Figure 74.1. Minutely Heart Rate in an untreated dysautonomic subject (heart rates are minutely values derived from a 24 hour Holter monitor recording).
With increasing time post injury, paroxysms decrease in duration, frequency and magnitude. The reduction in severity of paroxysms usually coincides with an observable improvement in neurological status [5,7], although whether this is causative or an epiphenomena has not been identified. As subjects improve, paroxysms become rarer and resting blood pressure, respiratory rate, heart rate and temperature trend towards normal values.
While TBI accounts for an estimated 80% of published cases of PSH, many other neurological disorders have been reported to cause PSH. These include (in order of decreasing frequency) severe hypoxic brain injury, stroke (hemorrhagic stroke in particular), acute hydrocephalus and tumors. There are also isolated case reports of uncommon etiologies, such as PSH following infection [8] and hypoglycemic coma [9]. However, despite the wide range of etiologies associated with the disorder, the limited available data suggests that the syndrome itself is equivalent irrespective of the causative condition.
74.2 Pathophysiology
The earliest theories for PSH suggested that seizure activity was the likely etiology [10]. For this reason the syndrome was termed diencephalic epilepsy. However, multiple attempts to either identify or treat epilepsy in these patients have produced negative results (for example [7,11-14] The limited autopsy and pathophysiological data suggest the syndrome results from a relative disconnection of pathways at or around the level of the midbrain (reviewed in [15]).
Most disconnection theories suggest that paroxysms are driven by upper brainstem and diencephalon lesions, with clinical features arising from either excitatory or inhibitory centers in these areas. However, more recent evidence suggests that spinal cord processes are instrumental in initiating the condition. Two studies have assessed the effect of standardized nociceptive stimuli in driving paroxysms. In the first study, endotracheal tube suctioning of severe TBI survivors on day 7 post injury found that PSH subjects had heart rate increases of twice the magnitude (16% vs. 8% averaged over 100 beats) and of longer duration when compared to non-PSH subjects [16]. In a separate study, subjects with PSH a mean of 5 years post injury (the majority of whom were no longer exhibiting paroxysms) showed disproportionately excessive sympathetic responses to the nociceptive stimulus of botulinum toxin A injections [17]. These studies provide empirical support to anecdotal reports dating back to 1954 [18] that multiple physical stimuli can provoke sympathetic paroxysms.
When considered as a whole, the literature suggests that PSH results from a combination of both cerebral and spinal cord changes. A theoretical model, the Excitatory/Inhibitory Ratio (EIR) model, has been proposed to promote testing of the interaction of spinal and cerebral influences on PSH [19]. This model highlights the previously noted physiological similarities of PSH and autonomic dysreflexia [20,21] (that is, sympathetic over-responsivity to stimuli resulting from the loss of supraspinal inputs) and combines them under a common pathophysiological process. This integrative model suggests that the causative brainstem centers are predominantly inhibitory in nature, and that damage to these structures predisposes the spinal cord to overreact to afferent stimuli in a manner akin to that which produces allodynia (where non-painful stimuli become perceived as nociceptive) [22].
74.3 The Clinical Relevance of PSH
In injury severity matched cohorts, prolonged PSH is associated with worse outcome following severe TBI [5,8]. Such individuals have been found to have prolonged swallowing abnormalities, longer duration of coma and post traumatic amnesia [5]. In addition, subjects with PSH often display features consistent with “locked-in” syndromes [2,23], a situation that can result in under-managed pain or a misdiagnosis of persistent vegetative state. Affected individuals show reduced capacity for voluntary movement, particularly during paroxysms, and may be exacerbated by under-managed spasticity and dystonia [5]. In addition, posturing subjects show increased metabolic rates [24,25] resulting in a highly catabolic state [26] and an estimated 25% loss of body weight in the first weeks post injury [2]. Affected individuals have also been found to be significantly more likely to develop heterotopic ossification [27]. These factors combine to place the individual at risk of developing critical illness or compression neuropathies, longer hospital admissions [2,5] and greater overall health care costs [2]. It has been argued that early identification of PSH may minimize unnecessary diagnostic testing [28], promote timely and appropriate intervention [29]. and minimize secondary morbidity [5,30].
