8 Perceptuo-motor learning
At the end of this chapter, you should be able to:
• describe the concept of perceptuo-motor learning and differentiate this from motor performance
• apply an information-processing (or motor programming) model to the process of perceptuo-motor learning
• distinguish between three main stages of motor learning and compare and contrast the needs of the patient (or learner) in each of these stages
• explain how perceptuo-motor learning may be facilitated through motivation, practice and feedback
• discuss how this information may be applied to rehabilitation practice.
Introduction
‘Practice makes perfect’ is a well-known adage used in a range of scenarios to encourage learning, from learning to play a musical instrument or a sport for the first time, to re-learning functional skills in the context of rehabilitation. Consider the case study below (Case study 8.1).
Following on from the chapter on perceptuo-motor control, this chapter will begin by introducing a number of key concepts and present an overview of the main stages of the perceptuo-motor learning process. We will build on the information processing approach to perceptuo-motor control, introduced in Chapter 7, for reasons explained there. A more detailed discussion will follow about key factors that impact on the process of skill acquisition, and what the implications for health care professionals involved in rehabilitation may be. But first, we need to go back in time and discuss how ‘learning’ has been studied in the past in order to avoid some pitfalls. For a more comprehensive overview of the history of motor learning research, the reader is referred to Summers (in Williams and Hodges 2004).
The learning curve: performance versus learning
How do we know whether patients have learned anything from what they practised? A ‘learning curve’ is a graphical representation of performance over time. Typically, performance on an outcome measure (Y-axis) is plotted at specific points in time (X-axis). Figure 8.1 presents a learning curve from a classic study by Stelmach in 1969, where performance was measured as the number of rungs climbed on the Bachman ladder (a novel ladder with unequally distributed rungs on either side of a vertical beam in the middle of the ladder). The purpose of the experiment was to compare the effectiveness of two different training regimes; ‘distributed practice’ (i.e. practice interspersed with rest, with more rest than practice) and ‘massed’ (i.e. more practice than rest).
Looking at figure 8.1, which schedule was more effective? Clearly, the distributed practice schedule resulted in a higher number of rungs climbed, compared to the massed practice schedule. Thus, motor performance appeared to be better following distributed practice.
In Stelmach’s study, a retention test was used, following a short delay (4 minutes only). It is interesting to note that at the retention test, there is now hardly any difference between the two practice schedules (Fig. 8.2). What happened in that short period of time? Imagine yourself undertaking the Bachman ladder task under the massed practice schedule: stepping up and down as fast as you can, again and again. It is likely that fatigue would set in and impair your performance: your leg extensors would fatigue quickly with the fast concentric and eccentric contractions. However, after a short break the increased muscle tension reduces, circulation improves, your attention may also become more focused, and altogether you are able to deliver a much better performance.
‘the behavioural act of performing a skill at a specific time and in a specific situation.’
(Magill (2007, p. 440)
In contrast, motor learning can be defined as:
Three points are worth noting in the motor learning definition:
1. Practice or experience is required, i.e. an initial successful attempt would not be defined as a learned action stored in memory
2. Changes in performance are relatively permanent
3. Motor learning is concerned with skilled action, i.e. goal-oriented behaviour, but not reflex-like behaviour, which is largely automatic.
What is the moral of the study by Stelmach? The important point to take away is that one should not jump to conclusions about the effectiveness of a specific training schedule, if performance has only been tested immediately at the end of the training. The clinical equivalent would be to use an outcome measure at the start and immediately after practising a specific activity, and base conclusions about the effectiveness of the intervention on just this outcome. In this case, the clinician will only have tested the patient’s performance. Patients may appear to perform well following the end of an intervention, but effects may not be sustained without further practice. The need to include a follow-up after the end of an intervention is illustrated in Research box 8.1 below on cueing in patients with Parkinson’s disease (PD).
