Keywords
Inherited metabolic diseases, sphingolipidoses, glycoproteinoses, amino acids, fatty acids, mitochondrial diseases, peroxisomal diseases, lipids, glycogen storage diseases, Leigh disease
Introduction
Peripheral neuropathy is associated with numerous inherited metabolic diseases, such as Fabry disease (trihexosylceramide lipidosis) where it may be the presenting complaint, or adrenomyeloneuropathy, in which it may play a relatively minor role in contrast to the more prominent and progressive spastic paraparesis. In the mucopolysaccharidoses, neuropathy may represent a complication of the underlying storage disease, such as an entrapment neuropathy. This chapter describes the specific inherited metabolic disorders in which peripheral neuropathies are common. While the biochemical and molecular bases of these disorders are well established, effective treatment is quite elusive without specific therapies directed at the primary metabolic defect. In some cases, pharmacologic interventions may be helpful.
The inherited metabolic diseases in which a peripheral neuropathy is prominent may be separated into several categories ( Table 19.1 ) including storage diseases, disorders of amino acid and fatty acid metabolism, and mitochondrial cytopathies.
Category | Disease | Stored Material | Biochemical Defect | Molecular Defect | Chromosome |
---|---|---|---|---|---|
| |||||
Sphingolipidoses | Krabbe disease | Galactosylceramide | Galactosylceramide β-galactosidase | GLAC | 14q25-31 |
Metachromatic leukodystrophy | Sulfatide | Sulfatide sulfatase (arylsulfatase A) | ARSA | 22q13.31-ter | |
Fabry disease | Trihexosylceramide | Trihexosylceramide α-galactosidase | GLA | Xq22.1 | |
Mucopolysac-charidoses | Multiple (see Table 19.3 ) | See Table 19.3 | See Table 19.3 | See Table 19.3 | See Table 19.3 |
Glycoprotein-oses | Fucosidosis | Oligosaccharides | α-fucosidase | 1p34.1-36.1 | |
α and β mannosidosis | Oligosaccharides | α and β mannosidase | FUCS1 MAN2B1 , (α) MANBA , (β) NAGA | α-19p13.2-q12 | |
β-4 | |||||
Sialidosis type II | Oligosaccharides | Sialidase | NEU | 6p21.3 | |
Schindler disease | Oligosaccharides | N-acetylgalactosaminidase | AGB | 22q13.1-13.2 | |
| Adrenomyelo-neuropathy | Very long chain fatty acids | VLCFA CoA synthetase | ALDP | X128 |
Refsum disease | Phytanic acid | Phytanic acid oxidase | PHYH | 10p | |
Hyperoxaluria | Calcium oxalate | Alanineglyoxylate aminotransferase | AGT | 2q36-37 | |
| Cerebrotendinous xanthomatosis | Cholestanol | Cholesterol 27-hydroxylase | CTX | 2q35 |
Cholesterol esters | |||||
Tangier disease | Unknown | ABCA1 | |||
Abetalipoproteinemia | Unknown | APOB, PCSK9, MTP | |||
| Adult polyglucosan body disease | Glycogen | Amylo-1, 4-1, 6 transglucosidase | GBE1 | 3p12 |
| Tyrosinemia type I | Tyrosine/succinyl-acetone | Fumarylaceto-acetate hydrolase | FAH | 15 |
| LCHAD | 3-Hydroxy dicarboxylic aciduria | Trifunctional protein and long-chain 3-hydroxy-acyl-CoA dehydrogenase | HADHA/HADHB | 2p23.3 |
Leigh | Lactate/pyruvate | Multiple enzymes | Multiple nucleotide | ||
NARP | Lactate/pyruvate | ATP synthase subunit 6 | 8993 (mtDNA) | ||
| Acute intermittent porphyria | δ-Aminolevulinic acid | Porphobilinogen deaminase | PBGD | 11q24.1-q24.2 |
δ-aminolevulinic acid dehydratase | ALAD | 9q34 |
Lysosomal Storage Diseases
Sphingolipidoses
Peripheral neuropathy is an integral component of several disorders of sphingolipid metabolism including Krabbe disease, metachromatic leukodystrophy, and Fabry disease. Histologic evidence of lipid storage in peripheral nerve also occurs in rapidly progressive forms of GM1 and GM2 gangliosidoses, infantile Gaucher disease, Niemann-Pick disease, and Farber disease, but in these disorders nerve involvement is not clinically evident, most likely due to rapid central nervous system progression. These conditions will therefore not be discussed further. Involvement of the peripheral and autonomic nervous systems has been recently recognized in chronic GM2 gangliosidosis. This rather uncommon form of the disease typically presents in late childhood or adolescence as ataxia, dystonia, motor neuron disease, and dementia. Nonetheless, peripheral nerve disease may be sufficient to produce clinical symptomatology and objective evidence of diffuse sensorimotor neuropathy with reduced NCV and amplitude ( Table 19.2 ).
