Chapter 195 Peripheral Nerve Injury
Classification
Much of our knowledge about peripheral nerve injuries and their effects came from World War I, where the number of traumatic nerve divisions was numerous. This afforded an unprecedented opportunity to observe and follow a wide spectrum of injuries and their effects if untreated over time.1 In several cases there was loss of function but maintenance of anatomic continuity, and complete peripheral degeneration ensued. In others there was loss of function but no degeneration, and rapid spontaneous recovery occurred. These observations led Seddon1,2 to develop a classification system of nerve injuries that dealt with peripheral nerve lesions causing loss of function. It could be used as a clinical guide for diagnosis and treatment. He classified nerve injury into three pathologic types based upon the three components of the normal anatomic structure of the nerve. This includes the axon, myelin sheath, and supporting connective tissue structures (endoneurium, perineurium, epineurium).
Seddon’s Classification System1,2
Neurapraxia
Neurapraxia is usually mild and reversible. Motor deficits are greater than sensory deficits. There is selective segmental demyelination, with a focal nerve conduction block across the lesion. The axon and its surrounding connective tissue supporting structures remain intact. No peripheral wallerian degeneration occurs. Complete spontaneous recovery usually occurs within weeks to months of injury. The etiology is least understood but has mostly been attributed to direct mechanical compression or transient ischemia.3,4 Experiments by Bentley and Schlapp5–7 showed that conduction block developed slower and was prolonged after release of direct pressure when compared to the faster onset and rapid recovery after relief of ischemia. Initial electromyography (EMG) shows no motor action potentials.
Axonotmesis
Axonotmesis is more severe than neurapraxia, with complete internal disruption of axons, loss of axon continuity, and demyelination. However, the epineurium, perineurium, and endoneurium remain intact. Complete distal wallerian degeneration occurs. Spontaneous recovery occurs at a regeneration rate of 1 mm per day (1 inch per month). It is usually complete as long as the regenerating fibers grow into their original endoneurial tubes, ensuring the original fiber pattern.3 Full functional recovery is expected but can take weeks, months, or even years. This injury can be caused by direct blunt injury, fractures, dislocations, contusions, or stretch or crush injuries and can result in motor, sensory, or autonomic paralysis in the autonomous distribution of the nerve. In the early phase of injury it is difficult to distinguish axonotmesis from neurotmesis on a clinical basis because the deficits are similar. In both lesions, initial electrical studies show no conduction distal to injury. After 3 weeks, EMG can show fibrillations and denervation potentials distal to the injury site.8–10 If the lesion was a pure axonometric lesion, recovery will be spontaneous and complete.
Neurotmesis
Neurotmetic injury can occur with or without loss of continuity. Complete severance of the nerve trunk results in loss of continuity of the axon, myelin, and surrounding connective tissue structures. On the other hand, lesions with intact epineurium remain in continuity but have intraneural fibrosis that limits or prevents spontaneous regeneration. Nevertheless, the pathologic and clinical manifestations are the same for both types of lesions. Wallerian degeneration occurs but spontaneous regeneration is less likely, especially if there is significant intervening scar tissue. If regeneration does occur it is not to a degree for useful functional recovery. Motor, sensory, and autonomic function are completely lost in the autonomous distribution of the affected nerve. Proximal sprouting axons that do not reach their target tissues can form neuromas. These injuries require surgical removal of scar tissue and repair with nerve grafts if large gaps are left. EMG can show fibrillations and denervation potentials distal to the injury site. These injuries are usually secondary to severe contusions, stretch, lacerations, or anesthetic toxicity, with complete transection being the most severe form.
Sunderland’s Classification System2
Sunderland described five pathologic degrees (grades 1 to 5) of injury based on the effect of the injury on the normal structural anatomy of the nerve trunk. He also addressed axonometric injuries with incomplete recovery and restoration of function that were not included in Seddon’s classification system. In these injuries there is disruption of the myelin sheaths and endoneurial tubes, allowing regenerating axons to escape from their original endoneurial tubes and enter distal foreign tubes. Therefore, continuity is not reestablished with the appropriate end organ originally innervated by it. The result is incomplete recovery with possible formation of neuromas. Sunderland described these lesions based upon disruption of the supportive connective tissue components of the nerve: the endoneurium, perineurium, and epineurium.