Research box 8.1 Short- and longer-term effects of cueing on the coordination of gait in Parkinson’s disease
The Rehabilitation in Parkinson’s Disease: Strategies for Cueing (ResCUE) trial, the first large scale randomised trial on cueing in Parkinson’s disease (PD), was designed to examine the effects of a home-based cueing programme on gait parameters, gait-related activity and health-related quality of life in people with PD (Nieuwboer et al. 2007). In this randomised cross-over trial, a total of 153 people with PD participated in a cueing intervention, which comprised a 3-week programme with nine treatment sessions of 30 minutes each. In the first week, patients tried auditory, visual as well as somatosensory cueing modalities, i.e. beeps administered via an earpiece, flashes from an LED mounted on a pair of glasses, and pulsed vibrations applied via a wristband-mounted device. Having tried each of these modalities, patients then continued training with the one they preferred. Most patients (67%) selected the auditory, while the remainder chose the somatosensory modality. While being instructed to time their heel strike according to the rhythm provided and to continue stepping through turning and other transitions, patients undertook a variety of tasks. These included starting and stopping walking, sideways and backward stepping, walking while doing another task (i.e. dual tasking), and walking outside over different surfaces. More information about the specific cueing intervention and the underlying evidence is detailed on the ReSCUE project CD-ROM (2002/3,http://www.rescueproject.org/). Depending on group allocation, this intervention was either preceded or followed by a 3-week period without any training, after which a 6-week follow-up period ensued.
We mentioned earlier that some factors may mask learning. For example, in neurological rehabilitation, pain and fatigue are common (Chapter 14). Physical practice may exacerbate pain and fatigue, which in turn may have a detrimental effect on concentration. Patients may even get bored with an activity! All these factors may affect performance. So how may a therapist obtain a more accurate picture of what a patient has actually learned? The evidence from experiments such as that by Stelmach (1969) shows that it is essential to:
1. undertake a retention test sometime after the end of the intervention. This will help reduce any practice-related effects on pain, attention and fatigue and show how much has actually been retained in memory. Assessing performance immediately after an intervention will merely show performance effects.
2. utilise a transfer test, i.e. a slight variation from the task that has been practised. This will reveal to what extent the patient is able to adapt what has been learned. This means that the outcome measure must be related but not identical to the tasks that were practised in the intervention.
Perceptuo-motor learning: what do we learn?
What is actually stored in long-term memory during the skill acquisition process? Based on Schmidt’s motor control model (Schmidt and Wrisberg 2008, see Chapter 7), the following elements of information are stored:
1. The initial conditions. E.g. if you were to reach for an object, this would involve information about the object, its location in relation to you and any other environmental conditions (e.g. predictability) that need to be incorporated in programming the action.
2. The parameters assigned to the generalised motor programme (GMP):
How do we learn? Stages of learning
Learning a new skill requires practice, and resultant changes in performance emerge over time. An understanding of this process, which can be described in stages, is important to therapists, as patients require different forms of guidance as they progress. The key stages, which were originally proposed by Fitts and Posner (1967), and later updated by Schmidt and Wrisberg (2008), are described below.
Verbal-cognitive stage
Given the limitations of short-term memory, which are often even further compromised in people with a condition affecting the CNS (Chapter 9), it is essential that therapists select key information, e.g. where to look during a balance task (rather than prescribing the optimal position of individual joints). It is important for therapists to consider the patient’s capacity to process information. For example, physiotherapy gyms or occupational therapy kitchens are often lively environments, where music may be used to create an enjoyable atmosphere. While not denying the importance of this, therapists need to consider whether this environment actually enables the patient to focus their attention on the task in hand. As Chapter 9 will explain, information that is not attended to will be poorly remembered.
Autonomous stage
In summary, the process of skill acquisition progresses through stages. Different authors have proposed more or fewer stages (e.g. see Gentile 2000), and may have used slightly different terminology. However, it is important to bear in mind that the boundaries between the stages are rather blurred. Therefore, it is sufficient to have a broad understanding of these main stages of motor learning – and the key role of the therapist in each stage.
Facilitating skill acquisition
• Practice: amount, type and scheduling of practice,
• Feedback: type and content of feedback, attentional focus, feedback scheduling.
We will now discuss each of these points in more depth.
Motivation
A technique to enhance motivation and engagement is goal setting, which is widely recognised in rehabilitation settings as a useful tool, even though there is a lack of robust evidence on its effectiveness in clinical populations (Levack et al. 2006) as well as debate on how best to conduct the process (Playford et al. 2009). Despite these uncertainties, goal setting is recommended in a number of UK clinical guidelines (RCP 2008, SIGN 2010) and there is broad consensus that the characteristics of successful goals should follow the SMARTER acronym, i.e. they should be:
Specific: goals need to be specific in order to focus one’s effort. Goals such as ‘I want to be normal again’ lack precision about the aim, and about how progress can be ascertained. A more specific goal could be: ‘I want to be able to ascend and descend one flight of stairs without assistance’.
Measurable: as indicated above, it is necessary to be able to assess to what extent a goal has been achieved. For example, ‘I want to be able to ascend one flight of stairs in 2 minutes without assistance’. Outcome measures comprising timed tests (e.g. the Timed Up and Go test) may be useful to provide detailed information on progress in function and activity.