As recovery continues, posturing patterns may change, revealing an underlying spastic tetraplegia or other focal neurological deficit. Most subjects are left with some permanent degree of dystonia and spasticity [2]. Sweating patterns change from whole body to upper trunk, head and neck, before ceasing entirely [31]. Three studies have reported changes in sweating in a longitudinal fashion. In two of these, paroxysmal diaphoresis resolved a mean of 2.5 months post injury in 35 subjects [5] and 5.9 months post-injury in 87 subjects [8]. In another study, 22 of 31 subject’s features had settled by 6 months post injury [32]. While the overt features of sympathetic hyperactivity settle over time, the tendency to display paroxysmal tachycardias remains evident for at least 14 months post injury [30].
74.4 Management
Despite the impressive nature of the symptomatology and the largely circumstantial evidence promoting the importance of early intervention, there is surprisingly little literature available to guide management [4,21]. As the diagnosis of PSH is currently one of exclusion, other primary disease processes such as acute hydrocephalus and sepsis need to be considered and treated. Various autonomic emergency syndromes with similar symptom complexes (for example, malignant hyperthermia and neuroleptic malignant syndrome) should be ruled out. A careful examination for evidence of triggering [16] and treatment of potential noxious stimuli (such as undiagnosed fractures, heterotopic ossification, pressure areas, painful spasticity or dystonias, infection, etc.) may decrease the frequency or severity of paroxysms [21,33]. In this context, one author has suggested pre-treatment of patients prior to nociceptive procedures being undertaken to reduce “triggering” of paroxysms [33].
In spite of these possible interventions, conservative measures alone are rarely sufficient to control the paroxysmal sympathetic overactivity in severe cases. Although a large number of medications have been used to treat the condition, information regarding their efficacy is largely restricted to anecdotal reports and has been reviewed elsewhere [3,4,21,34]. Accepting the limitations of current literature, a range of medications from many classes of neurologically active agents may be beneficial. In particular, these medications include alpha and beta antagonists, dopamine agonists, GABA A and B agonists, opioids and gabapentin.
The most effective intervention appears to be intrathecal baclofen (ITB), with anecdotal reports of a large reduction or cessation in paroxysmal sympathetic overactivity [29,35-38] However, deciding when to consider implementing ITB therapy can be difficult. Many subjects will exhibit paroxysmal overactivity lasting for only a few months [5,27], the procedure is invasive and has a relatively high complication rates (20-50% [39]). On the other hand, ITB is also effective for managing spasticity [40] and this may have long-term advantages in such patients. Intravenous morphine and midazolam are also effective, but their sedative effects limit their usefulness during rehabilitation [21].
Gabapentin has been reported to reduce the number and severity of sympathetic paroxysms [9,29] and allowed an overall reduction in other medications, including ITB, without a recurrence of symptoms [29]. However, the drug should be trialed at a low starting dose (e.g., 100 mg) to minimize the risk of marked bradypnea or sedation, presumed to result from a sudden loss of sympathetic drive [14].
Drugs with sympathetic activity have also been commonly used. Of the beta-blockers, propanolol has the largest literature base, with mixed reports between those where it has been useful and where it has been unable to control paroxysms [4]. Recent research suggests that beta-blockers improve survival post TBI [41] and propanolol has long been known to decrease catecholamine levels following TBI [42,43]. Labetalol has little literature available, evenly split between beneficial and unhelpful. Clonidine also reduces catecholamine levels [44], but has been reported to be useful in less than half of the cases in the literature [4]. It has been suggested that clonidine may control sympathetic crises but requires high doses (for example, 800 µg 2nd hourly) [45].
Several other pharmacological agents show a mixed picture in terms of efficacy. Dopamine agonists, predominantly bromocriptine, have a patchy literature of cases with at least partial efficacy versus where no effect was observed [4]. Benzodiazepines also present a mixed picture, with anecdotal reports favoring diazepam and midazolam [7]. Drugs that have been reported to be ineffective include methadone [7,11], metoprolol [13,29,33], oral baclofen [7,35], and phenytoin [11,28,46]. Oral dantrolene has been reported to be unhelpful in all but one patient [7,11,47].
