CHAPTER 316 Pathology of the Cervicothoracic Junction
Evaluation and Treatment
Anatomically, the cervicothoracic junction has varying definitions; however, it is strictly defined as the functional unit of the C7 and T1 vertebral bodies and the intervening disk space. Many authors define this region as a transition zone and include the distal cervical spine through the T3 disk space to account for the biomechanical properties and anatomic variability.1–3 We define the cervicothoracic junction as the superior end plate of the C7 vertebral body to the T3-4 disk space. This chapter reviews the unique anatomy and biomechanical principles of the cervicothoracic junction, imaging techniques, and surgical management of common pathologic entities involving this region.
History and Physical Examination
Pain, as is often seen in other spinal pathologies, is the most common complaint of patients with cervicothoracic pathology.4 Differentiating between musculoskeletal pain, osteoporotic fractures, pathologic fractures, degenerative disease, and infection can be difficult. For example, patients with a lung malignancy can have complaints of dysesthetic nerve root pain rather than neurological sequelae secondary to cord compression. Isolated axial pain is uncommon. Interestingly, isolated osteoporotic vertebral compression fractures at the cervicothoracic junction are rare because of the decreased load on the anterior column, and such fractures should be lower on the list of differential diagnoses. Therefore, the presence of a compression fracture over this region may suggest the possibility of a pathologic fracture from metastatic disease and should be evaluated as such.
Although not the initial symptom, up to 80% of patients with disorders of the cervicothoracic junction are found to have neurological impairment when first evaluated.1 This high incidence of neurological dysfunction is related to the limited amount of space in the canal as a result of the large spinal cord–to-canal ratio; the smaller caudal cervical and upper thoracic vertebral bodies, which can lead to earlier encroachment on nervous tissue by infection and neoplasm; and theoretically, the tenuous blood supply to the spinal cord over this region.5 Thus, patients commonly exhibit global destruction over the region, whereas isolated radiculopathy and individual nerve root injuries are uncommon findings.6 When patients have signs of neurological dysfunction, they are more often found to have myelopathic findings than patients with pathology in other regions of the spinal column. Patients may initially be seen with or without signs or symptoms in the upper extremities, depending on whether the disease process is affecting the C8 and T1 nerve roots. Myelopathic features encountered include long-tract signs, pathologic reflexes (Babinski’s signs), bowel or bladder dysfunction (or both), lower extremity weakness, and gait dysfunction.7
Patients may also exhibit or are found on examination to have Horner’s syndrome, defined as ptosis, miosis, anhidrosis, and enophthalmos, because of interruption of the sympathetic innervation of the ipsilateral orbit and face.8 Cervicothoracic junction pathology may disrupt either the second-order (damage to preganglionic axons as they approach the stellate ganglion) or third-order sympathetic neurons (damage to postganglionic axons within or on leaving the stellate ganglion).9 Diseases that are destructive or expansile may involve the sympathetic neurons and their associated ganglia. This is typically associated with extension of a primary lung neoplasm, infiltration of an infectious or metastatic process into the vertebral bodies, or iatrogenic injury after anterior spinal approaches.10
Anatomy
The Superior Mediastinum and Associated Vasculature
The superior mediastinum extends from the thoracic inlet to the plane between the manubriosternal joint and the T4-5 disk space (Fig. 316-1). Proximally, the thoracic inlet is bounded by the superior aspect of the manubrium, the superior border of the first rib, and the T1 vertebral body.7,11 The manubriosternal joint, also referred to as the angle of Louis, is the junction of the manubrium and body of the sternum and is the point of attachment of the second costal cartilages. An axial line drawn from this joint to the T4-5 disk space defines the inferior margin of the superior mediastinum.
