Abstract
Intrathecal therapy is a vital component in the treatment of moderate to severe refractory cancer or noncancer pain. From humble beginnings, the knowledge and clinical experience regarding engagement with targeted drug delivery continue to expand. It is moving away from salvage therapy for high-dose opioid failure to a renewed focus on earlier treatment engagement based on disease indications and greater appreciation for patient selection criteria and device placement optimization. This chapter focuses on the pharmacology of the intrathecal space and its role in improving patient safety and sustainable efficacious outcomes.
Keywords
Cerebrospinal fluid, Intrathecal therapy, Pharmacokinetics, Pharmacology, Targeted drug delivery
Introduction
The history of intrathecal (IT) implantable drug delivery system (IDDS) therapy for chronic cancer and noncancer pain is rooted in experimentation with local anesthetics administered into the cerebrospinal fluid (CSF). For a thorough historical review see Chapter 60 .
Since the first implantable pumps were approved by the United States Food and Drug Administration (FDA), experience with more than 300,000 implanted pumps has established IDDS as a safe and effective route of administration for medications used to treat intractable spasticity and cancer and noncancer pain ( ). IDDSs were used when conservative medical management and interventional and surgical therapies failed. IT delivery was also considered when there were intolerable side-effects to oral opioids, such as sedation, constipation, and urinary retention ( ).
As the need for IDDS therapy grew and new innovations arrived, a number of issues became apparent. These included which drug or combination of drugs would be most effective for treatment of neuropathic, nociceptive, or mixed-pain disease. Other issues included the concentration of medicines to use, catheter placement, and infusion strategies such as continuous, bolus, or patient-activated bolus. This called for more research on the dynamic forces present in the IT space. Research began to elucidate the solutions to these complexities ( ). Based on new data, guideline statements were created to improve patient safety by reducing variability in catheter placement, determining which medications should be used for specific pain types, and selecting patients who would benefit most from IT therapy ( ).
A subsequent comprehensive review resulted in consensus guidelines for the selection of patients with noncancer pain for IDDS, and concluded that IT therapies were effective, cost neutral, and appropriate ( ). While device-related complications such as catheter kinking or transection can occur, the main therapy-related safety issues are respiratory depression, inflammatory mass formation, dosing errors, and pump refilling errors where the medication is mistakenly delivered into the tissue surrounding the pump rather than into the pump itself ( ). The formation of the Polyanalgesic Consensus Conference (PACC) addressed these safety concerns by analyzing all available high-quality research as well as provider experience to identify best practices, ensure safety, and optimize therapy ( ).
A serious risk with IT therapy is the formation of a sterile, expanding inflammatory mass known as a granuloma at the tip of the spinal catheter. Development of a granuloma is one of the most serious risks of IT therapy because of the potential for temporary or permanent neurological injury. The first report of granuloma formation associated with IT therapy was published in 1991, a decade after implantable pumps were introduced ( ). A retrospective longitudinal study that included 56 patients on long-term IT therapy with morphine or diamorphine found granuloma development reported in 4 patients ( ). In one study granuloma formulation was found to have a significant positive correlation with opioid dose and yearly increase in opioid dose, but not with flow rate or opioid concentration ( ).
Indications
Patients with chronic pain can have nociceptive, neuropathic, or mixed-pain states. Neuropathic pain is typically described as burning, gnawing, and lancinating, whereas nociceptive pain is commonly described as aching, mechanical, and sharp. Conservative therapies include ultrasound or fluoroscopically guided injections, oral medications such as gabapentin or tricyclic antidepressants, systemic medication trials with intravenous lidocaine or ketamine, and careful use of opioids. Chronic opioid therapy has not been found beneficial for long-term treatment of neuropathic pain, and in one study was found actually to worsen pain of HIV peripheral neuropathy ( ).