It is readily apparent that there is insufficient available literature to provide a definitive protocol to guide management. However, in terms of minimizing the possibility of adverse drug reactions, it would appear appropriate to utilize morphine/midazolam, gabapentin and/or propanolol in the first instance. There are occasional reports of excellent efficacy with bromocriptine, suggesting it may have a secondary role. ITB is most useful in cases that are resistant to oral medication, are of greater severity or longer duration.
74.5 Current Controversies
Despite increasing research into PSH over the last decade, the current estimated incidence of the condition varies across a considerable range (between 8 and 33%) [4]. Also complicating the literature are the large number of synonyms used for the condition (around 33 in total), most of which have only been used once or twice in the literature. The more commonly used names include dysautonomia, autonomic dysfunction syndrome, paroxysmal sympathetic storms, paroxysmal autonomic instability with dystonia and so on [4]. Another difficulty with research into the condition is the 5 current sets of overlapping diagnostic criteria [5,27,34]. In addition, it has recently been suggested that the concept of “triggering” of paroxysms following minor stimuli has been proposed as a clinical sign that may help to differentiate individuals with and without the syndrome [16]. While moves to critically appraise these criteria have begun [48], there is insufficient research available to be able to establish consensus criteria at this time.
Linked in with the issue of the lack of diagnostic criteria, there has also been a lack of quantitative mechanisms to investigate the pharmacological management of dysautonomia. Dysautonomic subjects tend to show marked day to day variability in their presentation (as illustrated in Figure 74.1) and an appropriate research tool would need to accurately measure the frequency, severity and duration of paroxysms over time. Recently, a number of tools have been proposed to overcome this limitation including event related heart rate changes and heart rate variability paradigms, either over 5 minute intervals [16] or 24 hour time scales [9]. Research techniques such as these are required to raise awareness of the syndrome and to properly evaluate treatment efficacy.
74.6 Key Concepts