The subclavian artery and accompanying vein traverse the superior aspect of the first rib and are in close proximity to the anterior scalene muscle, which can be used to localize these structures. The subclavian vein passes anterior to the anterior scalene muscle, whereas the subclavian artery passes posteriorly. At the medial border of the anterior scalene muscle, the left subclavian vein joins with the left internal jugular vein at a sharp angle to form the left brachiocephalic vein. The thoracic duct, which returns lymphatic drainage to the circulatory system, drains into this left venous angle in the majority of patients.11,12 The left brachiocephalic vein then courses inferiorly and medially, posterior to the manubrium, until it reaches the first right intercostal space and unites with the right brachiocephalic vein to create the superior vena cava.11
Neural Structures of the Superior Mediastinum
The Vagus and Recurrent Laryngeal Nerves
Two important anatomic relationships exist between the vagus nerves and associated structures. First, the vagus nerves descend in the carotid sheath and pass anterior to the major arterial structures of the superior mediastinum. The right vagus nerve travels ventral to the right subclavian artery, whereas the contralateral vagus nerve descends anterior to the aortic arch. Both vagus nerves then descend posterior to the hilar structures of the lung. However, before reaching the lung hila, both vagus nerves give rise to an RLN. The right RLN descends parallel to the carotid artery and loops beneath the right subclavian artery, typically at the level of T3.13 It then courses medially and superiorly to reenter the neck, hence the name recurrent, and travels along the tracheoesophageal groove. On the left side, the RLN also descends parallel to the carotid; however, because of the presence of the aortic arch, it loops around distally at the level of the ligamentum arteriosum before entering the tracheoesophageal groove. Both RLNs then ascend posterior to the lateral lobes of the thyroid gland and have a variable relationship to the inferior thyroidal artery and veins, potentially crossing either ventral or dorsal to the vessels. In the cervical region, a left-sided approach is believed to be associated with a lower incidence of injury because the left RLN travels distally and more frequently within the tracheoesophageal groove than the right RLN does.13,14
The Phrenic Nerves
The phrenic nerves, which innervate the diaphragm, arise from the motor component of the C3-5 nerve roots and leave the cervical spinal cord to enter the mediastinum posterior to the ventral portion of the first rib. These nerves descend anterior to the aorta on the left and the subclavian artery on the right. Their course through the mediastinum differs from that of the vagus nerves in that they pass anterior to the hila of the lungs.6,11
Viscera of the Superior Mediastinum
The Esophagus, Trachea, and Lungs
The trachea divides into the primary bronchi at the manubriosternal joint. Along with the esophagus, it courses through the mediastinum from C6 to T5.6,11 During dissection, this close association between these structures allows them to be mobilized medially as a single unit.
The lung apices and associated pleura are consistently exposed during posterolateral or ventral approaches to the distal thoracic spine (T8 to T12); however, anterior exposure of the cervicothoracic junction will also expose these structures. Stanescu and coauthors reported that in 46% of cadaveric axial sections through the T1 body, the pleural cavity was directly anterolateral to the vertebral body.3 Thus, even a distal anterior approach, without manubriotomy or sternotomy, will expose the pleura to potential injury.9
The Thoracic Duct
Although a left-sided approach to the cervical spine is reported to have a lower incidence of RLN injury, it does potentially expose the thoracic duct to injury.15,16 The thoracic duct, which returns lymphatic drainage to the systemic circulation, typically empties into the left jugulosubclavian junction located between C7 and T1.15 However, this course is variable and the termination point may extend cranially to C6. The jugulosubclavian junction lies within a triangle bounded by the medially placed esophagus and longus colli muscles, the laterally lying anterior scalene, and the inferiorly based first rib.17 When dissecting through this region, identification and manipulation of the thoracic duct are necessary, and Resnick reported that ligation and division of this structure is well-tolerated.16
The Vertebral Arteries
The vertebral artery typically enters the cervical foramen transversarium at the C6 level, but in up to 5% of patients the vertebral artery enters at the C7 foramen.11,18 The vertebral artery, from its origin at the subclavian to its entrance into the foramen magnum, follows a variable path. The artery may be located anterior to the lateral mass (0% to 14%) or lateral to the pedicle (14% to 100%), or rarely, it may be shifted so far anterior that it is found lateral to the cervical vertebral bodies.3
The Cervicothoracic Spinal Column
Surgical treatment of the cervicothoracic junction can be a challenging endeavor because of obstacles encountered during the anterior and posterior approaches. Posterior decompression and stabilization are technically difficult as a result of the transitional and variable anatomic dimensions of each vertebral segment. Anterior access to the upper thoracic vertebrae is limited by the mediastinal structures and, additionally, by the thoracic kyphosis, which angles the disk spaces away from the surgeon. Distally in the cervical spine, the lateral masses decrease in anteroposterior thickness, thus limiting the area for internal fixation. Pedicle width increases from C5 to C7-T1 but then decreases from T1 to T5.3
To assess the variability in the dimensions of the bony structures in the cervicothoracic junction, Stanescu’s group analyzed 324 cross-sectional spinal segments from nine cadaveric specimens.3 Anteroposterior lateral mass thickness measured in the sagittal plane was found to be greatest at C5 and then successively decreased, with the greatest decrease occurring between C6 and C7.3 Pedicle width measured at the vertebral base progressively increased from C5 to T1 and then decreased from T1 to T5. The pedicle base measurements had a more significant change in width than did the laminar measurements.3,7,14 Pedicle height increases from C5 to T5, whereas medial angulation decreases from C7 to T2 because of the progressive decrease in width of the thoracic lamina.3,7,14
The diameter of the vertebral bodies and spinal canal is also an important dimension to consider when treating disorders of the cervicothoracic junction. Vertebral body sagittal diameter from C5 to T3 remains relatively constant, with a minimal increase in diameter of the superior end plate from 18.9 to 21.4 mm. In the coronal plane, vertebral body height increases from 27.9 mm at C5 to 29 mm at T1 and then decreases to 25.4 mm at T3.3 The spinal canal is relatively narrow through this region and assumes an oval shape averaging 24 by 15 mm, with the widest dimension in the transverse plane. The spinal cord enlarges over C3 to T2 because of the presence of the brachial plexus.6 The combination of diminishing bony dimensions and enlarging neural structures results in two thirds of the spinal canal being occupied by the spinal cord, with little room left for space-occupying pathology. Additionally, the large nerve roots associated with the cervical enlargement occupy two thirds of the intervertebral foramina.