Grade 1
Grade 1 is similar to Seddon’s neurapraxia. Symptoms range from mild to severe, with paresis or paralysis and minor or complete sensory loss. Clinical observations have shown motor and proprioceptive fibers to be less resistant to injury than sympathetic fibers. Paresthesias can persist for several days. Proprioceptive losses usually occur first, followed by touch, temperature, and pain. Cutaneous sensory changes are usually transient. There is a reversible local conduction block at the lesion site. Duration is variable and depends on severity of injury. The myelin sheath is damaged and the histologic changes remain even after conduction is restored. Axon continuity between nerve and end organ is preserved. Complete spontaneous recovery occurs in weeks to 4 months.
Grade 2
Grade 2 is similar to Seddon’s axonotmesis, with loss of axon continuity at the injured site. There is no disruption of the axon sheaths, endoneurium, or perineurium, and other supporting structures remain intact. Loss of sensory, motor, and sympathetic function occur distal to the lesion in the distribution of the nerve. Wallerian degeneration occurs, and sometimes proximal retrograde degeneration occurs. There is breakdown of axon and myelin sheath at the injured site. During regeneration, the intact proximal portion of the axon grows distally at approximately 1 mm per day (1 inch per month) within its original endoneurial tube until it reaches its original end organ. The nerve fiber pattern remains the same, ensuring complete restoration of function. The Hoffman-Tinel test can be used to monitor the progress of regeneration. The timing and degree of recovery depends on the extent of retrograde axonal loss and the time to regenerate and reinnervate target muscles or sensory end organs and also depends on the distance from injury to target muscles. Reinnervation and onset of recovery in individual muscles proceeds from proximal to distal. The peripheral nerves with simple branching patterns and pure sensory or motor nerves have a higher chance of successful reinnervation than mixed nerves.
Grade 3
Grade 3 injuries are characterized by loss of endoneurial tube continuity in addition to axon disruption, demyelination, and wallerian degeneration. The perineurium and epineurium remain intact. The internal structure of the fasciculus is disorganized but the bundles remain in continuity. Intrafascicular fibrosis occurs from hemorrhage, edema, vascular stasis, and ischemia and can cause intrafascicular fibrosis. This delays and limits spontaneous regeneration. Fewer axons are available for regeneration because of retrograde degeneration. The remaining axons sprout and descend within the fascicle but outside of their original endoneurial tubes.
Intrafascicular fibrosis causes some axons to enter functionally unrelated tubes, resulting in a new pattern of innervation in the distal end organ. Sunderland refers to this as cross-shunting of axons.11 The consequences are different for an individual fascicle containing fibers from several different branches as opposed to the similar branches. If all of the fascicles are involved there is complete loss of motor, sensory, and sympathetic function in the autonomous distribution of the nerve. For partial lesions the fiber composition of the injured fascicle(s) determines the type and extent of defect. There can be mild to severe motor and sensory losses.
Grade 4
In grade 4 injury, the entire fascicle and its surrounding perineurium is disrupted and is very disorganized. All bundles are breached. The epineurium is intact and continuity of the nerve trunk is maintained, but one cannot delineate the fasciculi from the epineurium. Severe fibrosis limits axon regeneration. Neuromas form. The incidence of retrograde degeneration and axonal loss is higher. Wallerian degeneration occurs in the distal stump. During regeneration, axons enter the interfascicular spaces. Some terminate blindly. Very few reach their original distal endoneurial tubes, resulting in spontaneous recovery. Others enter functionally unrelated foreign tubes, thereby changing the pattern of reinnervation (cross-shunting) and resulting in poor and incomplete recovery. If all fascicles are involved, there is complete loss of motor, sensory, and sympathetic function in the distribution of the nerve. Electrical studies show no conduction across the lesion 3 to 4 months from injury. Therefore, for any possibility of functional recovery, surgical resection of the injured segment, removal of scar tissue, and interpositional nerve grafting are required.