Category | Disease | Neuropathic Features |
---|---|---|
| ||
Sphingolipidoses | Krabbe disease | Schwann cell inclusions |
Segmental demyelination | ||
Metachromatic leukodystrophy | Schwann cell sulfatide (metachromatic) accumulation | |
Segmental demyelination | ||
Fabry disease | Lamellar inclusions in perineurial cells | |
Axonal loss | ||
Mucopolysaccharidoses | Multiple (see Table 19.3 ) | Entrapment neuropathy |
Glycoproteinoses | Fucosidosis | Entrapment neuropathy |
Mannosidosis | Axonal inclusion | |
Sialidosis type II | Schwann cell vacuoles | |
Axonal inclusions | ||
Schindler disease | Axonal spheroids | |
| Adrenomyeloneuropathy | Schwann cell inclusions |
Segmental demyelination | ||
Refsum disease | Schwann cell inclusions | |
Onion-bulb formation | ||
Segmental demyelination | ||
Hyperoxaluria | Axonal degeneration | |
Segmental demyelination | ||
| Cerebrotendinous xanthomatosis | Axonal loss |
Schwann cell vacuoles | ||
Tangier disease | Axonal loss | |
Schwann cell vacuoles | ||
Abetalipoproteinemia | Axonal degeneration | |
Segmental demyelination | ||
| Adult polyglucosan body disease | Schwann cell inclusions (polyglucosan bodies) |
| Tyrosinemia type I | Axonal loss |
Segmental demyelination | ||
| LCHAD | Axonal loss |
Segmental demyelination | ||
Leigh | Segmental demyelination | |
NARP | Segmental demyelination | |
| Acute intermittent | Axonal degeneration |
porphyria | Segmental demyelination |
Krabbe Disease: Globoid Cell Leukodystrophy (Galactosylceramide Lipidosis)
Clinical Characteristics
Globoid leukodystrophy (GLD), described in 1916 by the Danish physician Knud Krabbe, has four clinical presentations, based upon age of onset. The early infantile form (3–6 months of age) presents with episodes of rigidity and opisthotonus, often in response to auditory, tactile, or visual stimuli; progressive psychomotor retardation; feeding difficulties; febrile episodes; spastic paraparesis; and optic atrophy. Results from an international registry demonstrated abnormalities in most children with early infantile form; 3-year survival is 14%. The late infantile form (6 months to 3 years) presents with ataxia, generalized hypertonicity, psychomotor regression, and visual failure. The combined incidence of these two forms is estimated at 1:100,000 to 1:200,000. The juvenile form (3–10 years of age) presents with dystonia, visual loss, and progressive spastic paraparesis, and the adult form with progressive spastic paraparesis, dementia, and optic atrophy.
Peripheral neuropathy is a prominent feature in the infantile forms, perhaps even before clinical symptoms, but is mild or absent in the juvenile and adult forms. The peripheral neuropathy is associated with elevated levels of cerebrospinal fluid protein (up to 400 mg/dL) and reduced nerve conduction velocities (NCV=10 m/s or lower). Distal latencies may be mildly prolonged. Compound motor action potential (CMAP) amplitudes may be mildly to moderately diminished. Electromyography (EMG) is normal. An infant with prenatal diagnosis demonstrated slowed NCVs at 7 weeks. Patients with the late-onset forms rarely show clinical evidence of peripheral nerve involvement, and their cerebrospinal fluid protein levels are normal or only mildly increased, but NCVs are decreased in most instances. Both axonal and demyelinating patterns have been reported in late onset Krabbe disease ; the latter occurs in about 80% of those who exhibit peripheral neuropathy (about 60% of adults).
Pathology
The main histopathological changes in the central nervous system are extensive demyelination, gliosis, and the presence of characteristic multinucleated cells, referred to as globoid cells. These phagocytic cells can be produced experimentally in rats by injecting galactosylceramide into the spleen or brain white matter. Peripheral nerves demonstrate segmental demyelination and remyelination with marked reduction in the density of myelinated fibers and a relative predominance of small thinly myelinated fibers ( Table 19.2 ). Sural nerve biopsies demonstrate Schwann cell cytoplasmic inclusions similar to those in the brain; however, classic globoid cells are not found.
Biochemistry
GLD is due to deficiency of the lysosomal enzyme galactosylceramidase (galactosylceramide-β-galactosidase), which metabolizes galactosylceramide to ceramide and galactose as well as galactosylsphingosine (psychosine) to sphingosine and galactose. Diagnosis is made by measurement of GALC activity in white blood cells, cultured skin fibroblasts, amniocytes, or chorionic villus cells. Psychosine accumulation in patients with GLD is a key factor in the pathogenesis of the disease. Animal model studies suggest an axonopathy related to psychosine.
Molecular Defect
GLD is an autosomal recessive disorder in which the gene GALC maps to 14q25-31. One hundred and forty-seven disease-causing mutations are known. The most common, a 502T/del, is present in 40% of GLD patients of European origin. The second most frequent mutation, a C>T change at nucleotide 1538, is found in approximately 10% of patients with the infantile phenotype. Mutation analysis is the most reliable method of carrier identification.
Management
Hematopoietic stem cell transplantation improves survival in babies with Krabbe disease treated within the first few weeks of life, but its benefits may be transient. Hematopoietic stem cell transplantation (HSCT) can be effective in the juvenile form of the disease but does not improve nerve conduction velocities.
Metachromatic Leukodystrophy (Sulfatide Lipidosis)
Clinical Characteristics
Metachromatic leukodystrophy (MLD) presents in late infancy (most commonly), adolescence or adulthood. Onset is between 1 and 3 years of age with loss of previously acquired motor skills such as ambulation, followed later by ataxia, dysarthria and dysphagia, and impairment of cognitive function, vision, and hearing. The deficits are progressive, leading to death after several years. Occasionally, MLD presents in infancy as peripheral neuropathy without overt CNS findings ; cranial neuropathy (esotropia and facial weakness) has also been reported as a presenting feature, associated with cranial nerve enhancement on MR imaging. Neurologic examination shows evidence of both central nervous and peripheral nerve dysfunction, with hypotonia and diminished muscle stretch reflexes and positive dorsal plantar responses, ataxia, dystonic movements, and nystagmus. The juvenile form is similar to the late infantile form but with slower progression. In the adult form, behavioral and cognitive changes may precede motor changes by years or even decades. The frontal lobe syndrome in adult MLD may include disinhibition, impulsivity, poor judgment, emotional lability, social inappropriateness, and poor attention span.
Peripheral nerve involvement is a prominent feature in all forms of MLD, the combined peripheral and central nervous system involvement being an important diagnostic clue. NCVs are significantly decreased (<10 m/second) and distal latencies may be prolonged. Sural nerve biopsy shows metachromatic staining. In juvenile metachromatic leukodystrophy, diminished NCVs may prompt evaluation for combined central and peripheral nervous system demyelination. A rapidly progressive ascending paralysis has been described in an 18-month-old girl with MLD. Respiratory failure and ptosis responded to intravenous immunoglobulin, but limb weakness did not, suggesting that the child had an immune-mediated neuropathy superimposed on the chronic neuropathy of MLD.