Failure of patients to respond to these conservative treatments provokes consideration of advanced therapies such as spinal cord stimulation (SCS) and IT therapy. For many chronic pain syndromes, SCS is used before IT therapy as there is compelling data suggesting SCS is the safer option to begin treatment ( ). Patient populations likely to benefit from IT therapy include those with failed back surgery syndrome, vertebral compression fractures, nonoperative spondylolisthesis, and radiculopathy ( ). These advanced therapies can also treat visceral, pelvic, and abdominal pain in both cancer and noncancer patients ( ). To be an IT therapy candidate, the patient must meet both the disease indications and patient selection criteria to optimize outcomes. Patient selection criteria include preoperative stabilization of medical comorbidities, capacity to understand the therapy, ability to be present for medication refills and scheduled visits, and stabilization of any psychiatric diagnoses such as depression, anxiety, or personality disorders ( ).
Pharmacokinetics and Pharmacodynamics
After delivery into the CSF, active molecules must diffuse across the pia mater and white matter of the spinal cord to reach target receptors in the gray matter or nerve rootlets ( ). The pia mater, being only a single layer of cells without intercellular junctions, is very permeable to IT medications. For medications to enter the parenchyma of the spinal cord, fluid pathways paralleling the intraparenchymal vasculature can be used ( ). The spinal cord white matter consists of myelinated axons, making it hydrophobic, whereas the gray matter consists of cell bodies, making it hydrophilic ( ). Continuous IT infusion results in stable CSF drug concentrations, establishing a gradient that drives drug diffusion into neural tissue ( ).
A number of physicochemical factors intrinsic to the chosen IT medication determine the level of drug uptake. Of these, lipid solubility and molecular weight are the most important. Hydrophilic medications, such as morphine and hydromorphone, have a pharmacokinetic advantage in the CSF over hydrophobic agents, such as fentanyl and bupivacaine. In the CSF, hydrophilic molecules have longer half-lives because hydrophobic agents enter the vasculature and are readily cleared out of the subarachnoid space. Hydrophilic medications have smaller volumes of distribution, resulting in deeper cord penetration and more rostral spread ( ). Hydrophobic medications have the advantage of limited spread. This is useful when precise, targeted delivery is desired ( ).
Opioids
Three types of G-protein-linked opioid receptors can be distinguished: mu, delta, and kappa. Presynaptic opioid receptor activation inhibits the release of the neurotransmitters substance-P and calcitonin gene-related peptide. This is done by downstream inhibition of N-type voltage-dependent calcium channels, which reduces calcium influx and thereby minimizes neurotransmitter release ( ). Postsynaptically, activation of opioid receptors leads to inhibition of adenylate cyclase and the opening of potassium channels ( ). Open potassium channels hyperpolarize cells, rendering the postsynaptic second-order neurons less responsive.
The pharmacokinetics of IT opioid administration is very complex, especially with low-volume infusions. The opioid receptors are concentrated in lamina II (substantia gelatinosa) of the dorsal horn (DH). Early work in rabbit models showed that hydrophilic opioids administered into the CSF diffused further into the gray matter than hydrophobic opioids ( ). Later, work using pig models showed hydrophobic opioids readily diffused across the dura into the epidural fat, thereby failing to activate the opiate receptors contained within the gray matter ( ). These findings demonstrated that the superior bioavailability of hydrophilic opioids is due to their longer presence in the CSF, allowing for greater penetration and concentration into the hydrophilic DH gray matter ( ). Morphine and hydromorphone are cleared from the CSF by simple diffusion into the plasma; once in the plasma they are metabolized in the liver and renally excreted.
Common side-effects of IT opioids include respiratory depression, development of tolerance, hyperalgesia, urinary retention, constipation, pruritus, peripheral edema, and hypogonadism ( ). Respiratory depression happens with rostral spread of opioids, and is more likely with hydrophilic opioids such as morphine; it most commonly results from too-rapid titration and accidental overdosing ( ). Thus strict monitoring of a patient’s respirations during trialing and ensuring proper infusion concentrations are vital. Tolerance to IT opioids is most common in patients younger than 50 years old ( ), and leads to dose escalation. Opioid tolerance can be attenuated by using a combination of IT opioid and bupivacaine ( ). Lowering patients’ oral opioid dose or weaning them off of opioids prior to trialing and implant has also been shown to limit dose escalation ( ).