Grade 5
Grade 5 is the most severe grade of injury. There is complete transection, with loss of continuity of the entire nerve trunk, including the epineurium. In the proximal trunk, sprouting axons tangle and can form neuromas. These are usually laceration or severe stretch injuries. There is complete loss of motor, sensory, and sympathetic function in the autonomous distribution of the severed nerve. Wallerian degeneration occurs in the distal stump. Retrograde neuronal degeneration and axonal losses are higher than in grade 4 injury. Regenerating axons do not reach their original fasciculi and endoneurial tubes in the distal stump secondary to large injury gaps. Wasteful regeneration occurs because the axons escape into intervening tissue from the open end of the proximal stump. The distal stump shrinks over time because of denervation. Intervening scar causes obstruction, and cross-shunting occurs. The chance of any functional recovery requires surgical repair with or without nerve grafting. The quality of recovery depends on the degree of axon loss and disorganization of fiber pattern. Complete restoration is not possible.
Partial And Mixed Injuries (Combined)
Sunderland’s classification system2 can be used to describe partial and mixed injuries. In partial lesions, only a portion of the nerve fibers may be injured while the remaining are intact. In mixed (combined) injuries, all parts of the nerve are affected, with some areas more severely affected than others. For example, partial severance or subtotal fourth-degree involvement of the fascicles can coexist with minor degrees of injury to the remaining fibers of the nerve. Fascicles in continuity can contain fibers with neurapraxic, axonometric, or both types of injuries inside the same bundle. There may be complete loss of motor, sensory, and autonomic function in the distribution of the affected nerve(s). The duration, course, and quality of recovery depend on the severity, number, and type of injury to each individual nerve fiber or fascicle.
Preoperative Assessment
History
The history and physical examination with a detailed neurologic examination should be the initial step for assessing peripheral nerve injuries. This aids in localizing the lesion to the peripheral or central nervous system. Additional tests or studies may be needed to evaluate for systemic disease, toxicities, and lesions in bone or connective tissue. The history should be thorough. The symptoms can involve pain, paresthesias, dysesthesias, weakness, and/or loss of autonomic function.
Neurologic Examination
A thorough physical examination should follow with emphasis on the muscles supplied by the autonomous zone of the injured nerve. This involves a detailed sensory and motor examination. Muscle strength should be graded. The sensory examinaiton should test for light touch, pinprick, two-point discrimination, vibration, and proprioception.
Hoffman-Tinel Test
The Hoffman-Tinel test is used to evaluate and monitor the progress of spontaneous regeneration of sensory axons. It is performed several months after injury, when wallerian degeneration has already started. When the nerve is percussed, paresthesias are felt in the distribution of the nerve. This is due to mechanosensitivity of unmyelinated axons early in regeneration. Their disappearance after myelination indicates growing fibers after spontaneous recovery.12
When deciding on when to do exploratory surgery one must determine if the injury is open or closed and whether it is acute or chronic and clean or dirty. Most open traumatic peripheral nerve injuries are caused by acute clean, sharp lacerations. These require immediate exploration and closure with end-to-end epineural sutures if possible (Fig. 195-1).13 Repair of a blunt injury should be delayed to allow the full extent of scar to develop. Most closed traumatic injuries are in continuity. Early after injury, neurapraxic and axonotmetic injuries should be followed for 3 to 4 months with serial clinical and electrodiagnostic examinations to confirm objective signs of spontaneous regeneration and recovery. The EMG findings are normal in neurapraxic lesions. Surgical exploration is indicated if the clinical examination and electrical studies show no improvement. Intraoperative assessment with recording of nerve action potentials (NAPs) is paramount to determine whether resection with or without nerve grafting, internal neurolysis, or only external neurolysis is appropriate.
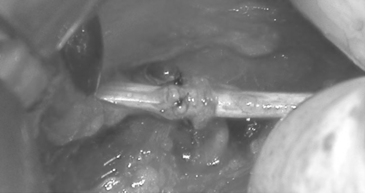
FIGURE 195-1 Intraoperative photograph demonstrating tension-free, end-to-end suture anastomosis of an obturator nerve that was sharply transected during an abdominal hysterectomy.