Pathology
The pathology of MLD consists primarily of demyelination and metachromatic granule deposition in the central and peripheral nervous system ( Figure 19.1 ). These changes can also be seen in the kidney, urine (urinary sulfatide excretion is increased), and other tissues. Viewed by electron microscopy the metachromatic granules present a hexagonal honeycomb pattern, and are referred to as tuffstone bodies. Peripheral nerves show segmental demyelination, with metachromatic granules singly or in clusters in Schwann cells ( Table 19.2 , Figure 19.2 ), in endoneurial macrophages, and to a lesser extent in the Remak cells associated with unmyelinated nerve fibers. In addition to the expected metachromatic granules revealed by cresyl violet staining, one study of 40 cases of nerve biopsies in MLD showed accumulation of orthochromatic granules in perivascular macrophages, suggesting storage of multiple species of glycosphingolipids.


Biochemistry
The key abnormality in MLD is the accumulation of sulfatide (galactosylceramide-3-sulfate), the metachromasia due to the sulfate group. Deficiency of the lysosomal enzyme arylsulfatase-A (ASA), which cleaves sulfatide to galactosylceramide and sulfate, is the most common cause of sulfatide accumulation, with an estimated incidence of 1:40,000. Diagnosis of ASA deficiency can be determined in white blood cells, cultured skin fibroblasts, amniocytes, or chorion villus cells. Great caution must be used to distinguish MLD from a condition referred to as pseudoarylsulfatase deficiency (PSD). PSD is a common and entirely benign condition in which ASA activity is reduced, at times to levels nearly as low as in MLD. Persons with PSD do not display any of the clinical manifestations of MLD. Distinction between PSD and MLD is crucial in prenatal studies and in asymptomatic infants or adults. Molecular analysis and measurement of urinary sulfatide levels aid the distinction. Urinary sulfatide levels are increased in MLD but not PSD. Less commonly, sulfatide accumulation is due to multiple sulfatase deficiency, in which at least seven sulfated lipids or mucopolysaccharides accumulate. The third and least common cause is a deficiency of saposin B, one of the sphingolipid activator proteins, which is required for the biological activity of ASA. Mutations in the PSAP gene, which encodes saposin B, are associated with a wide variety of phenotypes.
Molecular Defect
All forms of MLD are transmitted as autosomal recessive traits, the gene, ASA , mapping to 22q13, with more than 150 mutations identified. Mutations that abolish function completely cause the severe late infantile phenotype. Missense mutations, in which up to 3% to 5% of normal activity is maintained, tend to be associated with the adult form of the disease. Individuals who are heterozygous for a null mutation and one of the milder missense mutations often have the juvenile phenotype of MLD.
Management
Bone marrow transplantation (BMT) can stabilize or slow the rate of progression of central nervous system dysfunction, and stabilize peripheral nerve involvement in patients with the juvenile, but not the early infantile presentation of MLD. BMT is recommended only for patients with relatively mild nervous system involvement, or for asymptomatic patients identified by testing of at-risk relatives, with care taken to differentiate MLD from PSD. The procedure is not recommended for patients with advanced involvement, as it is associated with a high risk of additional worsening of the disability. Gene therapy is under investigation in cultured cells and in animal models. Enzyme replacement therapy is currently in human trials, after promising results in animal models.
Cholecystectomy is occasionally required in those in whom the accumulated sulfatide has formed gallstones.
Fabry Disease (Trihexosylceramide Lipidosis)
Clinical Characteristics
Trihexosylceramide lipidosis (angiokeratoma corporis diffusum universale, Fabry disease) was described independently in 1893 by Fabry and Anderson. Among the sphingolipid storage diseases, it is the only X-linked disorder. The principal clinical manifestations involve skin, heart, kidney, and peripheral nerve.
The hallmark of Fabry disease is its cutaneous manifestation— angiokeratoma —violaceous angiectatic lesions over the genitalia, thighs, buttock, back, and lower abdomen. Similar lesions may be seen in the oral mucosa. Angiokeratomata have also been described in fucosidosis, β-mannosidosis, galactosialidosis, and Schindler disease, and are not specific for Fabry disease. Fabry disease has its onset most commonly in adolescence or early adulthood. Despite significant chronic medical issues, survival is often quite prolonged. Ultimately, renal or cardiac involvement will determine longevity. Cardiac manifestations include left ventricular hypertrophy, valvular dysfunction (especially mitral insufficiency), and conduction abnormalities. Death usually occurs in the fourth or fifth decades.
Chronic renal disease poses the major clinical problem. As such, Fabry disease had been regarded as the best candidate among the sphingolipidoses for direct therapeutic intervention. However, improvement of renal function with dialysis or renal transplantation has not reversed other aspects of the disease.
The most common neurological problem is a painful peripheral neuropathy, which generally involves the distal extremities (acroparesthesias). Clinical features include both severe crises of lancinating pain and chronic, unremitting pain. The painful crises may be precipitated by exercise, fatigue, stress, or exposure to sunshine or hot weather. Decreased sweating (hypohidrosis) leads to temperature intolerance and untoward responses to sun exposure.
Other common features are corneal and lenticular opacifications and retinal vasculopathies. CNS vaso-occlusive events may be noted, and Fabry disease should be considered in the differential diagnosis of stroke in the young individual. Cranial imaging often demonstrates changes consistent with stroke, even the absence of a history of stroke-like events. Occasionally, lymphedema and episodic diarrhea may be significant problems. Both are attributed to lipid storage.
Female carriers (heterozygotes) of trihexosylceramide lipidosis may develop signs similar to those in affected males, although typically much later in adulthood. Corneal opacifications are the most common signs, being present in nearly three-fourths of carriers. Other features are less prevalent but equally severe to those seen in men.
Cardiac Variant
A variant form of Fabry disease is limited predominantly to cardiac involvement with onset typically after age 40, without other features of Fabry disease except for mild proteinuria. The investigation of unexplained proteinuria in these males led to the recognition of this milder variant .