Research suggests that PSH is a distinct but under-recognized clinical syndrome that can result from numerous forms of acquired brain injury. While short duration sympathetic hyperactivity does not appear to be associated with worse outcome, clinical research suggests that prolonged dysautonomia places a considerable burden on both patient and health care services. There is potential for reducing this burden through timely recognition and intervention, however, the field is hampered by a lack of clinical awareness, a poor understanding of pathophysiology and anecdotal management protocols. Investigatory techniques are becoming available that will allow the development of evidence based treatment paradigms for the first time. However, due to the relatively low incidence of the condition, multicentre research using standardized nomenclature and diagnostic criteria will be required to achieve this aim. It is hoped that more effective treatment protocols will, in turn, result in improved outcomes for individuals affected by this condition.
74.7 References
1. Jennett B, Teasdale G, Galbraith S, et al. Severe head injuries in three countries. J Neurol Neurosurg Psychiatry 1977; 40: 291-8
2. Baguley IJ, Slewa-Younan S, Heriseanu RE, et al. The incidence of Dysautonomia and its relationship with autonomic arousal following traumatic brain injury. Brain Inj 2007; 21: 1175-82
3. Rabinstein AA, Benarroch EE. Treatment of paroxysmal sympathetic hyperactivity. Curr Treat Options Neurol 2008; 10: 151-7
4. Baguley IJ. Autonomic complications following central nervous system injury. Semin Neurol 2008; 28: 716-25
5. Baguley IJ, Nicholls JL, Felmingham KL, et al. Dysautonomia after traumatic brain injury: a forgotten syndrome? J Neurol Neurosurg Psychiatry 1999; 67: 39-43
6. Bricolo A, Turazzi S, Alexandre A, et al. Decerebrate rigidity in acute head injury. J Neurosurg 1977; 47: 680-98
7. Pranzatelli MR, Pavlakis SG, Gould RG, et al. Hypothalamic-Midbrain Dysregulation Syndrome: Hypertension, Hyperthermia, Hyperventilation, and Decerebration. J Child Neurol 1991; 6: 115-22
8. Dolce G, Quintieri M, Leto E, et al. Dysautonomia and Clinical Outcome in Vegetative State. J Neurotrauma 2008; 25: 1079-82
9. Baguley IJ, Nott M. Quantitating the Efficacy of Gabapentin in a Novel Case of Dysautonomia. Neurorehabil Neural Repair 2008; 22: 570-1
10. Penfield W. Diencephalic autonomic epilepsy. Arch Neurol Psychiatry 1929; 22: 358-74
11. Rossitch E, Bullard DE. The Autonomic Dysfunction Syndrome: aetiology and treatment. Br J Neurosurg 1988; 2: 471-8
12. Bhigjee AI, Ames FR, Rutherford GS. Adult aqueduct stenosis and diencephalic epilepsy. A case report. J Neurol Sci 1985; 71: 77-89
13. Do D, Sheen VL, Broomfield E. Treatment of paroxysmal sympathetic storm with labetalol. J Neurol Neurosurg Psychiatry 2000; 69: 832-3
14. Baguley IJ, Heriseanu RE, Gurka JA, et al. Gabapentin for the management of dysautonomia following severe traumatic brain injury: a cautionary tale. J Neurol Neurosurg Psychiatry 2007; 78: 539-41
15. Baguley IJ, Heriseanu RE, Cameron ID, et al. A critical review of the pathophysiology of Dysautonomia following traumatic brain injury. Neurocrit Care 2008; 8: 293-300