Blood supply to the spinal cord undergoes a transition through this region as the anterior and posterior spinal arteries become less prominent. The caudal cervical spinal cord derives its blood supply from radicular branches of the vertebral, thyrocervical, and costoclavicular arteries, whereas the upper thoracic cord receives branches from the supreme intercostal arteries.7,11
Biomechanical Considerations
The transition from the cervical to the thoracic spine must be accounted for when treating pathology in this region. The mobile, lordotic cervical spine transitions into the relatively rigid, thoracic kyphosis. Through a wide range of flexion, extension, and rotation of the cervical spine, the head can assume a multitude of positions and maintains overall sagittal balance. The cervical spine has a range of 60 to 75 degrees in flexion-extension.19 Axial rotation distal to C6 is limited to a range of just 4 to 8 degrees.19,20 In addition, lateral bending has a range of 6 to 8 degrees from C5 to T6.19,21 Little bony stability is derived from the osseous cervical spine, and the majority of cervical stabilization is derived from the ligamentous structures. The thoracic spine, through its association with the stabilizing architecture of the chest, including the scapulae, clavicles, ribs, and sternum, is relatively rigid and immobile.22,23 The stark contrast in range of motion between these two adjacent regions subjects the cervicothoracic junction to translational forces, excessive flexion, and a significant transfer of axial loads (Fig. 316-2).5
Trauma, degeneration, infection, and neoplasia can result in instability of the cervicothoracic segment because of compromise of these biomechanical properties. Destabilization of the cervicothoracic junction can result in a progressive kyphotic deformity, translation of the vertebral elements, narrowing of the spinal canal, and compression of the spinal cord and neural elements.24 Failure to account for these unique biomechanical principles when operating in this region may result in construct failure, a worsening deformity, and neurological compromise.
Diagnostic Studies
Plain Radiography
Evaluation of the cervicothoracic junction with plain roentgenographic imaging is frequently limited, and adequate visualization may not be achieved in up to 26% of patients.25 The swimmer’s view displaces the shoulder from its position overlying the spine, yet limiting factors such as coexisting neurological impairment, injuries to the upper extremity or chest, and persistent obscuration by the shoulder commonly render this view inadequate.26 In a series of 100 swimmer’s view radiographs obtained over a 2-year period in trauma patients, Rethnam and colleagues evaluated the images for adequate visualization of the C7 body and the C7-T1 junction.26 Only 55% of the images were adequate, with none of the inadequate radiographs satisfactorily visualizing the C7 body and the C7-T1 junction.26 In 2000, Jelly and coauthors published a prospective study that examined a series of 73 trauma patients with spiral CT, 12 of whom were found to have fractures at the cervicothoracic junction.27 These fractures were demonstrated with conventional radiography in only 5 of the 12 patients (42%).27
Computed Tomography
The use of high-resolution multidetector CT has increased the speed, sensitivity, and accuracy of the diagnosis of fractures of the cervicothoracic junction.28 In Tan and associates’ series of 360 blunt trauma patients in whom the C7-T1 level was not adequately visualized on plain radiographs, CT led to detection of 11 injuries to the cervicothoracic junction.29 Avoidance of the potential sequelae of undiagnosed cervicothoracic junction injuries proved cost-effective, with $9192 saved for each fracture identified, $16,852 for each potentially or definitely unstable fracture identified, and $50,557 for each definitely unstable fracture identified.29
CT of the cervicothoracic junction has proved to be useful in surgical planning because it details the bony and soft tissue relationships in a region of anatomic variability and visualizes compromise of neural structures.1 CT reconstructions provide a three-dimensional image of the osseous spine and allow the surgeon to make measurements for designing constructs preoperatively.