Pathology
Lipid materials accumulate in vascular smooth muscle and endothelium, kidney, cornea, peripheral nerves, and in neurons of the autonomic nervous system, both central and peripheral. Electron microscopy of peripheral nerves reveals lamellar inclusions in perineurial cells. Similar lamellar inclusions can be demonstrated in vascular smooth muscle. The degree of peripheral nerve involvement can be easily assessed by analysis of biopsy specimens ( Table 19.2 ). A decrease in small peripheral sensory neurons may be noted.
Biochemistry
Deficient α-galactosidase (trihexosylceramide α-galactosidase) activity is the basis for Fabry disease. Trihexosylceramide is an important membrane sphingolipid in kidney and the first intermediate in the degradation of globoside, the prominent sphingolipid of red cell membranes. In Fabry disease, trihexosylceramide accumulates in kidney, liver, and lung from 30 to 300 times normal levels. A lesser degree of digalactosylceramide accumulation is also seen, primarily in the kidney. Diagnosis can be made in leukocytes and cultured skin fibroblasts, and numerous mutations are described.
Management
The painful and disabling dysesthesias of hands and feet may be treated with carbamazepine, phenytoin, gabapentin, or pregabalin. The systemic clinical manifestations, including neurological problems, arise from vaso-occlusion due to sphingolipid deposition in vascular endothelial cells, and may be difficult to treat. Renal transplantation may be required for end-stage kidney disease, but does not usually result in overall clinical and biochemical improvement.
Enzyme replacement therapy (ERT) was approved for use in Fabry disease in Europe in 2001 and in the USA in 2003; the response to therapy is variable. ERT appears to be least effective in individuals with advanced disease. ERT reduces neuropathic pain, improves the detection threshold for cold and warm sensation in the hand and foot, and leads to improvement in sweating and heat tolerance, but not does not fully reverse peripheral nerve involvement in Fabry disease. The extent of renal impairment correlates with small fiber response to ERT; i.e. patients with more severe renal impairment have a more severe neuropathy. The deacylated product, trihexosylsphingosine, has been suggested as a useful biomarker following ERT for Fabry disease. Newborn screening in Fabry disease suggests a high frequency of under-diagnosis of later onset forms that could be treated effectively if diagnosed.
Mucopolysaccharidoses
The mucopolysaccharidoses ( Table 19.3 ) are lysosomal storage diseases due to deficiencies of specific lysosomal enzymes responsible for the degradation of heparan sulfate, dermatan sulfate, or keratan sulfate, singly or in combination. Lack of lysosomal enzymes activity specific to each disorder leads to tissue accumulation and urinary excretion of mucopolysaccharides.
Disease | Common Name | Relevant Metabolite | Metabolic Defect | Clinical Features |
---|---|---|---|---|
MPS I H | Hurler | Dermatan sulfate | α-L-Iduronidase | Severe physical and cognitive difficulties; early death |
Heparan sulfate | ||||
MPS I S | Scheie | Dermatan sulfate | α-L-Iduronidase | Severe physical difficulties; normal mentation and survival |
Heparan sulfate | ||||
MPS I H/S | Hurler-Scheie | Dermatan sulfate | α-L-Iduronidase | Intermediate |
Heparan sulfate | ||||
MPS II | Hunter | Dermatan sulfate | Iduronate-2-sulfatase | Similar to Hurler, somewhat milder |
Heparan sulfate | ||||
MPS III A | Sanfilippo A | Heparan sulfate | Heparan-N-sulfatase | Severe cognitive difficulties; mild physical changes |
MPS III B | Sanfilippo B | Heparan sulfate | N-Acetyl-α-glucosaminidase | Severe cognitive difficulties; mild physical changes |
MPS III C | Sanfilippo C | Heparan sulfate | Acetyl-CoA: α-glucosaminide | Severe cognitive difficulties; mild physical changes |
N-acetyltransferase | ||||
MPS III D | Sanfilippo D | Heparan sulfate | N-Acetyl-α-glucosamine-6-sulfatase | Severe cognitive difficulties; mild physical changes |
MPS IV A | Morquio A | Keratan sulfate | N-Acetylgalactosamine-6-sulfatase; galactose-6-sulfate sulfatase | Severe skeletal features; normal mentation |
Chondroitin 6-sulfate | ||||
MPS IV B | Morquio B | Keratan sulfate | β-Galactosidase | Milder skeletal features |
MPS VI | Maroteaux-Lamy | Dermatan sulfate | Arylsulfatase B | Severe skeletal features; normal mentation |
MPS VII | Sly | Dermatan sulfate | β-Glucuronidase | Severe skeletal features; moderate cognitive impairment |
Heparan sulfate | ||||
Chondroitin 4-, 6-sulfate |
The mucopolysaccharidoses have many common clinical features including hepatosplenomegaly, deposition of mucopolysaccharide in arterial smooth muscle with resultant coronary insufficiency and compromise of the aortic wall, and accumulation in heart valves producing both insufficiency and stenosis. Varying degrees of skeletal involvement (dysostosis multiplex) and joint contractures with limitation of motion occur in all but Morquio disease. Mixed or sensorineural deafness occurs in all except Sanfilippo disease. Corneal clouding occurs to varying degrees in these disorders, but may be absent or seen only by slit lamp in Hunter disease. Pigmentary retinopathy is occasionally seen in Scheie, Sanfilippo, and Hunter diseases.
The most frequent neurologic manifestation is psychomotor retardation. This is present, albeit with varying severity, in Hurler, Hunter, and Sanfilippo diseases, the result, most likely, of mucopolysaccharide and lipid deposition in brain cells. Thickening of the meninges secondary to mucopolysaccharide storage may result in hydrocephalus, particularly in Hurler and Hunter disease. Meningeal thickening in the cervical region may result in myelopathy secondary to spinal cord compression and nerve root compromise.
Peripheral neuropathy may develop from nerve entrapment as the result of mucopolysaccharide accumulation in adjacent connective tissue ( Table 19.2 ). Median nerve compression in the carpal tunnel is the most common neuropathic manifestation. Carpal tunnel compression has been noted prominently in Scheie (MPS I S), Hunter (MPS II), and Maroteaux-Lamy (MPS VI) diseases. Mucopolysaccharidoses are the most common cause of carpal tunnel syndrome in childhood, usually presenting with impaired fine motor function. One study found that 43 of 45 boys with MPS II had carpal tunnel syndrome; it was bilateral in all cases. The profound cognitive impairment in Scheie or Hunter disease may delay recognition of peripheral nerve symptoms until involvement is advanced. Surgical management is generally effective.