Agreed: it is important that a goal is agreed between the patient and the therapist, since ownership of the goal is key to intrinsic motivation. Other authors recommend that the ‘A’ stands for ‘achievable, while others argue this should stand for ‘ambitious’.
Relevant: as indicated above, goals need to be seen to be relevant by the patient. However, it is not always possible to formulate goals that meet this criterion. E.g. for a patient with traumatic brain injury, who has to have a plaster applied to his leg to prevent contracture of the ankle plantar flexors, the goal to increase range of movement in the ankle may not seem to be immediately relevant. However, having sufficient ankle movement to achieve 90 degrees of dorsiflexion is an essential requirement for weight bearing, which in turn is necessary to reach the longer term goal of being able to walk. Therapists often need to translate short-term, impairment-based goals into goals that are perceived to be relevant in the eyes of the patient.
Time-based: goals need a timeline, otherwise interventions could be endless. Setting deadlines is one of the most difficult aspects of goal setting in clinical practice however, due to the problems with predicting outcomes. For example, ‘In 1 week from now I want to be able to ascend one flight of stairs in 2 minutes without assistance’.
E: engaging: health care professionals may forget that therapeutic activities – however beneficial for the patient – can sometimes be tedious. Devising activities that are engaging and enjoyable is key to stimulating intrinsic motivation – the stepping stone to successful self-management after discharge. The rapidly growing field of ‘games for health’, using technology such as the Nintendo Wii and Microsoft Kinect, is an interesting development in this respect.
R: reviewed. In order to keep on track, or identify what holds patients back, goals need to be regularly reviewed.
Practice: amount, types and schedules of practice
Amount of practice
To master a skill, one needs to physically practise it, but how many times? It is clear that there is a lack of clarity on this issue. Ideally, several thousands of repetitions may be required to build and refine the required motor program and for this to be mastered at an automatic level, but this depends on the individual, their baseline performance, and the nature of the skill. For example, a group of people with stroke improved upper limb function significantly by practising an average of 322 repetitions per session (3 sessions a week for 6 weeks). (Birkenmeier et al. 2010). The consensus in the skill acquisition literature is, ideally, that ‘overlearning’ is required, i.e.continuing to practice beyond proficiency. Overlearning involves using the neural network required for the execution of a task over and over again, increasing efficiency and processing speed.
In reality, do patients get an opportunity to overlearn in the clinical setting? A study by de Wit et al. (2005) showed that, in the UK, stroke patients on stroke units (the accepted gold standard for hospital care after stroke) spent on average just 10% of their waking hours in therapy. Lang et al. (2009) showed that the average number of repetitions of task-specific training for people with stroke in a number of rehabilitation units in the USA was only 32 per therapy session. It is essential that therapists make the most of the very limited time available to increase opportunities for patients to practise the skills they need to be ready for discharge. One way to do so is to extend what we mean by ‘practice’.
Mental practice and observational learning
Observational learning can be defined as:
Since the pioneering work by Bandura (1986), we have known that observation can be a powerful tool in learning. Social situations offer opportunities for role-modelling; a principle that is widely used in education in general and the field of skill acquisition in particular. However, it was not until relatively recently that a neural explanation for the phenomenon of observational learning emerged. Pioneers in observational learning, Di Pellegrino and his group, made their discovery in 1992 purely by serendipity. They observed monkeys interacting with objects, using single cell recordings of neurones in the monkeys’ cerebral cortex. To cut a long and fascinating story short, they discovered that the same network of neurones was activated when the monkey was looking at an experimenter interacting with an object, as when the monkey itself was interacting with the object (Rizzolatti and Fadiga 2005). This discovery led to the ‘direct matching hypothesis’, which postulates that by observing an actor interacting with an object, the visual representation of that activity is mapped onto the motor representation of the same activity (quite literally, a case of ‘monkey see, monkey do’). In other words, the visual map of the activity is reflected onto the motor map of the same activity. The neural network involved in this process of ‘reflection’ was aptly named the ‘mirror neurone system’. Much more work has been done since on the roles of the mirror neurone system including on humans, where a similar system has been confirmed. This discovery is so exciting, as it not only confirms earlier findings that observational learning can be effective, but it provides us with a much deeper understanding of how this process is thought to work.