Local Anesthetics
Local anesthetics act by blocking the voltage-gated Na + channels in neuronal cell membranes. They preferentially act on the fila radicularia, given the large surface-to-volume ratio of these nerve rootlets relative to the spinal cord ( ). Local anesthetics have been shown to act synergistically with opioids when administered intrathecally in cancer pain studies ( ). A retrospective review showed that coadministration of IT bupivacaine with an opioid decreased the rate of opioid dose escalation by 65% over the first year in noncancer pain patients ( ). However, a double-blind cross-over multicenter randomized controlled trial (RCT) showed that the combination of opioids and bupivacaine had no additional analgesic efficacy ( ). Nonetheless, bupivacaine is often coadministered to reduce opioid dose escalation, thus minimizing opioid side-effects.
The most concerning complication associated with IT local anesthetic use is neurotoxicity; other complications include extremity weakness, paresthesias, hypotension, and urinary retention ( ). Bupivacaine is the only local anesthetic included in the PACC treatment algorithms, and is considered a first line in combination with morphine for neuropathic pain and a second-line agent in combination with morphine, hydromorphone, or fentanyl for nociceptive pain ( ). Safety studies show no neurologic sequelae or toxicity at IT doses of up to 30 mg/day ( ).
Ziconotide
Ziconotide is a peptide synthesized from the venom of Conus magus , a marine snail. It reversibly binds N-type voltage-gated calcium channels in the central nervous system (CNS) and prevents the release of glutamate, calcitonin gene-related peptide, and substance-P in the DH of the spinal cord ( ). Ziconotide possesses linear pharmacokinetics within the CSF and is cleared by diffusion into the systemic circulation, where it is metabolized by peptidases and proteases ( ). Its safety and efficacy have been evaluated in several double-blinded placebo-controlled RCTs in humans with malignant and nonmalignant pain ( ). These trials showed a significant, albeit small, decrease in Visual Analog Scale of Pain Intensity (VASPI) scores when compared with placebo.
Respiratory depression, granuloma formation, and development of tolerance to the drug have not been found to occur. Intolerable effects are primarily neurologic and psychologic ( ). The most common adverse neurologic reactions associated with ziconotide are dizziness, nystagmus, confusion, memory impairment, and nausea. Psychiatric side-effects, such as increased risk of suicide in patients with a history of depression, happen with a fast titration rate and are more common in elderly patients. Discontinuation of ziconotide therapy due to intolerable side-effects is generally low, but has been reported to be as high as 61% ( ). Creatinine kinase levels also need to be checked at baseline and even intermittently during therapy with ziconotide. Clinical trials showed that creatinine kinase levels can rise to two or three times the upper limit of normal, suggesting rhabdomyolysis ( ).
Clonidine
This medication is an alpha-2 receptor agonist; when delivered intrathecally it is thought to provide pain relief by inhibiting the activation of NF-κB and p38 in glial cells. This causes inhibition of several proinflammatory cytokines that have been associated with neuropathic pain states ( ). Like opioids, when delivered systemically clonidine reduces presynaptic calcium entry and increases postsynaptic potassium influx, hyperpolarizing the neuron. Clonidine is typically combined with local anesthetics and morphine due to their synergistic actions for pain relief ( ). IT clonidine has been shown to reduce the risk of morphine tolerance ( ). Serious side-effects include sedation, bradycardia, and hypotension. Sudden discontinuation of long-term IT therapy may lead to rebound hypertension, panic attacks, and psychotic behavior ( ).
CSF and Catheter Placement
Understanding of the dynamics of drug distribution in the CSF has been a large focus of investigation. Initial concepts of CSF flow in the IT space were of a cephalad-to-caudad bulk flow along the posterior surface of the spinal cord ( ). If true, this suggests CSF motion would be expected to move IT drugs considerable distances. Animal studies have found that this portrayal of CSF movement is incorrect ( ). Numerous human studies using magnetic resonance imaging techniques have shown that CSF oscillates back and forth along the rostrocaudal axis ( ). This motion is driven by cyclic expansion and contraction of the cerebrospinal vasculature during cardiac systole and diastole. The magnitude of the motion is greatest in the upper cervical regions and decreases with more caudal distance from the foramen magnum. This oscillation of the CSF becomes negligible at the level of the cauda equina ( ).