The critical window for muscle reinnervation is within 2 years after injury for any chance of useful functional recovery. Chronic denervation results in irreversible atrophy.9 Therefore, the evaluation and decision regarding surgery should be done ideally 3 to 4 months after injury.9,12 Clinical and electrodiagnostic studies are similar in complete axonotmetic or neurotmetic injuries. However, axonotmetic injuries undergo spontaneous regeneration whereas neurotmetic injuries in continuity or discontinuity must undergo immediate surgery to reestablish continuity for any chance of useful functional recovery. Surgery is reserved for severe intractable symptoms and neurologic deficits.
Electrodiagnostic Studies
EMG is the clinical study of the electrical activity of muscle.14 It helps to define the etiology of muscle weakness and evaluate the recovery progress during nerve regeneration and muscle healing. It can be performed as early as 10 days after injury but will certainly be accurate by 4 to 6 weeks after injury. It will be normal in neurapraxic injuries and abnormal in axonotmetic or neurotmetic injuries.9,10 Abnormal findings such as fibrillations, fasciculations, and positive sharp waves may be present.8 EMG evidence of reinnervation and regeneration precedes voluntary muscle contraction by several weeks.7,9,14–16 The duration for regeneration depends on the distance from the injury to the end organ.17
In brachial palsy, EMG in combination with nerve conduction studies can help distinguish preganglionic (including avulsion) from postganglionc cervical root injury or plexus injury. Motor axonal injury shows denervation fibrillation potentials beginning approximately 3 weeks after injury.7,9,14 Nerve conduction studies (NCS) assess peripheral motor and sensory nerve function by evaluation of the electronic potential following nerve stimulation.14,18 They can help localize peripheral nerve lesions and differentiate them from disorders of muscle or neuromuscular disease. They also help delineate segmental demyelination from axonal degeneration as in neurapraxic and/or axonometric injuries as early as 1 week after injury. Serial studies are done to monitor progression of disease.
In brachial plexus lesions, sensory nerve evoked potential (SEP) recordings can help differentiate between proximal preganglionic (intraforaminal, intraspinal, intradural) and distal postganglionic (extraforaminal, extraspinal, extradural) lesions. It is well understood that the survival of the sensory nerve fibers depends on the cell bodies within the dorsal root ganglion. The motor nerve fibers depend upon the nerve’s cell bodies within the spinal cord.19
In preganglionic brachial plexus root avulsion from the spinal cord, the cells in the dorsal root ganglion and the peripheral nerve fibers are intact and evoke a sensory nerve action potential (SNAP). SNAPs are absent in postganglionic lesions because of wallerian degeneration of injured nerve fibers. However, these recordings only evaluate the function of the posterior dorsal root and not the ventral root. Therefore, the integrity of an isolated ventral root injury or of combined preganglionic and postganglionic lesions cannot be determined using only this method.20–24 The presence of an intact SNAP in the face of severe EMG changes indicates a preganglionic lesion. If there is absence of the SNAP then the lesion must be postganglionic, but this does not preclude the presence of a combined pre- and postganglionic lesion.
At present, the indirect method of evaluating motor root fibers is via EMG testing of individual muscles or groups of muscles for function via contractile responses. Therefore, the combination of EMG and SNAP recordings is more valuable than any one study for detecting spinal root or cord injury. However, motor evoked potentials (MEPS) are considered the gold standard for evaluating motor function.25 MEPS are produced via transcranial stimulation (electrical or magnetic) of the contralateral motor cortex and recording from the spinal nerve (neurogenic MEP) at its exit from the intervertebral foramen and the EMG from involved muscles (CMAP) or spinal cord (D-wave; direct corticospinal wave).23–27 Transcranial stimulation (TcMEP) produces contralateral motor activity representative of functioning corticospinal tracts. Changes in MEPs are much more sensitive than those in somatosensory evoked potentials (SSEPs) in detecting postoperative motor deficits. This is especially important in isolated motor injury with no change in SSEPs.25,28
The limitation of TcMEPs is that they cannot be continuously monitored. Monitoring requires total intravenous anesthesia for adequate signals, and TcMEPs are technically more difficult to obtain than SSEPs.25 However, continuous monitoring with spontaneous EMG allows detection of possible injury to the spinal nerve, plexus, or roots during cervical or lumbosacral spine procedures and during minimally invasive procedures. Triggered EMG is helpful in localizing functional neural structures in the surgical field as well as evaluating injury to nerve roots after medial wall breach during pedicle screw instrumentation.25 A large prospective study of 1055 patients demonstrated that the combination of EMG, SSEPs, and MEPs increased the chances of detecting neurologic injury during cervical spine procedures.28 The consensus is that the use of multimodality neurophysiologic monitoring in spinal procedures aids in preventing postoperative neurologic deficits.