The original classification of the mucopolysaccharidoses as distinct disorders—Hurler, Hunter, Sanfilippo, Morquio, Scheie, Maroteaux-Lamy, and β-glucuronidase deficiency—derived from their variable clinical features. Subsequent biochemical and molecular studies indicated that Hurler and Scheie are allelic, caused by different mutations of the same gene. In contrast, Sanfilippo and Morquio diseases have multiple subtypes caused by mutations in different genes. Enzyme replacement therapy delivered by intravenous infusion has been approved in the USA for MPS types I, II, and VI; it ameliorates somatic manifestations, but does not influence nervous system disease. Several lines of evidence support early initiation of ERT in this population to minimize the consequences of irreversible tissue damage. Hematopoietic stem cell transplantation stabilizes cognitive decline and prolongs survival in MPS type I, but not in other MPS disorders; animal studies suggest that substrate reduction therapy and gene therapy are promising alternative strategies for MPS disorders with no, or currently inadequate, treatment.
Glycoproteinoses
The glycoproteinoses include fucosidosis, α- and β-mannosidosis, the sialidoses, and aspartylglucosaminuria, and resemble the other lysosomal hydrolase deficiency diseases in many respects ( Table 19.4 ). These glycoproteinoses are characterized by the inability to degrade the oligosaccharide portion of glycoproteins, resulting in the excretion of large quantities of oligosaccharides in urine. Significant clinical heterogeneity exists within the specific entities, each of which is inherited in an autosomal recessive fashion. Prenatal diagnosis is possible, but specific treatment is lacking.
Disease | Relevant Metabolite | Metabolic Defect | Clinical Features |
---|---|---|---|
Fucosidosis | Fucose-containing glycoproteins and oligosaccharides | α-fucosidase | Coarse facies |
Dysostosis multiplex | |||
Hearing loss | |||
Psychomotor decline | |||
α -Mannosidosis | Mannose-containing glycoproteins and oligosaccharides | α-mannosidase | Coarse facies |
Dysostosis multiplex | |||
Hearing loss | |||
Psychomotor decline | |||
β-Mannosidosis | Mannosyl-β-N-acetyl-glucosamine | β-mannosidase | Recurrent infections |
Psychomotor decline | |||
Peripheral neuropathy in delayed-onset forms | |||
Sialidoses | |||
Type I | Sialic acid-containing oligosaccharides | Sialidase | Progressive myoclonus |
Visual loss | |||
Cherry-red maculae | |||
Ataxia | |||
Type II | Sialic-acid containing oligosaccharides | Sialidase | Coarse facies |
Contractures of major joints | |||
Mild dysostosis multiplex | |||
Cherry-red maculae | |||
Psychomotor decline | |||
Peripheral neuropathy | |||
Sialic acid storage diseases | Sialic acid | Sialic acid lysosomal transport | Coarse facies |
Dysostosis multiplex | |||
Growth retardation | |||
Psychomotor decline | |||
Galactosialidosis | Sialic-acid containing oligosaccharides | Protective protein/Cathepsin A | Similar to type II sialidosis |
Aspartylglucosaminuria | Aspartylglucosamine | Aspartylglucosaminidase | Coarse facies |
Mild dysostosis multiplex | |||
Cognitive impairment | |||
N-acetylgalactos-aminidase deficiency (Schindler disease) | Sialic acid-containing and asialoglycoproteins and oligosaccharides | α-N-acetylgalactosaminidase | Psychomotor decline |
Myoclonic epilepsy | |||
Cortical blindness |
Fucosidosis, mannosidosis, type II sialidosis, and infantile sialic acid storage disease have many features in common with the mucopolysaccharidoses. As such, entrapment neuropathies may be noted. In addition, inclusions have been noted in peripheral nerves and sympathetic neurons in type II sialidosis ( Table 19.2 ).
Cherry Red Spot Myoclonus Syndrome (Sialidosis I)
Debilitating myoclonus occurs in the second or third decade in cherry red spot myoclonus syndrome and is associated with nonpigmentary macular degeneration secondary to lysosomal neuraminidase deficiency. Sural nerve biopsy may demonstrate segmental demyelination with Schwann cells containing indeterminate abnormal storage material within membrane bound vacuoles.
Schindler Disease
Clinical Characteristics
Peripheral nerve involvement in α-N-acetylgalactosaminidase deficiency (Schindler disease) is much more marked than in the other glycoproteinoses. The hallmark of Schindler disease is a central and peripheral axonopathy.
Schindler disease is a very rare disorder, described in only a small number of individuals. An acute form has its onset from 9 to 15 months as an exaggerated startle response after normal early infancy. Psychomotor deterioration is rapid, leading to marked spasticity, cortical blindness, and myoclonic epilepsy. The EEG shows background slowing and multifocal spike and spike and wave activity. The VER, ABR, and SSEP are reduced, whereas NCV and ERG are normal.
A chronic form features mild cognitive impairment and slow progression with dilated, tortuous retinal vessels and cutaneous angiokeratoma, most prominent over the torso.
Pathology
An axonal neuropathy is prominent, resembling that of infantile neuroaxonal dystrophy. Axonal spheroids are present in peripheral nerve axons and in axons ranging from cerebral cortex to the myenteric plexus. Electron microscopy revealed membranous cytoplasmic bodies in these tissues. α-N-acetylgalactosaminidase B deficiency, which establishes the diagnosis, may be measured in leucocytes or cultured skin fibroblasts. This enzyme is identical to its isomer, α-galactosidase A, the deficient enzyme in Fabry disease.