Other research has shown that net CSF motion is limited for multiple reasons, such as CSF propulsion in opposite directions during each cardiac cycle and the lack of circumferential CSF motion ( ). This finding explains why patients can have markedly different rostrocaudal CSF concentration gradients for many molecules. These pharmacokinetic properties of medications in the CSF have led to a paradigm shift regarding the importance of catheter position. Most practitioners determine catheter placement based on dermatomal location of pain or SCS paresthesia mapping ( ).
All drugs delivered by IDDS are poorly dissipated in the CSF, due to a combination of factors including the low kinetic energy provided by implantable pump delivery, a limited liquid/liquid diffusion coefficient, and the absence of meaningful CSF bulk flow. Fine structures within the subarachnoid space, including nerve rootlets, venous plexuses, and epidural fat, create barriers for bidirectional flow, resulting in complex local mixing ( ). In a small-volume computational flow-dynamics model, speed of drug transport and mixing are strongly affected by the frequency and volume of CSF pulsations ( ). For a more detailed discussion of CSF dynamics see Chapter 67 .
This suggests that the location of the infusion catheter tip relative to the targeted spinal cord segment is critical, given the limited distribution of the delivered medication ( ). This is exemplified in cases where patients had marked improvement of spasticity and pain after injection of baclofen or morphine during their trial, yet found limited or no relief after the implant. Many of these patients can be rescued by repositioning the catheter tip ( ). Attempting to make up for poor catheter position by increasing the flow rate seemed like a viable option, so the idea was tested in chronic regional pain syndrome patients by van der Plas et al. When the daily dose of baclofen was maintained and a fourfold increase in flow rate was implemented, side-effects increased but there was no positive effect on dystonia or pain ( ). Another study examined increased flow rates in chronic pain patients and found that a fourfold increase in flow rate, after an established constant daily dose, did not result in improved pain scores and was associated with a significant decrease in quality of life (QOL) scores ( ).
Agent Selection
Morphine
Morphine and other opiates used in IT therapy underwent preclinical safety examination in animals, as measured by the neurotoxicity standardized assessments. In chronically catheterized large-animal models the data shows that continuous infusions of morphine, hydromorphone, methadone, or fentanyl for at least 28 days causes no spinal tissue damage ( ). Morphine monotherapy efficacy and clinical data continue to support its use as a first-line therapy. Results from several long-term studies support the efficacy of IT morphine for treating patients with both cancer and noncancer pain ( ). One retrospective study examined patients with chronic malignant pain on long-term IT opioid therapy, including morphine, hydromorphone, and sufentanil ( ). They noted that the VASPI score significantly decreased from baseline up to the time of first refill. These scores remained stable and significantly lower than baseline scores for 3 years.
Recent results from several long-term studies support the efficacy of IT morphine. In a prospective open-label study of IT morphine infusion, 110 patients with chronic pain were implanted and followed for 1 year ( ). Pain relief was noted within 1 month and was sustained for the 12-month period. Another open-label study examined patients with intractable pain due to chronic pancreatitis. These results showed a reduction in pain scores from an average of 8.3 to an average of 0.75, sustained for 29 months ( ).
For patients with vertebral fractures due to osteoporosis who do not respond to oral opiates, an open-label study of IT morphine was conducted ( ). The mean VASPI scores decreased significantly from 8.7 cm before IT therapy to 1.9 cm after 1 year. They also saw improvements in QOL, ambulation, and perception of health status. Interestingly, a retrospective study designed to identify characteristics of patients likely to benefit from IT morphine therapy found a greater than 50% decrease in pain in 73% of patients ( ). The study included patients with multiple types of pain, including cancer-related, nociceptive, and neuropathic pain. No differences in responder rates were noted regardless of pain type, patient age, or morphine dosage.
