of 6 on a 10-point scale during wakefulness, triggered clear cortical arousals to approximately 50% of stimulations in stage 2 sleep, and responses to only approximately 30% of stimulations in SWS (stages 3 and 4) or REM sleep. These data indicate that the nociceptive temperature threshold is higher in SWS and REM sleep.41
Another pain model infuses hypertonic saline (5%) into muscle to evoke during wakefulness a pain intensity of 3 of 10 for more than 70 seconds. Using this model, where experimental pain mimics a muscle cramp, clear awakening responses are produced with a similar response rate across sleep stages; interestingly, most patients remembered a pain experience during their sleep, in contrast to the thermal stimulation experiments described previously.42,43 Again, available data demonstrating how ongoing sleep is interrupted by clinical pain are very limited.
Circadian Variation in Pain Perception
In healthy subjects, most studies suggest that pain perception to experimental thermal stimulation, chemical infusion, or mechanical pressure does not show variation over a 24-hour period or between evening and morning measurements when data are controlled for mood state and sex.41,44 However, patients’ self-reports of pain intensity vary with daytime symptoms. For example, patients with arthritis report that pain is worst in the morning with body motion. About 50% to 80% of those with muscle-related pain, including headaches, report higher pain intensity in the afternoon and evening, whereas pain from torticollis is completely relieved by sleep.45,46
Cognitive Impairment, Sleep Deprivation, and Pain Perception
Regardless of its cause, sleep fragmentation is associated with a subsequent increase in sleepiness and fatigue and a decrease in cognitive and motor performance. Effects of pain on sleep and cognition may therefore be nonspecific and mediated simply by the degree to which pain causes sleep fragmentation.4,47
Few studies suggest that memory and attention are impaired in patients with chronic musculoskeletal pain and fibromyalgia.48,49 More studies are needed to clarify the cognitive changes associated with chronic pain and poor sleep, taking into consideration the impact of sleep fragmentation and of concomitant anxiety, mood dysfunction, fatigue, and the like. Development or validation of more naturalistic tests related to real-life working or driving performance may provide new information on the cognitive deficit related to the interaction of pain and sleep or secondary to medication prescribed in its management.15,50 Moreover, all sleep stages seem to be necessary for memory consolidation, and a nap of at least 60 to 90 minutes offers similar advantages.51,52 In studying the interaction of pain and sleep, it is important to control for both napping and sleep continuity in assessing poor sleep quality in patients with chronic pain when daytime napping habits are unknown.47
To study the short-term effects on pain sensitivity and clinical pain reports, several studies (using relatively small samples) have disrupted or fragmented sleep, selectively abolished time spent in various sleep stages, curtailed total sleep time, or implemented total sleep deprivation procedures.53 Although the sleep manipulation methods were heterogeneous and the studies varied in the quality of the experimental design and the extent to which confounders were controlled, most reported that sleep loss and disruption produced significant hyperalgesia or induced spontaneous pain reports in healthy subjects. Earlier work suggested that SWS loss versus REM deprivation was differentially hyperalgesic,54,55 but those findings have not always been replicated.56 Data exist that show that thermal pain sensitivity is enhanced by both SWS deprivation and forced awakenings during REM sleep.56,57
Not enough studies have been done using the same sleep disruption techniques or pain testing modalities to clearly specify which type of sleep disruption, and what degree of it, causes which type of alterations in pain sensitivity. Future sleep deprivation studies need to account for the complex interactions between fatigue, cognitive function, Physiological dysregulation, and pain. The mechanisms by which sleep deprivation contributes to pain remain largely unknown; they are most likely multiple and determined by complex interacting factors. A study of 6 days of sleep restriction is associated with elevated neuroimmune markers, including proinflammatory cytokines, specifically interleukin (IL)-6, which are known to sensitize nociceptors.58 In another experiment, 3 nights of sleep continuity disturbance (multiple prolonged forced awakenings) impairs psychophysical measures of endogenous pain inhibition and increases spontaneous clinical pain reports in healthy young women.59 Descending pain inhibitory mechanisms are believed to be mediated in part by opioidergic and serotoninergic pathways in the brainstem, which are in turn regulated by multiple higher cortical structures including the anterior cingulate and regions of the frontal cortex (see Chapter 18).