The only three patients described with Schindler disease and neuroaxonal dystrophy were all related; this observation, and the wide range of phenotypes associated with α-N-acetylgalactosaminidase B deficiency, has led some authors to propose that children with enzyme deficiency and the neurological phenotype also have mutations in the calcium-independent phospholipase A 2 gene, PLA2G6 , which is the cause of infantile neuroaxonal dystrophy.
Peroxisomal Storage Diseases
Peripheral neuropathy has been described mainly in three peroxisomal storage diseases, Refsum disease, adrenomyeloneuropathy, and hyperoxaluria ( Table 19.2 ). Refsum disease is quite responsive to dietary therapy when early diagnosis is established. Hyperoxaluria may be treated with combined liver-renal transplantation.
Phytanic Acid Lipidosis (Refsum Disease; Heredopathia Atactica Polyneuritiformis)
Clinical Characteristics
Refsum disease, or heredopathia atactica polyneuritiformis, was first described by Sigvald Refsum in Norway. The primary clinical features are the triad of retinitis pigmentosa, chronic polyneuropathy, and cerebellar ataxia. Most patients have additional clinical features such as sensorineural hearing loss, anosmia, skin changes, skeletal malformations, and cardiac abnormalities. Clinical manifestations are observed before age 10 years in 43% and before age 20 in 75% of patients. Refsum disease must be distinguished clearly from infantile Refsum disease, which is a disorder of peroxisome biogenesis, presenting in infancy with a wide range of clinical manifestations. Refsum disease is restricted to those individuals with clinical manifestations of heredopathia atactica polyneuritiformis and the biochemical and gene defects described below.
Peripheral neuropathy is a prominent part of the symptomatology, but may be preceded for several years by retinal degeneration. The neuropathy is symmetric and usually affects the distal parts of the lower limbs with muscle atrophy, weakness, and foot drop. The upper extremities are usually also involved. Over the course of the illness, muscle weakness can become widespread and disabling. The trunk musculature is also commonly involved. Sensory disturbances are frequent, usually involving vibration and position sense, but cutaneous hypesthesia of the glove and stocking type may also occur. The peripheral nerves (ulnar, radial, peroneal, and great auricular) may be palpably enlarged and firm. Motor and sensory conduction velocities are markedly reduced. The level of cerebrospinal fluid protein is increased.
Pigmentary retinal degeneration is often the first clinical manifestation of Refsum disease, night blindness often being the only clinical manifestation in children and young adults. Electroretinograms (ERG) demonstrate a reduction or complete absence of rod and cone responses even at that time. Concentric visual field constriction gradually develops, so that only tubular vision remains. It is important to consider Refsum disease in all patients with retinitis pigmentosa. Other ocular abnormalities that may be seen in Refsum disease include cataracts, optic atrophy, and vitreous opacities.
Other clinical manifestations include hyposmia or anosmia , which is an early clinical sign. Progressive loss of hearing is often an early symptom and may eventually become almost complete. Cerebellar ataxia was originally considered part of the principal clinical triad of Refsum disease, but reevaluation by Skjeldal et al. concluded that the unsteadiness of gait is attributable mainly to the neuropathy, with clinically detectable signs of cerebellar involvement found in less than a third of individuals. Cardiac abnormalities are common, and include cardiac enlargement, heart failure, conduction disturbances, and electrocardiographic changes. Cardiac failure is the most common cause of sudden death in Refsum disease. Dry skin and ichthyosis , related to phytanic acid accumulation, occur to a variable extent.
Pathology
Pathologically, lipid storage is evident in the leptomeninges, the ependyma, the choroid epithelium, the liver, and the kidneys. The peripheral nerves are enlarged by a hypertrophic neuropathy with onion bulbs and segmental demyelination. Electron microscopy shows the frequent presence of lipid granules in the Schwann cell cytoplasm. The central nervous system may show diffuse myelin destruction around the inferior olivary nuclei and in the brain stem and dentate nucleus. The retina shows an almost complete loss of photoreceptors, thinning of the inner nuclear layer, and reduction in the number of ganglion cells.
Biochemistry
The principal biochemical abnormality is the accumulation of phytanic acid, a branched chain fatty acid, 3,7,11,15-tetramethylhexadecanoic acid, which is toxic to cells and is exclusively of dietary origin.
Phytanic acid levels are increased in the plasma. Due to the presence of the α-methyl group, phytanic acid cannot be degraded by the beta-oxidation mechanism used for most fatty acids, and an alpha oxidation reaction that results in the oxidation of phytanic acid to pristanic acid (2,6,10,14-tetramethylpentadecanoic acid) is the first step in phytanic acid degradation.
The enzymatic defect in Refsum disease, phytanoyl-CoA hydroxylase deficiency, has been localized to the peroxisome. Phytanic acid accumulates in several peroxisomal disorders other than Refsum disease, including rhizomelic chondrodysplasia punctata and deficiency of peroxisomal bifunctional enzyme and the disorders of peroxisome biogenesis, infantile Refsum disease, neonatal adrenoleukodystrophy, and Zellweger syndrome. Determination of the ratio of phytanic to pristanic acid and the measurement of other peroxisomal functions aid the distinction between Refsum disease and these other disorders. In Refsum disease, biochemical defects are usually confined to phytanic acid, except for what appears to be an allelic disorder in which pipecolic acid levels are also increased. Plasma phytanic acid levels are elevated, while the levels of pristanic acid, which is below the metabolic block, are low or undetectable.
Molecular Defect
Refsum disease is inherited as an autosomal recessive trait, the gene encoding phytanoyl-CoA hydroxylase, PHYH , mapping to 10p. A subgroup of affected individuals (about 10% of patients with clinical and biochemical features of Refsum disease), do not have mutations in PHYH , but rather in PEX7 , which encodes the peroxin 7 receptor protein, an important component of the peroxisomal protein import system. A disorder with features mimicking Refsum disease, but with normal phytanic and pristanic acid levels, has been reported in a consanguineous Norwegian kindred and linked to chromosome 20.