Expert consensus recommends morphine as a first-line agent for neuropathic and nociceptive pain at starting doses of 0.1–0.5 mg/day, 20 mg/mL maximum concentration, and a 15 mg/day maximum dose ( ).
Ziconotide
Ziconotide is the only nonopioid FDA-approved intrathecal option for the treatment of chronic refractory pain ( ). Three RCTs suggest efficacy, and another open-label multicenter study demonstrates safety ( ). Staats et al. designed an RCT using 111 patients with refractory pain due to cancer or AIDS. There was a statistically significant improvement in pain and reduced opioid requirements in the ziconotide group when compared with patients receiving placebo. A high number of adverse events (AEs) (30.6%), including dizziness, somnolence, nausea, and nystagmus, was seen in the ziconotide group. The study had several design limitations, including a limited 2-week exposure to ziconotide as well as variability in management of the IT infusions and the washout period prior to the RCT ( ).
Wallace et al. repeated this study design in patients with severe chronic nonmalignant pain. Ziconotide-treated patients had a mean improvement in VASPI scores of 31.2%, compared with 6.0% improvement in the placebo group. Unlike Staats et al., there was no decrease in the concomitant use of oral pain medications. Twenty-four ziconotide-treated patients and none of the patients receiving placebo discontinued the study at the initial titration due to drug side-effects ( ).
Lastly, Rauck et al. performed a double-blind RCT of IT ziconotide in adults with severe chronic pain. The authors reported a 14.7% improvement in pain from baseline in the ziconotide group compared to a 7.2% improvement in the placebo group after 3 weeks of treatment. There was no difference in systemic opioid use between groups. AEs were reported in both the placebo and ziconotide groups, with CNS AEs being reported more frequently using ziconotide ( ).
Ziconotide has also been studied in combination therapy. In a phase 2 open-label study the addition of IT ziconotide was examined in 26 patients with suboptimal pain relief on IT morphine therapy ( ). Ziconotide therapy began at a dosage of 0.6 mcg/day and was titrated to a maximum of 7.2 mcg/day. Reduction in VASPI scores was noted after 2 weeks of combination therapy, with a 14.5% mean VASPI score improvement by week 5. Notably, use of systemic opioids was reduced in these patients. A retrospective observational study of severe chronic pain of noncancer origin included 16 patients who had inadequate analgesia with IT opioid therapy and had ziconotide added to their IT regimen ( ). Ziconotide therapy began at a dosage of 0.5 mcg/day, and was titrated to a mean dosage of 2.64 mcg/day over the course of 12 weeks. At the end of this titration period, 20% of patients had a four-point decrease in their VASPI scores and 20% reported increased functional capacity.
Fentanyl
Fentanyl is an anilinopiperidine analog with limited rostral spread relative to morphine. With limited CSF distribution, precise catheter placement is required. The availability of fentanyl as a sterile, preservative-free injectable solution has led to its increased IT use for the management of refractory chronic pain, as reported in physician surveys ( ). Although animal studies using fentanyl for a limited duration have demonstrated efficacy, high-quality clinical evidence for its effectiveness in treating neuropathic and nociceptive pain is lacking ( ). IT-administered fentanyl rarely causes drug tolerance or the formation of granulomas. Studies examining neurotoxic effects of fentanyl showed no neural tissue damage at concentrations of 5000 mcg/mL ( ). The 2012 PACC group suggests maximum concentrations of 10 mg/mL for fentanyl; the majority of 2012 PACC members agreed that in most clinical settings patients will not benefit from daily doses higher than 1000 mcg of fentanyl ( ).
Hydromorphone
Hydromorphone is a hydrophilic semisynthetic opioid, similar in structure to morphine but with greater potency. Hydromorphone is highly soluble in a compounded mixture up to 80 mg/mL. In a retrospective review of 24 patients with refractory chronic nonmalignant pain, 8 patients began IT hydromorphone at the time of pump placement and 16 changed to hydromorphone after failed epidural or IT morphine trials ( ). No change in opioid-related side-effects was reported. Although average pain scores were significantly lower at the 3-month follow-up, worst pain scores were unchanged ( ). Despite a lack of controlled studies, hydromorphone is included in the algorithms for nociceptive and neuropathic pain as a first- and second-line agent, respectively. The recommended starting doses are 0.02–0.5 mg/day, up to a maximum of 10 mg/day, and with a maximum concentration of 15 mg/mL ( ).
