Abstract
Pain is an unpleasant sensory and emotional experience associated with actual or potential tissue damage, or described in terms of such damage. The experience of pain serves as one of the most important protective mechanisms of the body. Consistent with its important role, the physiology of pain spans the entire nervous system, from peripheral nociceptors to central thalamocortical loops. In this chapter we describe the physiology and pathophysiology of pain. After a brief summary of treatment strategies, featured in detail elsewhere in this chapter, we examine the flow and modulation of pain signals from the periphery to the center. Pain transmission is a complex process involving many levels of endogenous modulation. However, at each level the process can become pathologic and lead to development of chronic pain. These pathological processes and strategies for treatment are also discussed.
Keywords
Chronic pain, Neuropathic pain, Nociception, Pain modulation, Sensory transmission
Physiology and Pathophysiology of Chronic Pain
Pain Pathogenesis
Pain is an unpleasant sensory and emotional experience that involves complex processes of neuronal signaling in the peripheral nervous system and central nervous system (CNS). Chronic pain is defined as pain lasting >3–6 months and is very common in medical practice. It is reported by 20%–50% of patients seen in primary care ( ), with an estimated annual burden of $90 billion ( ). Pain is classified as either neuropathic or nociceptive, though frequently the types can coexist. According to the International Association for the Study of Pain, neuropathic pain is defined as the type of pain caused by a demonstrable lesion or disease of the somatosensory nerve structure itself. Classic signs of neuropathic pain are hyperalgesia, allodynia, and spontaneous pain without a stimulus. Common examples include diabetic neuropathy, postherpetic neuralgia, lumbar radiculopathy, multiple sclerosis, and complex regional pain syndromes. In contrast, nociceptive pain arises from actual or potential damage to nonneural tissue via the activation of nociceptors. Nociceptive pain can be somatic, such as acute pain after a burn, or visceral, such as the pain felt with abdominal cramps ( ).
Nociceptors
Nociceptors are very specialized receptors of primary sensory neurons that respond only to pain. They can be classified by the type of pain stimulation they conduct and their response to that stimulus as unimodal, responding to either mechanical or thermal stimulation, or polymodal, responding to thermal, mechanical, and chemical stimuli. Mechanoreceptors are those that are physically deformed, and include free nerve endings, Merkel’s discs, and Meissner’s and Pacinian corpuscles. However, only free nerve endings transmit noxious information, carried by both A delta (Aδ)- and C-fibers to the dorsal horn (DH) of the spinal cord, as shown in Fig. 43.1 . Other types of mechanoreceptors carry nonnoxious stimulation, such as light touch and vibration, via Aβ fibers. Thermoreceptors can transmit either hot or cold sensations, and are activated at free nerve endings. In addition to these stimuli, polymodal receptors can be activated by chemical stimuli, such as hydrogen ions, and are carried via C-fibers to the CNS. Among nociceptive neurons, Aδ-fibers are fast-conducting thinly myelinated fibers and result in immediate sharp pain. C-fibers are slow-conducting unmyelinated fibers which cause long-lasting pain that is typically described as dull ( ).

Peripheral Mechanisms of Chronic Pain
Peripheral mechanisms by which acute noxious stimulation becomes chronic include sensitization in nociceptive processes and the development of ephaptic or ectopic discharges in neuropathic pain. Peripheral sensitization occurs by upregulation of existing receptors and transcription of new nociceptive mediators. The process of receptor upregulation is initiated when inflammatory cytokines are released from injured cells and bind to their receptors, triggering an enzymatic cascade. Downstream phosphorylation leads to insertion of channels into the cell membrane, resulting in increased sensitivity to stimuli and resultant increased action potentials (APs) ( ). Increased transcription of nociceptive mediators also occurs after ligand binding, when new genes are transcribed and translated. For example, following interleukin-1β binding to its receptor, the NF-Κβ and mitogen-activated protein (MAP) kinase pathways are activated, leading to transcription of nociceptive mediators such as prostaglandins, interleukins, matrix metalloproteinases, and substance-P. These new protein complexes are then transported to the nociceptive nerve terminal, where they are released ( ).