16. Baguley IJ, Nott MT, Slewa-Younan S, et al. Diagnosing Dysautonomia following acute traumatic brain injury: evidence for over-responsiveness to afferent stimuli. Arch Phys Med Rehabil 2009; 90: 580-6
17. Baguley IJ, Heriseanu RE, Nott MT, et al. Dysautonomia after severe traumatic brain injury: evidence of persisting overresponsiveness to afferent stimuli. Am J Phys Med Rehabil 2009; 88: 615-22
18. Penfield W, Jasper H. Somatic motor seizures. In: Penfield W (ed.). Epilepsy and the functional anatomy of the human brain. London: J & A Churchill Ltd 1954; pp. 350-88
19. Baguley IJ. The excitatory: inhibitory model (EIR model): An integrative explanation of acute autonomic overactivity syndromes. Medical Hypotheses 2007; 70: 26-35
20. Sandel ME, Abrams PL, Horn LJ. Hypertension after Brain Injury: Case Report. Arch Phys Med Rehabil 1986; 67: 469-72
21. Baguley IJ, Cameron ID, Green AM, et al. Pharmacological management of Dysautonomia following traumatic brain injury. Brain Inj 2004; 18: 409-17
22. Cervero F, Laird JM. Mechanisms of touch-evoked pain (allodynia): a new model. Pain 1996; 68: 13-23
23. Baguley IJ. Nomenclature of “paroxysmal sympathetic storms” [letter; comment]. Mayo Clinic Proceedings 1999; 74: 105
24. Clifton GL, Robertson CS, Choi SC. Assessments of nutritional requirements of head-injured patients. J Neurosurg 1986; 64: 895-901
25. Moore R, Najarian MP, Konvolinka CW. Measured Energy Expenditure in Severe Head Trauma. J Trauma 1989; 29: 1633-6
26. Mehta NM, Bechard LJ, Leavitt K, et al. Severe weight loss and hypermetabolic paroxysmal Dysautonomia following hypoxic ischemic brain injury: the role of indirect calorimetry in the Intensive Care Unit. JPEN J Parenter Enteral Nutr 2008; 32: 281-4
27. Hendricks HT, Guerts ACH, van Ginnekin BC, et al. Brain injury severity and autonomic dysregulation accurately predict heterotopic ossification in patients with traumatic brain injury. Clinical Rehabilitation 2007; 21: 545-53
28. Boeve BF, Wijdicks EF, Benarroch EE, et al. Paroxysmal sympathetic storms (“diencephalic seizures”) after severe diffuse axonal head injury. Mayo Clinic Proceedings 1998; 73: 148-52
29. Baguley IJ, Heriseanu RE, Gurka JA, et al. Gabapentin in the management of dysautonomia following severe traumatic brain injury: a case series. J Neurol Neurosurg Psychiatry 2007; 78: 539-41
30. Baguley IJ, Heriseanu RE, Felmingham KL, et al. Dysautonomia and Heart Rate Variability Following Severe Traumatic Brain Injury. Brain Inj 2006; 20: 437-44
31. Bullard DE. Diencephalic Seizures: Responsiveness to Bromocriptine and Morphine. Ann Neurol 1987; 21: 609-11
32. Krach LE, Kriel RL, Morris WF, et al. Central autonomic dysfunction after acquired brain injury in children. J Neurol Rehabil 1997; 11: 41-5