Management
Dietary therapy is based on the knowledge that phytanic acid, which is derived exclusively from dietary intake, is the toxic agent in this disease. Reduction of dietary phytanic acid intake lowers plasma levels of phytanic acid over some months. This goal can be accomplished by restricting the intake of products from ruminant animals and fish. The contribution of phytanic acid from vegetables is negligible. Brown et al. have measured the phytanic acid content of 151 foods and provide practical guidelines on how to provide a nutritionally adequate and acceptable diet. Dietary therapy improves peripheral nerve function and appears to stabilize central nervous system deficits. Early, presymptomatic diagnosis combined with prompt institution of dietary therapy may prevent many disease sequelae. Very high plasma levels of phytanic acid may produce toxic and life-threatening symptoms, including quadriparesis and cardiac dysfunction, necessitating the use of plasmapheresis.
Peripheral Neuropathy in Disorders of Peroxisome Biogenesis
Peripheral neuropathy may also be a presenting symptom in disorders of peroxisome biogenesis ( Table 19.2 ). Baumgartner et al. (case 26 in ) described a woman who developed normally until 11 years of age and then developed ataxia and gait difficulties. EMG and nerve conduction studies displayed axonal sensory polyneuropathy. Slight elevation of plasma phytanic acid levels was found and Refsum disease was diagnosed. However, at age 21 years, her condition worsened and she developed dementia and evidence of severe and progressive central nervous system involvement. Subsequent studies revealed a disorder of peroxisome biogenesis.
X-linked Adrenoleukodystrophy
X-linked adrenoleukodystrophy (X-ALD) is a peroxisomal disorder that mainly affects the central nervous system white matter and axons, the adrenal cortex, and the testis, with an incidence of 1:17,000.
Phenotypic expression varies widely, ranging from the rapidly progressive childhood cerebral form that often leads to total disability and death by 10 years of age, to the somewhat milder adult form that presents in young adulthood with a paraparesis that progresses over decades and is referred to as adrenomyeloneuropathy (AMN). Some males with the same gene defect have adrenocortical insufficiency (Addison disease) without apparent nervous system involvement. At least 70% of males with X-ALD have adrenocortical insufficiency. Approximately 50% of female heterozygotes develop a milder AMN-like syndrome in middle age or later. This form is often unrecognized.
The principal biochemical abnormality is the accumulation of saturated very long chain fatty acids (VLCFA) in tissues and plasma. Measurement of plasma VLCFA is the most common diagnostic assay. The defective gene maps to Xq28, encoding a peroxisomal membrane protein referred to as ALDP or ABCD 1. More than 690 nonrecurrent mutations have been identified and are accessible at the X-ALD database website ( www.x-ald.nl ).
The childhood cerebral and the AMN phenotypes co-occur, often within the same family. The rapidly progressive cerebral phenotypes are associated with an inflammatory demyelinating response that may be mediated by cytokines and autoimmune mechanisms. AMN, in contrast, presents as a noninflammatory distal axonopathy that involves the spinal cord most severely, with the gracile tracts involved most in the cervical cord, and the corticospinal tracts in the lumbar region. Some degree of peripheral nerve involvement occurs in AMN and in heterozygous women. It is generally not a feature in childhood X-ALD.
Clinical Characteristics of AMN
The typical AMN patient presents with stiffness or clumsiness in the legs in the third or fourth decade, with inexorable progression over the next several decades to severe spastic paraparesis. Voiding abnormalities are seen early in the disease, as are impaired vibration and position sense with abnormal somatosensory and brainstem auditory evoked responses.
Several large studies of peripheral nerve function in AMN have been reported, describing abnormalities more commonly in the legs than in the arms. Almost 60% of X-ALD heterozygotes develop peripheral neuropathy. Abnormalities represent either a mixture of axonal loss and multifocal demyelination or primary axonopathy; 26% of individuals partially fulfilled the neurophysiological criteria of primary demyelination. Restuccia et al. demonstrated abnormalities of motor and sensory evoked responses in 12 of 19 heterozygotes. Approximately 30% of AMN patients develop cerebral involvement. When this occurs, the disorder may progress rapidly.
Pathology
The most severe pathological changes in AMN affect the spinal cord, are bilateral, and are usually symmetrical. Ascending tract (gracile and dorsal spinocerebellar) degeneration is worse in the upper cord (further from the cell of origin), and descending tract degeneration (pyramidal) is worse in the lower cord (furthest from the cell of origin). Axonal loss is equal to or greater than myelin loss, and controversy exists about the degree of peripheral nerve involvement as peripheral nerve lesions are variable and mild compared with the myelopathy. Spinal nerve roots and ganglia, sciatic, popliteal, and ulnar nerves have been reported as unremarkable, whereas sural and peroneal nerves reveal loss of large and small diameter myelinated fibers.
Management
It is essential that adrenocortical function be monitored in all male X-ALD patients and treated by replacement therapy in order to prevent death or morbidity from adrenal crisis. Boys and adolescents in the early stages of cerebral involvement can benefit from bone marrow transplantation, which may stabilize cerebral lesion long-term, but which is a high-risk procedure. The favorable effect of BMT in these patients appears to be on the brain inflammatory response. It is not known whether BMT has a favorable effect on the noninflammatory myelopathy in AMN. Cyclophosphamide therapy is ineffective. Dietary therapy with a 4:1 mixture of glyceryl trioleate and glyceryl trierucate can normalize the plasma VLCFA levels in patients with X-ALD, but favorable clinical effects have not been demonstrated.
Hyperoxaluria
Hyperoxaluria may be primary, due to an inherited metabolic disease, or secondary to environmental factors including diet, drugs, or coincident renal disease.
Hyperoxaluria resulting from an inherited metabolic defect is rather rare, arising from deficiency of the liver peroxisomal enzyme, alanine-glyoxylate aminotransferase (AGT), or the cytosolic enzyme, D-glycerate dehydrogenase/glyoxylate reductase (DGDH/GR). As such, these disorders are classified as primary hyperoxaluria type 1 (PH1) due to AGT deficiency, type 2 (PH2) due to DGDH/GR deficiency, and type 3 (PH3) associated with defects in the liver-specific mitochondrial enzyme 4-hydroxy-2-oxoglutarate aldolase (HOGA). Only PH1 has systemic features, including peripheral neuropathy, due to oxalate storage in multiple tissues, whereas PH2 is restricted to renal involvement, and PH3 has the mildest phenotype.