Increased expression of cation channels and ligand-gated receptors has been associated with ectopic and ephaptic discharges, both of which have been implicated in the transition to chronic pain following neuronal injury ( ). Ectopic discharges occur after peripheral nerve injury when receptors, such as ligand-gated sodium channels, become upregulated on injured nerve endings and therefore more sensitive to stimuli. This can lead to abnormal discharges and development of pathologic oscillations ( ), causing spontaneous activation centrally ( ). Further, cell damage and injury cause an inflammatory response by inducing glial nociceptors to produce neuropeptides such as cytokines, chemokines, bradykinin, histamine, and prostaglandins. This results in changes in the chemical environment at the peripheral terminal of nociceptors, leading to an increased sensitivity to stimuli and ectopic activity ( ). Ephaptic discharges occur due to the abnormal spreading of signals via nerve fiber collaterals that results in APs being propagated to the DH in fibers that are not directly stimulated ( ).
Many of the pharmacologic therapies developed for pain relief are targeted at peripheral inflammatory mechanisms. Corticosteroids have potent antiinflammatory effects and inhibit inflammatory cytokine transcription and translation, as well as limiting macrophage response to inflammation and the resultant release of interleukins and tumor necrosis factor-α ( ). Tissue injury results in arachidonic acid release and prostaglandin synthesis via cyclooxygenase-2 (COX-2) activation, which then activate nociceptors. Inhibitors at any of these steps can reduce pain transmission, and include the irreversible inhibition of COX-2 by aspirin, COX-2 and prostaglandin inhibition by acetaminophen ( ), nonselective nonsteroidal antiinflammatory drugs (NSAIDs), and selective NSAIDs such as celecoxib and meloxicam ( ).
Nerve, Root, and Spinal Mechanisms
Once stimulated, Aδ- and C-fibers carry pain signals in peripheral nerves, intermixed with Aβ-fibers carrying other sensory information and efferent motor signals from the anterior horn of the spinal cord. At the level of the lateral spinal neural foramen the peripheral nerve divides into ventral and dorsal roots, where motor and sensory fibers separate. The dorsal root ganglion (DRG), the site of pseudounipolar cell bodies of sensory afferents, is located at the medial aspect of the foramen and continues as the dorsal root to the spinal cord, where it emanates into several rootlets and enters at the posterolateral sulcus ( ). While the pseudounipolar nature of the sensory neurons facilitates quick transmission of pain to the CNS, the location of the DRG exposes it to pathologic mobility at the intervertebral joints and make it subject to possible compression by herniated discs ( ). In addition, the DRG outer membrane lacks tight junctions (blood–DRG barrier), allowing increased permeability, which contributes to its sensitivity to both endogenous molecules and exogenously administered steroids, analgesics, or stimulation that provide relief ( ). The proximal axon of the DRG passes from the ganglion to the spinal cord, ascending several spinal levels in Lissauer’s tract, located in the posterolateral white matter. At this point, sensory fibers divide to synapse on second-order neurons in various Rexed laminae, depending on the type of sensory information they transmit ( ). Lamina I receives both Aδ- and C-fibers from somatic and visceral afferents ( ). Aδ-fibers also synapse in lamina V and C-fibers also synapse in lamina II, also known as the substantia gelatinosa (SG) ( ). Several dorsal root neurons from different levels may converge at a particular spinal level, providing one possible mechanism for pain integration at the spinal cord ( ).
The primary neurotransmitter released from first-order neurons at the synapse is glutamate, resulting in excitation of the postsynaptic membrane through binding to its receptors: the ionotropic cation channels α-amino-3-hydroxy-5-methyl-4-isoxazolepropionic acid (AMPA) receptor, the N-methyl- d -aspartate (NMDA) receptor, and kainite receptors ( ). In addition to glutamate, C-fibers release the peptides substance-P and calcitonin gene-related peptide (CGRP) ( ).
Though cognitive processing of pain information relies on ascending transmission from projection neurons, only about 5% of second-order neurons in the DH are responsible for this. The remainder are involved in local circuits and feedback, providing a complex network for modulation at this level ( ). Presynaptic inhibition is a process by which primary afferents release CGRP that binds to the postsynaptic membrane of GABAergic interneurons. These neurons then release GABA from axodendritic synapses at primary afferents, inhibiting further neurotransmission ( ). Other endogenous mediators within the DH include dynorphin, glycine, adenosine, enkephalin, galanin, somatostatin, neurotensin, and cholecystokinin, which act on their various receptors to give local modulation ( ).