Magnetic Resonance Imaging and Myelography
MRI offers a noninvasive detailed assessment of the soft tissues, neural anatomy, and potential pathology over the cervicothoracic region and is thus used more often than invasive myelography. In traumatic injury, MRI evaluates for the presence of hematomas, ligamentous injury, and disk herniations while also identifying nerve root and spinal cord compression or signal abnormality.1 The addition of a contrast agent (gadolinium) provides detailed enhancement of tumors and abscesses while also improving the visualization of neural tissue.
Preoperative sagittal MRI scout images can be used to evaluate the relationship between the sternal notch and level of pathology to determine whether access without a thoracotomy is possible.30 In patients who have contraindications to MRI (metal prostheses, spinal cord stimulators, implantable defibrillators) or in whom the MRI table will not support their body habitus, myelography with postmyelographic CT is an alternative. Cord compression is most effectively demonstrated in the sagittal plane, whereas axial imaging allows visualization of dye-filling defects in the nerve root sleeve but does not effectively visualize intraspinal pathology.
Surgical Approaches
Anterior Approaches
The Low Anterior Cervical Approach
Pathology ventral to the cervical spinal cord has traditionally been addressed through the anteromedial or Smith-Robinson approach. Although this approach effectively exposes pathology in the upper cervical spine, it is limited in the caudal cervical and upper thoracic spine, particularly distal to the T2 vertebra. Access to the vertebral elements is restricted by body habitus and the regional anatomy of the manubrium and sternum. In a series of 7 patients, Sharan and coworkers concluded that the upper thoracic vertebrae can be exposed through a suprasternal approach without sternotomy or thoracotomy.30 Midsagittal cervicothoracic MRI was used to identify the thoracic vertebrae above the sternum, thus determining whether the low suprasternal approach was feasible. In a review of MRI of 103 cervicothoracic junctions, Sharan and colleagues reported that the T1-2 disk space could be accessed through a suprasternal route. A direct suprasternal approach to the T2-3 disk space was possible in only 15 of 103 (15%) patients.30 However, if the goal of surgery was corpectomy, exposure down to the T3 vertebral body could be achieved. Corpectomy enlarges the area of exposure because of greater visualization of the thecal sac along a more rostral-caudal trajectory and by eliminating the restricted angulation of the disk space. Pathology below the level of the sternal notch on preoperative imaging was addressed with a lateral extracavitary or transpedicular approach, thereby avoiding sternotomy or manubriectomy (Fig. 316-3).30
A low cervical approach is performed with the patient supine and the neck and head in maximal extension. A longitudinal incision parallel to the sternocleidomastoid muscle is brought down to the sternal notch. The platysma muscle is divided and the sternohyoid and sternothyroid muscles are then mobilized medially. The omohyoid, which often lies in the field when the lower cervical spine is exposed, is divided if necessary. Blunt dissection is carried down to the vertebral bodies, with care taken to maintain the tracheoesophageal structures medially and the carotid sheath laterally.7,15,25 If the plane of dissection is not maintained medial to the carotid sheath or if the carotid is subjected to excessive retraction, the descending limb of the RLN will be placed in jeopardy. Additionally, if the trachea is retracted too vigorously, the transverse or ascending segments of the RLN (or both) may suffer a stretch injury.15
Right versus Left-Sided Approach
The superiority of the left-sided versus the right-sided approach to the cervicothoracic junction is debated in the literature. In 1976, Fielding and Stillwell advocated a right-sided approach to avoid the thoracic duct and gain better control of the innominate vessels.31 In their series of 14 patients, Boockvar and coworkers also favored the right-sided approach because of the more variable course of the left RLN and left-sided thoracic duct.24
Resnick, in a series of 21 patients undergoing anterior cervicothoracic junction corpectomy and plate fixation, preferred a left-sided approach.16 He noted that the right RLN, although more consistent in its path, crosses the operative field obliquely and was more susceptible to injury.16 Additionally, the only case of permanent vocal cord paralysis in his series resulted from a right-sided approach. He reported that with a left-sided approach, identification and retraction of the thoracic duct were achieved routinely and ligation was well tolerated. Ultimately, surgeon preference and familiarity are the determining factors when choosing a right- versus left-sided approach.