SSEP studies evaluate the dorsal column and medial lemniscus pathways, which helps assess proximal spinal nerve root injuries,9,29–31 spinal cord tumors, and other spinal disorders.32 SSEP involves electrical stimulation of peripheral nerves (median nerve, common peroneal nerve, posterior tibial nerve, or ulnar nerve). The signals are recorded centrally over the brain in the contralateral parietal cortex or in the spinal cord. A dorsal root (preganglionic) avulsion injury would have an absent SSEP in the presence of a positive postganglionic SNAP because the distal sensory fibers are still connected to the dorsal root ganglion.29,30 In distal postganglionic lesions, stimulation of the root proximal to the level of injury results in a positive SSEP in the absence of a SNAP.29
SSEPs can be invaluable for intraoperative monitoring.25,27,32 Early recognition of changes can signify possible injury and give the surgeon time to make the necessary adjustments to prevent postoperative neurologic deficits. According to Dinner and colleagues,32 significant changes such as loss in signal or decrease in amplitude in monitored SSEPs correlated with a higher possibility of postoperative neurologic deficit (three of seven SSEPs, 43%). If no changes occur, the chances of a deficit are low (1.8%, 4 of 213 cases). Sensory nerve evoked potentials (SNEPs) are useful in the resection of some intraneural tumors by distinguishing and functional from nonfunctional fascicles, thereby rendering safer the separation and resection of tumor from functional fascicles. SNEP is valuable in the surgical management of peripheral neural sheath tumors of major nerves including the brachial plexus.33,34 This is significant, especially in type 1 neurofibromatosis (NF1), which previously was thought to be unsafe for resection.3
Imaging Studies
Adjunctive tools for the neurologic assessment of peripheral nerve disorders include electrodiagnostic studies; EMG and NCS, magnetic resonance imaging (MRI), computed tomography (CT), myelography, CT myelogram, magnetic resonance neurography (MRN), and plain x-rays.
Plain x-rays can reveal some tumors, cysts, injury to the diaphragm (phrenic nerve), and skeletal fractures where specific locations can imply concomitant nerve injury. However, there is poor delineation of peripheral nerves from surrounding tissue.35
CT can identify soft tissue tumors, cysts, and skeletal fractures or hemorrhage and can help to delineate some peripheral nerve tumors from adjacent blood vessels or tissues, although in most cases visualization is usually poor.36
MRI can delineate the anatomic details of soft tissue, peripheral nerves, and perineural tissue much better than CT. It can give T1 and T2 multiplanar images (axial, coronal, sagittal) of large bulky tumors.37 Coronal and sagittal views show the full linear extent of the tumor with fewer cuts and produce clearer images than CT. CT can require several axial cuts. MRI can be used in patients with nonferromagnetic prosthetics. It is also useful in evaluating and diagnosing traumatic, compressive, and inflammatory lesions of peripheral nerves.35,37 Inadequate images are usually secondary to spatial and resolution limitations and motion artifacts.20,38 Traumatic peripheral nerve injury can result in axonal losses with degeneration and muscle denervation followed by muscle atrophy, if the injury is chronic. MRI of muscle distal to the lesion can show increased T2 and short tau inversion recovery (STIR) signals in the denervated muscle. This correlates well with clinical examination (grade 3 weakness) and EMG findings of fibrillation potentials. These signals can be seen as early as 4 days after nerve injury.39 MRI can in some instances distinguish neurapraxic from axonometric and neurometric injury.39,40 Both STIR and T2-weighted images are normal in neurapraxic lesions.