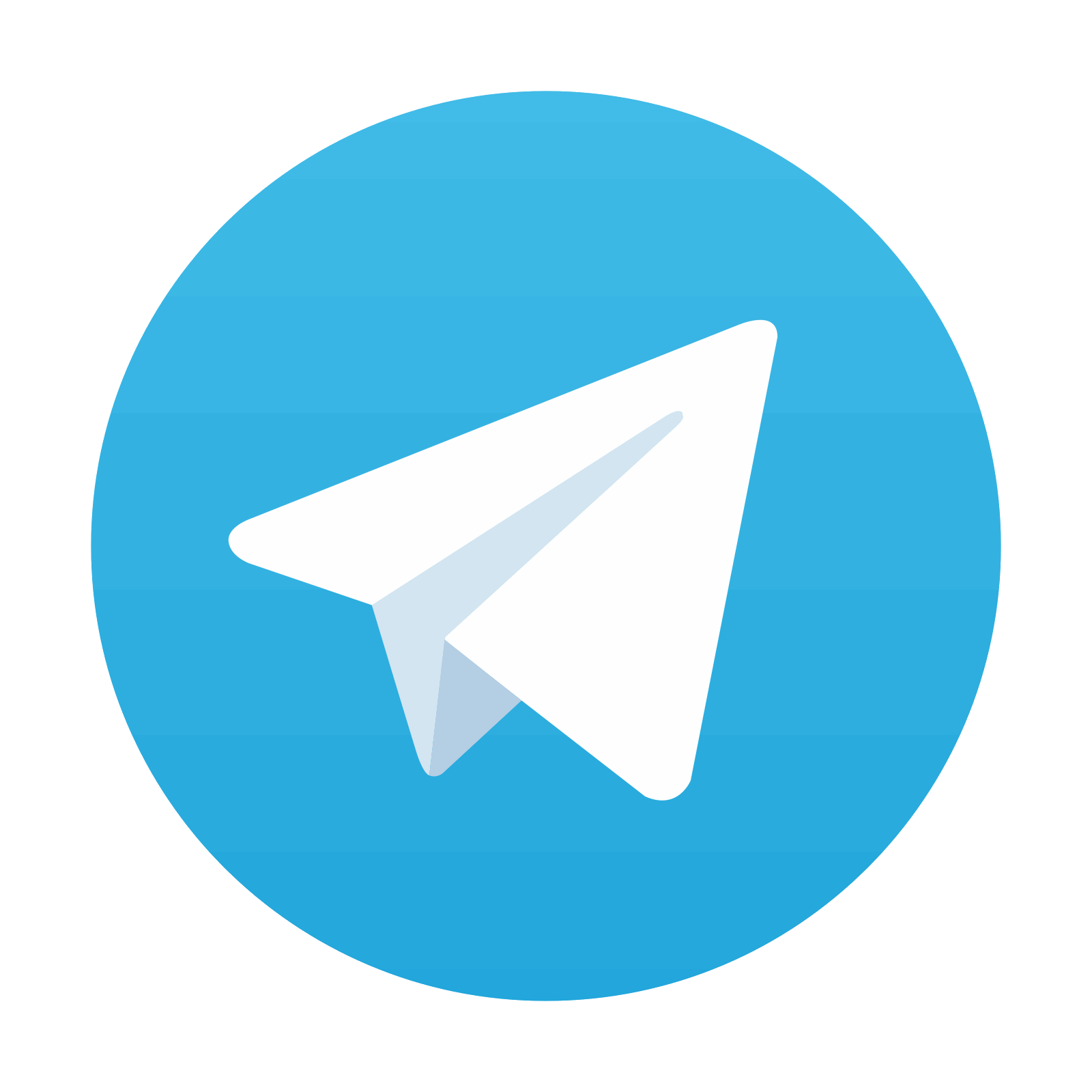
Stay updated, free articles. Join our Telegram channel

Full access? Get Clinical Tree
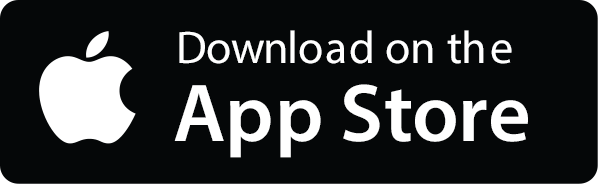
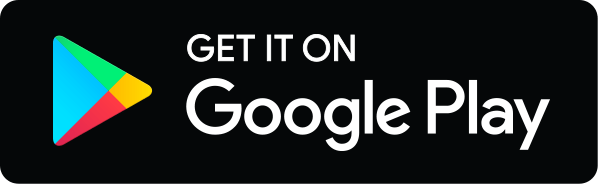