Aging, Pain Perception, and Dysfunctional Endogenous Analgesia
With age, pain complaints increase and pain perception is altered but, paradoxically, older patients are reported to cope better with the consequences of chronic pain than middle-aged subjects.60,61 Pain sensitivity is lower and the pain threshold higher probably because of age-related changes in tissues (e.g., reduced thickness, changes in microcirculation). In addition, there is lower cerebral discrimination of sensory information (processing at spinal cord or cortical levels). Despite increases in pain threshold, older adults demonstrate impairments in higher-order pain modulatory capacity (diffuse noxious inhibitory controls [DNIC]).62 The DNIC system is an upper-brain function that reduces pain perception in the whole body through the activation of neurochemicals (e.g., opioids) in distant body areas, in a nonselective distribution (i.e., a diffuse general reduction in pain threshold is found). As demonstrated by experimental manipulations, DNIC activation is apparently dysfunctional in patients with fibromyalgia.63 This is further supported by evidence that in the presence of generalized pain, such as in patients with fibromyalgia, an abnormal sensitization (e.g., generalized body pain) and abnormal temporal summation of pain are dominant.64 Recent evidence suggests that the DNIC system is dysfunctional in temporomandibular pain patients with poor sleep.65
Alpha Electroencephalogram Intrusions, Phasic Arousal, and Autonomic Activity
Alpha EEG intrusions were found in several sleep studies of patients with fibromyalgia, but more recent controlled and quantitative scoring failed to support the notion that alpha EEG intrusions were pain or fibromyalgia specific.22,66,67 The current trend is to reassess such findings in the setting of mood alterations, psychiatric conditions (e.g., insecure attachment, hypervigilance), and sleep respiratory and movement events (e.g., apnea, PLM), and to reconcile them with the fact that patients with pain have arousals in clusters or in a sequence of phasic events, also termed the cyclic alternating pattern.4,20,68–70
Neurochemistry, HPA Axis, and Genetics in the Interaction between Pain and Sleep
There is indirect evidence that pain modulatory systems may be altered in the context of chronic pain and poor sleep. Animal findings suggest that serotoninergic and nonserotoninergic brainstem neurons modify alertness in relation to the raphe magnus “off” cells, which prevent arousal in response to external sensory stimulations such as pain.36 Surprisingly, almost no evidence links the “alertness” or vigilance network (i.e., ascending reticular activating system) to changes in cholinergic, dopaminergic, gamma-aminobutyric acid, or adrenergic neuronal reticular and hypothalamic activity in relation to alterations in sleep homeostasis in the presence of chronic pain.37,71
Although hypothalamic orexinergic system—an important axis of vigilance—was suggested to be involved in pain and headache, the level of information in humans is rather low.72 More specifically, it is unknown whether hypocretin (orexin) gene activity and Clock gene expression are altered in the presence of chronic pain with secondary sleep alterations.73 Special interest has also been devoted to growth hormone because its release is associated with SWS. However, the evidence is limited that supports an alteration in serum levels of insulin-like growth factor (formerly called somatomedin C) in patients with fibromyalgia or other pain.74,75
There is evidence to suggest multiple interactions between pain and sleep in relationship to the arousal parameter hypothalamic-pituitary-adrenal axis (HPA) and the immune system. Prediction of new cases of chronic widespread musculoskeletal pain (OR-3) was possible with the dexamethasone cortisol suppression test and measures of evening and morning salivary cortisol.76 Insomnia, a frequent finding in pain patients,27 was also found to be associated with a rise in HPA, adrenocorticotropic hormone, cortisol secretion, and inflammatory cytokines IL-6 and tumor necrosis factor α.77 Deprivation of sleep in normal subjects also revealed a high correlation between insufficient sleep (4 instead of 8 hours) and pain rating, with a rise in IL-6.58 In the general population, nociception dysregulation is rarely hereditary and was reported to be modulated by genetic polymorphism such as opioid (Oprm gene), catecholamine-O-methyltransferase (COMT), interleukin-related genes (e.g., for IL-1), and others.14 Interestingly, COMT is associated with sleepiness in patients with Parkinson’s disease and narcolepsy.78,79 Some animal findings suggest that morphine dependence is linked to orexin gene expression.80 IL-1 gene expression was also found to interfere with circadian Clock genes in mice.81 Before concluding, however, that such findings can be extrapolated to explain the cause and effect of the circular relationship of pain and sleep, much more integrated research needs to be done.