In axonometric injuries, signs of reinnervation and recovery are reflected by normalization of signals, but the MRI findings can lag.39 Therefore surgical exploration with intraoperative electrical studies are necessary to appropriately assess for axonometric and neurometric injury. This is especially important because neurometric injuries require surgical repair for any chance of useful functional recovery. Increased T2 and STIR signals are also seen in chronically denervated muscles and nerves as in entrapment neuropathies such as carpal tunnel syndrome of the median nerve or in ulnar nerve entrapment at the elbow. In carpal tunnel syndrome these findings correlate well with EMG, nerve conduction studies, clinical examination, and operative findings; however, in the ulnar nerve, where symptom localization with electrodiagnostic studies is difficult, the imaging correlation is less clear.40–43 For reasons unknown, some abnormal MRI signals normalize over months even without signs of recovery.42
In a significant spinal study, MRI with and without contrast (gadolinium) was shown to quantify lumbar peridural fibrosis 6 months after discectomy for herniated lumbar disc.16 The increased scar correlated well with an increased probability of recurrent radicular pain. The imaging in this case looked more at the scar tissue than the nerve itself.
Despite all of the advantages of MRI and CT, in some instances it is still difficult to distinguish vascular structures and surrounding tissues from peripheral nerves. Despite the superior contrast enhancement of tumors with MRI, its utility is variable in peripheral nerve disorders.44 The development of MRN has helped to resolve this by its ability to directly visualize the normal fasicular structure and pattern of the nerve by the interfascicular and intrafascicular suppression of non-neural structures such as fat, muscle, and blood vessels.26,35,44 Therefore, the nerve fascicle signal predominates. Images are obtained using phased-array coils, fast spin echo (FSE) sequences, and fat-suppressed T2-weighted images. Signals are increased (hyperintense) in intraneural disease and correlate with clinical examination, intraoperative electrodiagnostic studies, and pathology.38 T1 spin-echo pulse sequences can show the course of the nerve in relation to adjacent structures.38,44 Longitudinal and cross-sectional fascicular images aid in distinguishing intraneural from perineural masses such as tumors or cysts.44 In one study, preoperative MRN distinguished nonfunctioning and functioning fascicles from tumor, allowing complete resection in 72.5% of patients with NF1.38 After traumatic peripheral nerve injury, MRN can assess nerve continuity and aid in surgical planning.44,45 Cudlip and colleagues17 used the rat sciatic nerve crush model to follow the MRN signal changes immediately after injury and throughout the degenerative and regenerative processes. The signal changes correlated with the functional deficits. Normalization of the signals correlated with functional recovery. Early after severe axonometric or neurometric injury, T2 signals are increased at and distal to the lesion. Electrical studies show no nerve conduction across the lesion.
After surgical repair of a peripheral nerve, the course of healing can be followed by the signal changes.26,44 After several weeks or months, signs of regeneration can be confirmed by clinical examination, decreased fibrillations on EMG, and distal normalization of the T2 signals. However, for reasons unknown, these signals can remain high in interpositional grafts.26 Overall, these findings show that MRN correlates well with the clinical and intraoperative electrodiagnostic findings during the course of recovery.26,38
MRI, CT, and myelography can be very sensitive but not specific in detecting multilevel degenerative disc disease of the cervical and lumbar spine.43 There are many false positives in asymptomatic patients. In these cases, MRN could be considered. It may be a useful adjunct because it has already been shown to help evaluate and diagnose traumatic and compressive lesions of peripheral nerves, nerve roots, and the brachial plexus.35,45 The MRN appearance can differ in the symptomatic root, allowing identification of the pathologic spinal level.
The assessment of acute brachial paralysis secondary to cervical root avulsion can be complex and difficult. It requires a thorough clinical evaluation, imaging, and electrodiagnostic studies. Traumatic meningoceles (pseudomeningoceles) on myelogram studies were considered to be pathognomonic for intradural (intraspinal) spinal root avulsions (Fig. 195-2).20,22 However, surgical exploration in some cases revealed intradural root avulsion even with a normal preoperative myelogram. Currently, CT myelogram done in 1- to 3-mm axial slices is considered accurate for assessment of avulsion of spinal roots, especially in the brachial plexus.20 A significant study has shown that 85% of roots evaluated by preoperative CT myelogram correlate to intraoperative findings of intradural avulsed cervical spinal roots compared to 52% evaluated by MRI.20,42 Avulsed spinal roots are mistakenly diagnosed more commonly at C5 or C6 in both imaging studies. MRI is less reliable at visualizing the intradural (intraspinal) dorsal and ventral roots.20 Intradural fibrosis or traumatic meningoceles (pseudomeningocele) may be contributing factors for poor visualization. For inconclusive or complex injuries of the brachial plexus, MRN should be considered in conjunction with conventional MRI and electrophysiologic studies to assist with localizing nerve root and brachial plexus lesions.45
Operative Management
The goal of any nerve repair is to reestablish continuity and provide an optimal environment and conditions for adequate axonal regeneration that will ultimately produce peripheral innervation patterns similar to the original ones and leading to useful functional recovery. In the latter half of the 20th century, many innovative techniques and instrumentation were developed to assist in achieving this goal. Sunderland11 suggested using the operating microscope in nerve repairs, but it did not come into favor clinically until the 1960s.13 Because most injuries are associated with some form of external fibrosis, the initial step in surgical decompression involves an external neurolysis. The procedure required is dictated by the type and severity of injury. Some of these are described later in further detail.