PH1 has its onset before age 5 in most instances, presenting with hematuria or renal colic secondary to urolithiasis. Ultimately, oxalate (calcium oxalate) accumulation leads to chronic renal disease. Oxalate accumulation is widespread including bone, eye, blood vessels, brain, and peripheral nerves.
Peripheral nerve involvement includes evidence of both axonal degeneration and segmental demyelination. Although painful paresthesias and muscle weakness have been described, clinical manifestations of peripheral neuropathy tend to be minor.
As effective therapy for PH1 is now feasible, appropriate diagnostic methodologies should be employed to distinguish PH1 from PH2 and PH3, as well as from secondary hyperoxaluria. AGT is a liver-specific peroxisomal enzyme. In the past, definitive diagnosis required liver biopsy. The distinction from PH2 was made by measuring DGDH activity in leucocytes. Now, direct molecular analysis of the genes responsible for PH permits rapid, noninvasive diagnosis.
Renal transplantation alone does not prevent ongoing oxalate accumulation, as the deficient enzyme, AGT, is present only in liver peroxisomes. In order to reverse systemic complications, and thus provide effective therapy for PH1, combined kidney-liver transplantation is required. Vitamin B6 therapy is effective in lowering oxalate levels in patients with specific mutations causing PH1.
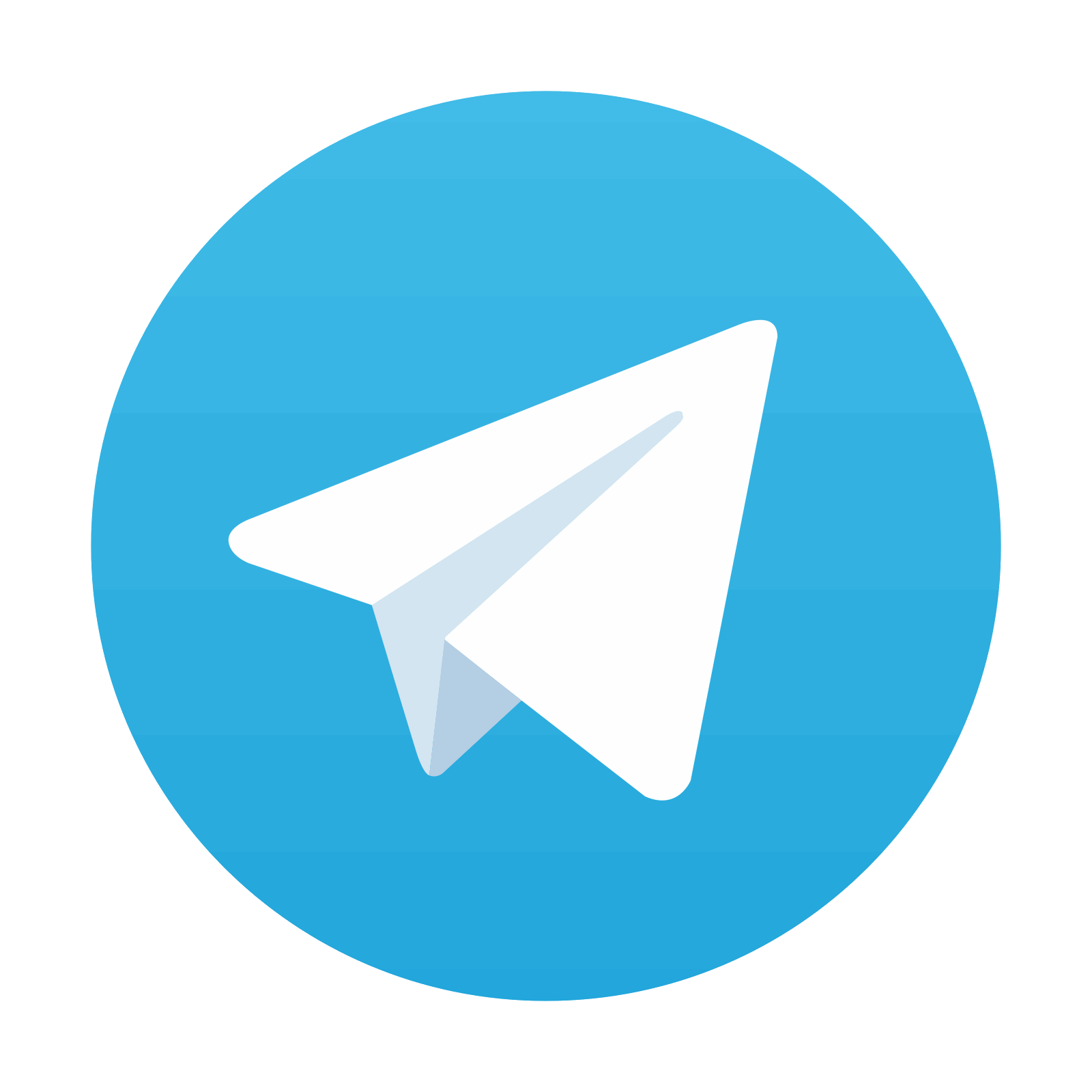
Stay updated, free articles. Join our Telegram channel

Full access? Get Clinical Tree
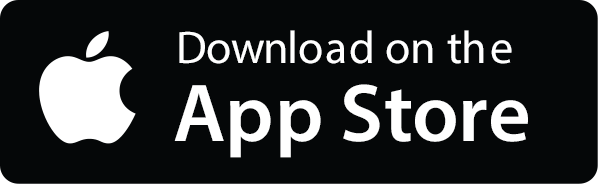
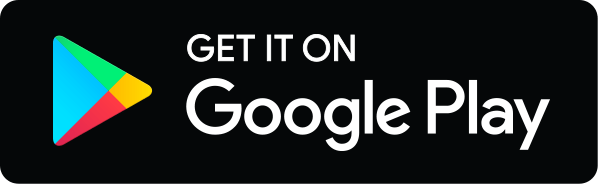