Preclinical studies of IT hydromorphone in large-animal models showed granuloma formation at higher concentrations ( ). Two case reports described granuloma development in patients treated with IT hydromorphone. The first report described a patient who developed a granuloma using IT morphine. Nine months after removal of the first granuloma, the patient developed another granuloma 1 month after starting IT hydromorphone therapy ( ). The second report described a 52-year-old man with a history of chronic lumbar spine pain who developed a granuloma while receiving high-concentration IT hydromorphone (85 mg/mL) at a dose of 19.8 mg/day ( ).
Bupivacaine
Bupivacaine is an amide local anesthetic with high lipid solubility that is often used off label for IT therapy ( ). Several pharmacodynamic properties give it utility with spinal delivery: delivery of low bupivacaine concentrations alters sensory processing while sparing motor function; there is synergy with other analgesic targets in animal and humans models ( ); and there is an absence of tachyphylaxis in patients with neuropathic or somatic pain. Early rat studies using a continuous infusion of 0.5% bupivacaine showed modest increases in neuronal vacuolation ( ). On autopsy, cancer patients who received a combination of IT bupivacaine and morphine showed no significant histopathology of the examined spinal tissue ( ).
A retrospective study of 109 patients who were initially given opioid-only treatment and then switched to combination therapy with bupivacaine and opioids found improved patient pain control and decreased oral opioid consumption ( ). The average daily bupivacaine dose in that study was 10 mg. A small double-blind RCT of postlaminectomy syndrome patients suggested that the combination of bupivacaine and morphine or hydromorphone did not result in improved pain control ( ). However, a large study in noncancer pain patients showed reduced opioid dose escalation when using combination therapy. The average bupivacaine daily dose in those patients was 9.8 mg 1 year after implant ( ).
No formal studies have been performed to assess starting and maximal doses of bupivacaine for IT therapy, and there are no prospective studies of chronic IT bupivacaine administration as a sole agent. The most recent PACC guidelines suggest a maximal concentration of 30 mg/mL, a starting dose of 1–4 mg/day, and a maximal daily dose of 10 mg ( ). Serious cardiotoxic side-effects can occur when significant amounts of bupivacaine reach the bloodstream, but this does not occur with IT administration ( ). Clearly, the limiting factor for bupivacaine infusions would be sensorimotor loss. Nevertheless, bupivacaine doses as high 5 mg/h and bolus doses as high as 7 mg in the high cervical space have been reported without complication ( ). The most recent PACC guidelines recommend combination bupivacaine/morphine therapy for treatment of neuropathic pain ( ). Bupivacaine in combination with morphine, fentanyl, and hydromorphone is a second-line therapy when treating nociceptive pain ( ).
Clonidine
The PACC 2012 guidelines categorized the use of clonidine for neuropathic pain as a second-line treatment when used in combination with hydromorphone or morphine ( ). Clonidine is recommended as a third-line agent when used as a monotherapy or when combined with fentanyl. For nociceptive pain it is categorized as a third-line agent when used in combination with an opioid, and a fourth line when combined with an opioid and bupivacaine. The recommended starting dose is 40–100 mcg/day, and the maximum recommended dose is 600 mcg/day ( ).