External Neurolysis
External neurolysis is indicated when electrical studies fail to improve or when they worsen after 3 to 4 months from the time of injury. This could be secondary to nerve compression, constriction, or tethering by scar tissue.1,14,46 Fibrosis can slow down and/or prevent spontaneous regeneration, resulting in poor, if any, recovery. It can also cause significant neuritic pain. Preoperative electrical studies are done to localize the area(s) of slowing or conduction block. Under general anesthesia, fibrotic tissue is resected until healthy planes are established around the nerve lesion and immediate injury site. Electrical studies are repeated intraoperatively to assess for any improvement. A nonconducting lesion requires resection and end-to-end repair or interpositional nerve grafting.
Internal Neurolysis
Internal neurolysis is usually done for patients with severe neuritic pain and incomplete losses distal to the injury.14 Electrical studies show no improvement or worsen after 3 to 4 months from the time of initial injury.14 The lesion must be in continuity and conduct a compound NAP preoperatively and intraoperatively. These are usually partial axonotmetic injuries with or without regeneration.8 After external neurolysis, recordings are repeated to identify the reduced conductive areas. These sites undergo an interfascicular dissection, with resection of fibrotic tissue surrounding individual fascicles.8 Each fascicle is tested individually for function and resected if nonconducting (Fig. 195-3). For partial lesions, a split (partial) repair is done in which the nonconducting portion is resected and repaired end-to-end or with an interpositional nerve graft if the defect is too large.14,46,47 Decompression by manipulation of the fascicles can induce fibrosis, which can cause injury to the regenerating axons as well as the normal fascicles, thereby further delaying or worsening recovery. The surgeon must weigh the risk of possible irreversible muscle loss from chronic denervation against that of surgery.
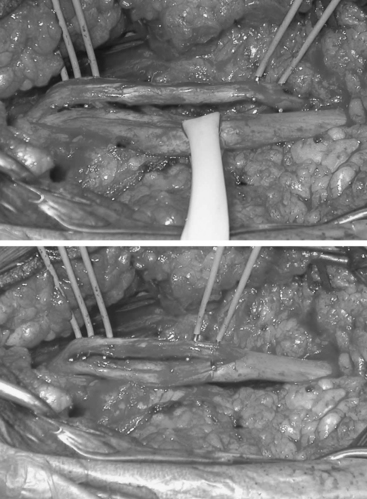
FIGURE 195-3 Intraoperative photo of a sciatic nerve at a thigh level that several months before had been injured during a lower extremity soft tissue tumor excision. Surgical exploration was performed because spontaneous recovery had not yet occurred. At operation, the nerve was first subjected to an external neurolysis and then separated into its tibial and peroneal divisions. An internal neurolysis divided each division into several fascicular bundles, each of which underwent nerve action potential recordings.