33. 33. Lemke DM. Sympathetic storming after severe traumatic brain injury. Critical Care Nurse 2007; 27: 30-7
34. Blackman JA, Patrick PD, Buck ml, et al. Paroxysmal autonomic instability with dystonia after brain injury. Arch Neurol 2004; 61: 321-8
35. Francois B, Vacher P, Roustan J, et al. Intrathecal baclofen after traumatic brain injury: early treatment using a new technique to prevent spasticity. J Trauma 2001; 50: 158-61
36. Cuny E, Richer E, Castel JP. Dysautonomia syndrome in the acute recovery phase after traumatic brain injury: relief with intrathecal Baclofen therapy. Brain Inj 2001; 15: 917-25
37. Becker R, Benes L, Sure U, et al. Intrathecal baclofen alleviates autonomic dysfunction in severe brain injury. J Clin Neurosci 2000; 7: 316-9
38. Anderson VL, Ahmed G, Duraski SA, et al. Alternative treatment in the management of combined hyperadrenergia and spasticity in the adult with a severe traumatic brain injury: A case report. Arch Phys Med Rehabil 2004; 85: e15
39. Follett KA, Burchiel K, Deer T, et al. Prevention of Intrathecal Drug Delivery Catheter-Related Complications. Neuromodulation 2003; 6: 32-41
40. Guillaume D, Van Havenbergh A, Vloeberghs M, et al. A clinical study of intrathecal baclofen using a programmable pump for intractable spasticity. Arch Phys Med Rehabil 2005; 86: 2165-71
41. Salim A, Hadjizacharia P, Brown C, et al. Significance of troponin elevation after severe traumatic brain injury. J Trauma 2008; 64: 46-52
42. Robertson CS, Clifton GL, Taylor AA, et al. Treatment of hypertension associated with head injury. J Neurosurg 1983; 59: 455-60
43. Feibel JH, Baldwin CA, Joynt RJ. Catecholamine-associated refractory hypertension following acute intracranial hemorrhage: control with propranolol. Ann Neurol 1981; 9: 340-3
44. Metz SA, Halter JB, Porte D Jr, et al. Autonomic epilepsy: clonidine blockade of paroxysmal catecholamine release and flushing. Ann Int Med 1978; 88: 189-93
45. Dunne SL. Clonidine for the treatment of paroxysmal autonomic instability with dystonia following traumatic brain injury. Paediatric and Perinatal Drug Therapy 2007; 8: 115
46. Talman WT, Florek G, Bullard DE. A hyperthermic syndrome in two subjects with acute hydrocephalus. Arch Neurol 1988; 45: 1037-40
47. Diesing TS, Wijdicks EF. Arc de Cercle and dysautonomia from anoxic injury. Movement Disorders 2006; 21: 868-9
48. Baguley IJ, Nott M. Investigating the Impact of Differing Diagnostic Criteria for Dysautonomia and ‘PAID’: Determining Caseness. Neurorehabilitation and Neural Repair 2008; 22: 570
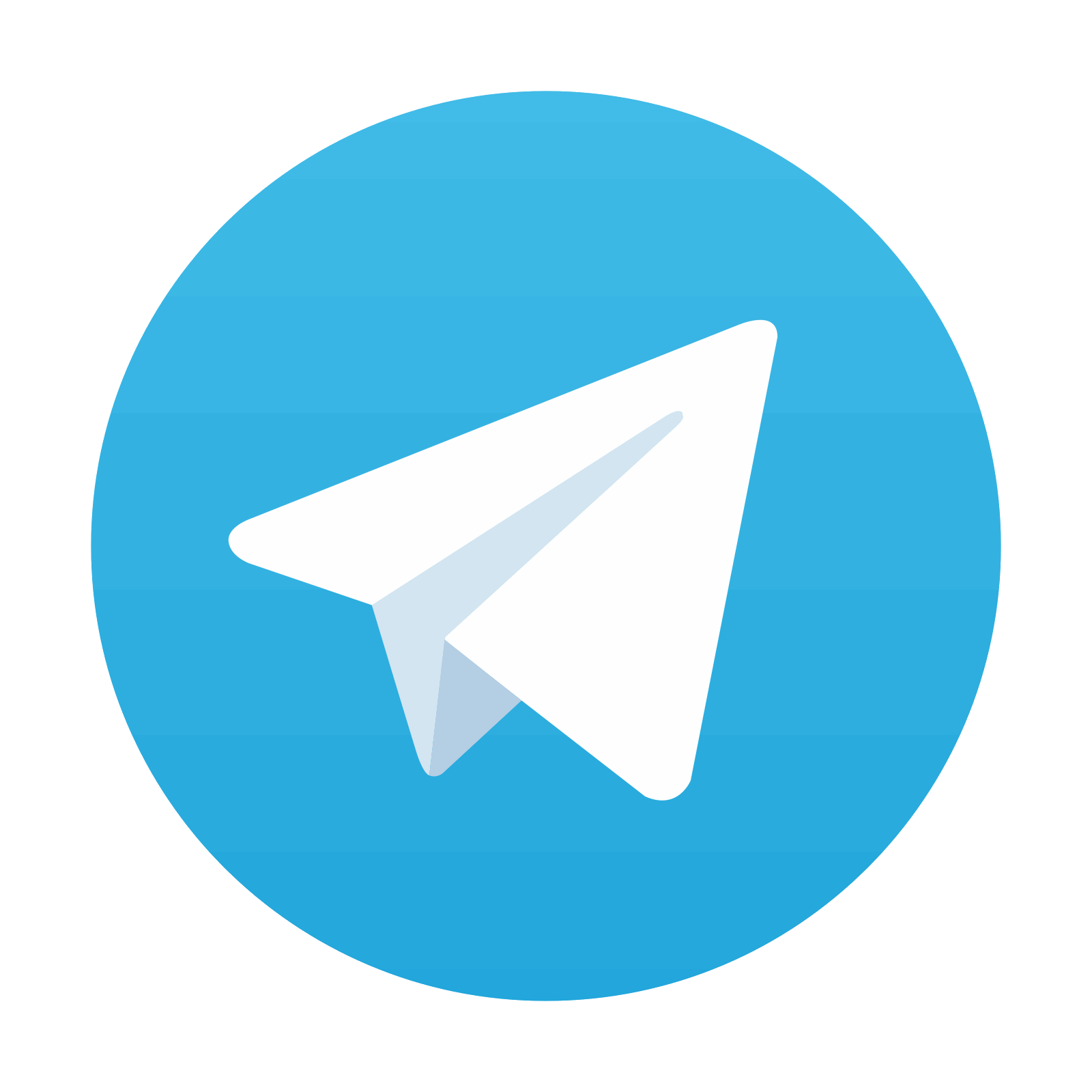
Stay updated, free articles. Join our Telegram channel

Full access? Get Clinical Tree
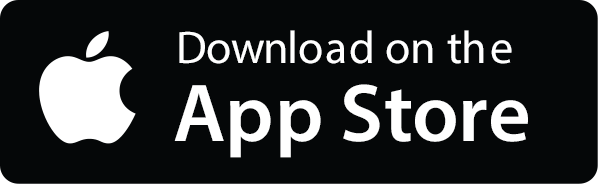
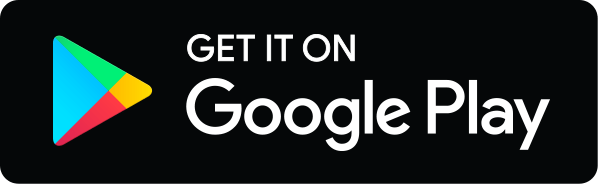