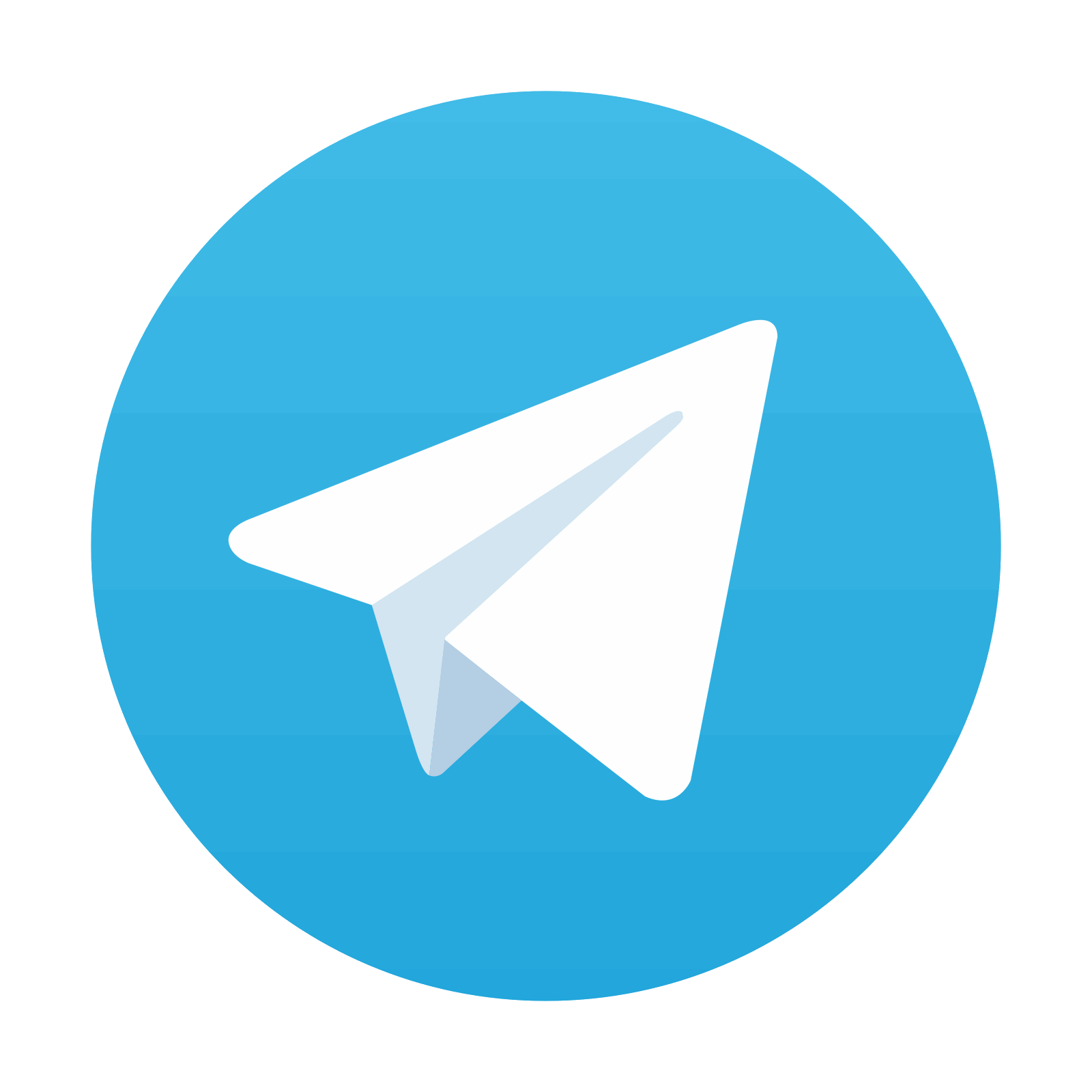
Stay updated, free articles. Join our Telegram channel

Full access? Get Clinical Tree
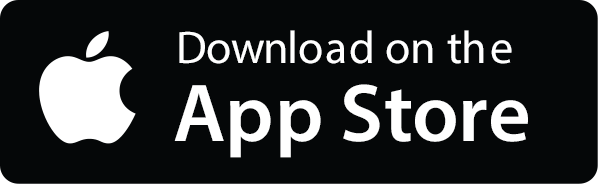
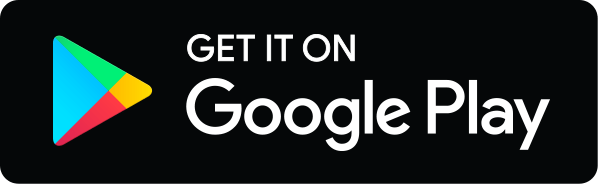