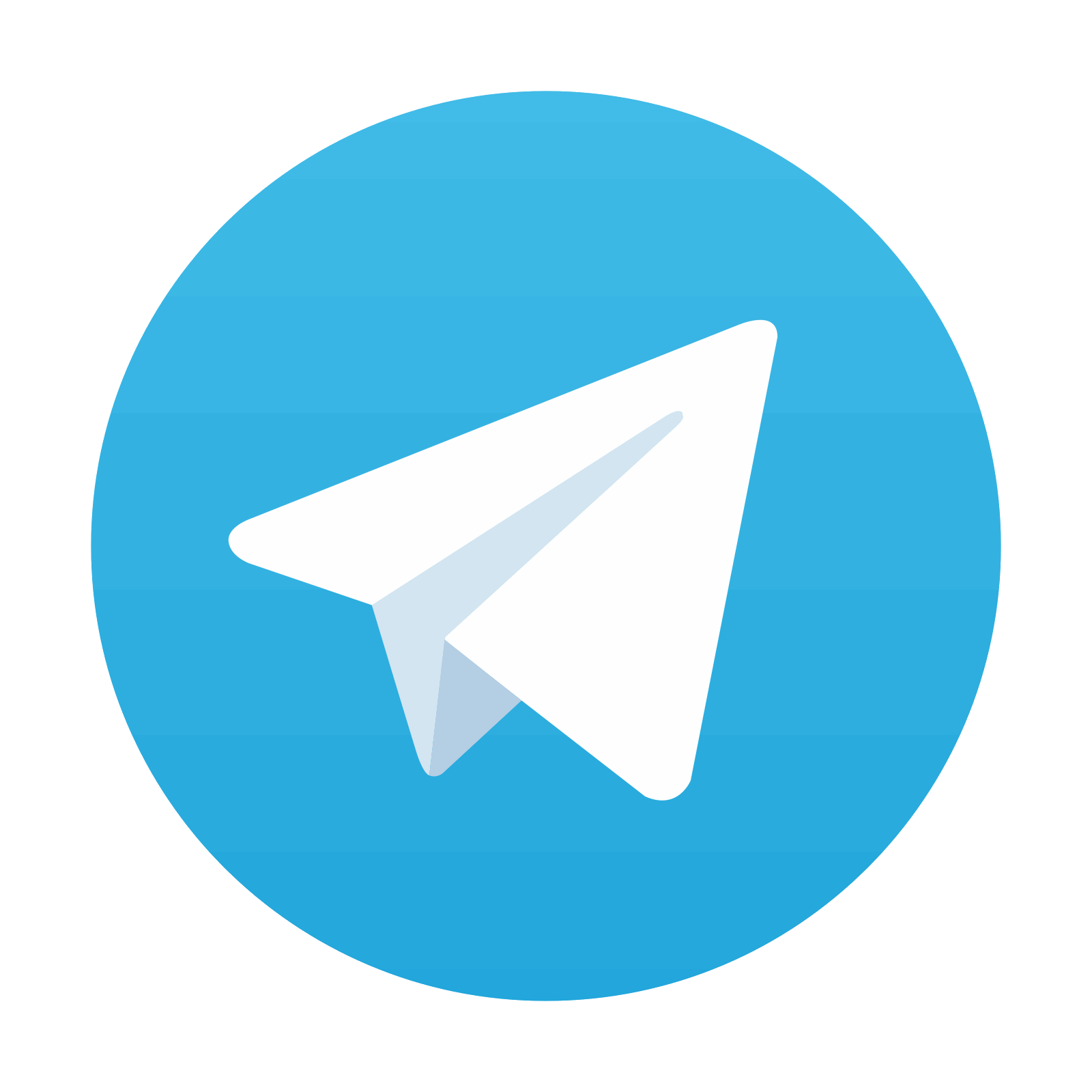
Stay updated, free articles. Join our Telegram channel

Full access? Get Clinical Tree
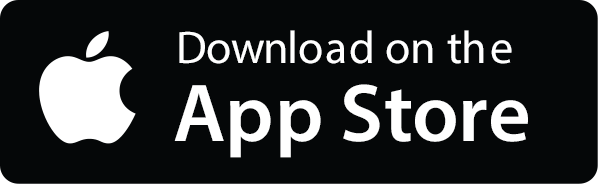
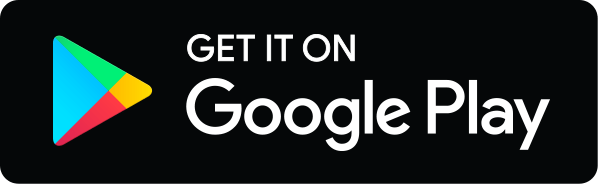