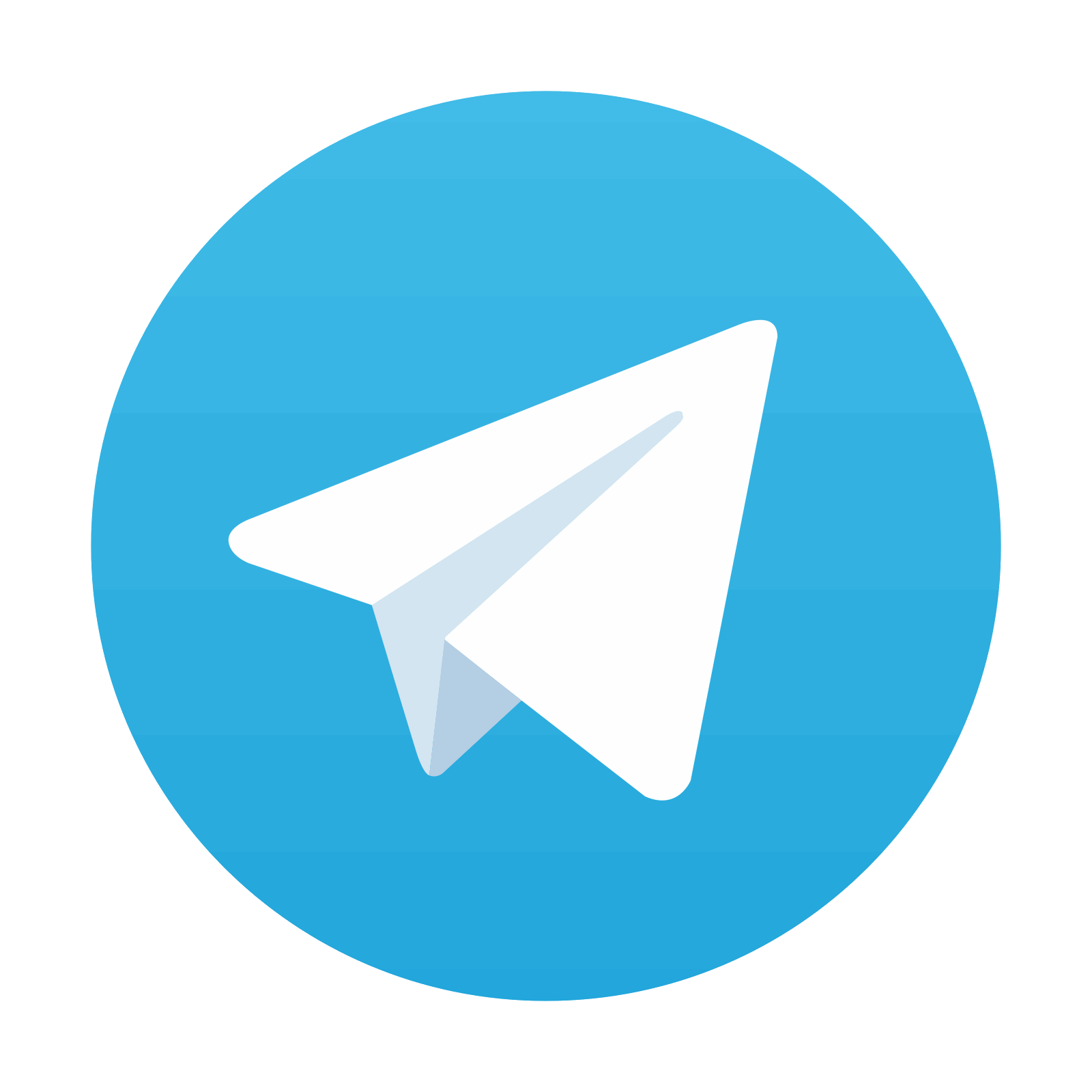
Stay updated, free articles. Join our Telegram channel

Full access? Get Clinical Tree
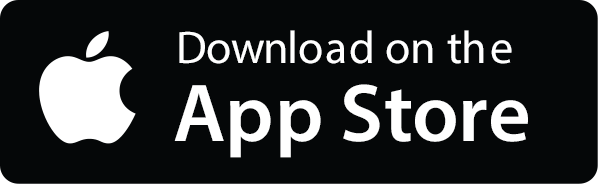
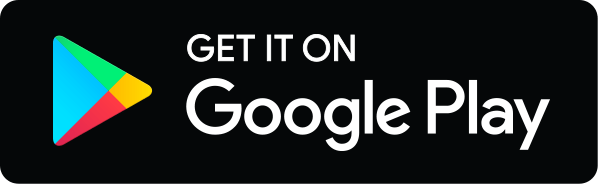