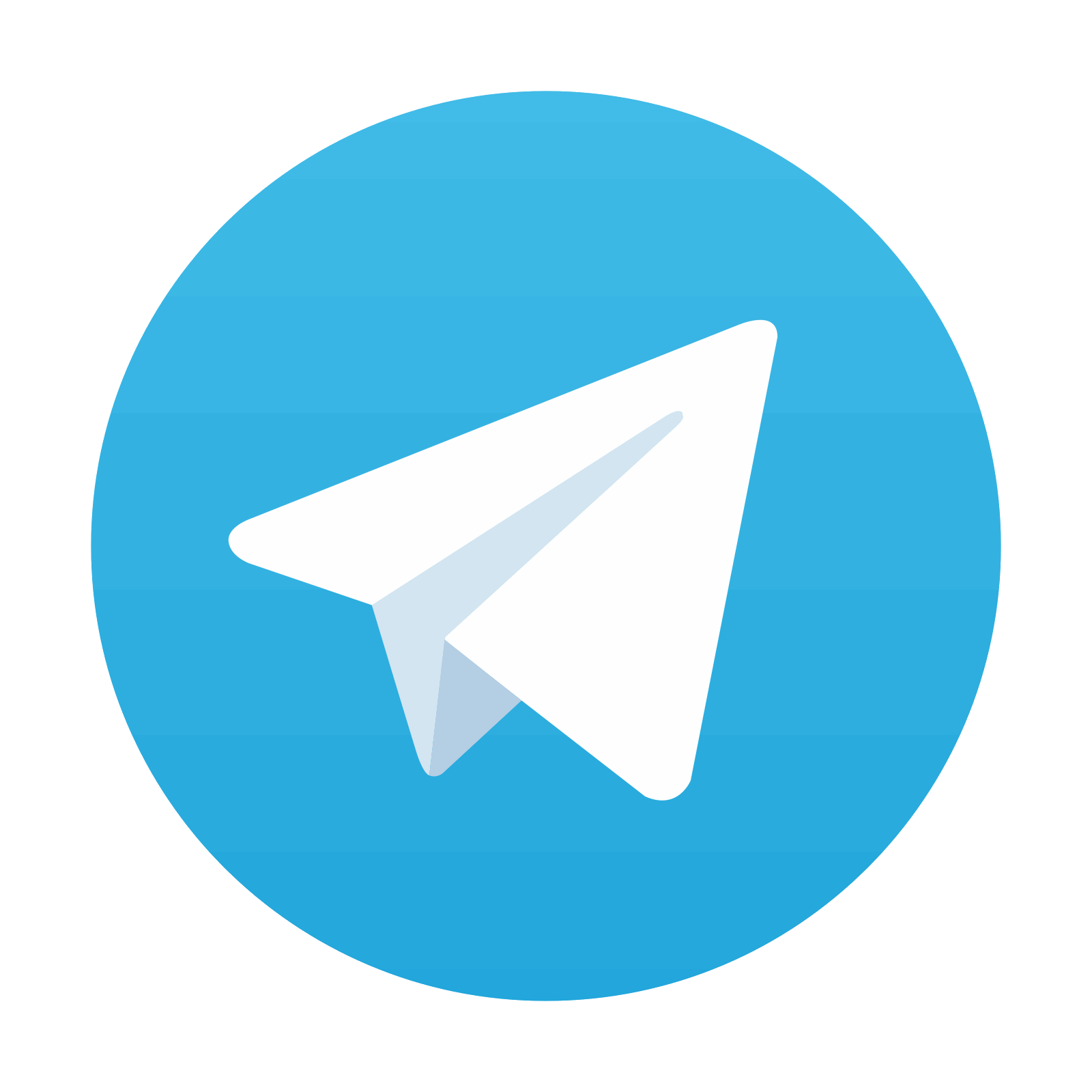
Stay updated, free articles. Join our Telegram channel

Full access? Get Clinical Tree
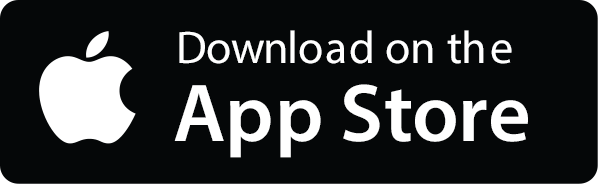
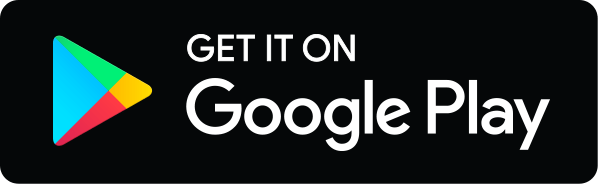