Mechanisms of chronic pain development are believed to arise from physiologic processes that are altered or persist longer than the pain stimulus. Central sensitization occurs when the CNS amplifies synaptic transfer from the nociceptor terminal to DH neurons, and has several complex mechanisms. Repetitive noxious stimulation, such as that from pain generators implicated in low-back pain (facet joints, ligamentous injury, etc.), cause peripherally sensitized C-fibers to release glutamate, substance-P, and CGRP. These act on postsynaptic NMDA receptors and result in removal of a magnesium ion from the NMDA channel, enabling prolonged duration of channel opening, a process known as “wind-up.” This prolonged duration is translated into increased APs. Thus while the amplitude, or strength, of incoming stimulation remains constant, the DH transmits a greater frequency of stimulation via the ascending pathways ( ). In addition to central sensitization, these activities lead to peripheral sensitization, which in turn results in increased activity at the DRG itself, where repetitive APs cause a decreased firing threshold and ectopic discharges. Glial and Schwann cells in the DRG also become activated and release proinflammatory mediators such as eicosanoids, prostaglandins, and a host of cytokines, chemokines, growth factors, and interleukins. Changes in the excitability of sodium, potassium, and calcium ion channels in the DRG can also initiate spontaneous activity ( ).
Summation of repetitive stimulation also causes high intracellular concentrations of calcium, which leads to activation of second-messenger pathways such as the protein kinase system. This results in phosphorylation of AMPA receptors and increased sensitivity of NMDA receptors to glutamate. Another mechanism of sensitization and the pathologic potentiation of acute to chronic pain is mediated by the dorsal root reflex. It is believed that if a sufficiently strong stimulus is transmitted by the dorsal root, the fiber terminal can become depolarized and transmit an AP back to periphery. This can cause neurotransmitter release into peripheral tissues that lead to neurogenic inflammation and hyperalgesia, and cause the cycle to persist ( ).
Disinhibition is another mechanism leading to the persistence of pain. In normal circumstances inhibitory pathways prevent pain signals from transmission to the DH. However, blocking those pathways can have an effect similar to increased excitability, mainly through two mechanisms. First, GABA blockade can activate a silent synaptic pathway by recruiting new Aβ-fibers to lamina II cells. Second, partial nerve injury reduces inhibition in the DH by inducing apoptosis in GABAergic inhibitory interneurons ( ). As in the DRG, macrophage-like glial cells in the spinal cord are activated after nerve injury and enhance pain by releasing cytokines and chemokines, altering the gene transcription in neurons and glia ( ).
There are several currently available techniques for modulating local spinal circuits for pain control. Perhaps the best understood of these involves the administration of opioids, which act by binding to mu-opioid receptors dispersed throughout the CNS ( ). The binding of opioids and their derivatives block other nociceptive molecules, such as substance-P. In addition, morphine may cause presynaptic inhibition of neurotransmitter release from first-order neurons ( ) and may lead to receptor upregulation at the DRG ( ). Opioids have a common molecular mechanism of action that involves hyperpolarization of the nerve cell leading to decreased neuronal excitability. This occurs as a result of endogenous (e.g., endorphin) or exogenous (e.g., morphine, fentanyl) opioid agonists binding to the G protein coupled opioid receptor which activates inhibitory G proteins. The G alpha and G beta/gamma subunits dissociate from one another and subsequently act on various intracellular effector pathways. The G alpha protein inhibits adenylyl cyclase, resulting in reduced intracellular cyclic adenosine monophosphate and downstream phosphorylation, while the G beta/gamma subunit binds to the inward rectifying potassium channel, causing channel activation, hyperpolarizing the cell, and preventing signal propagation. Binding to the N-type calcium channel results in its deactivation, causing inhibition of further depolarization of the neuron ( ). Local anesthetics also work at the spinal cord level and inhibit sodium channels in the nerve fibers, resulting in inhibition of AP propagation ( ). As glutamate is the primary neurotransmitter released from pain fibers, antagonists of NMDA receptors inhibit pain transmission and are used to treat chronic pain. These include ketamine, typically used for acute pain, dextromethorphan, and memantine ( ). Due to the high doses required to treat refractory pain, an alternative route of delivery of these medications is directly to the intrathecal space with implanted pumps. Intrathecal delivery provides comparable pain relief at smaller does, reducing side-effects ( ). Medications delivered by the intrathecal route include morphine and hydromorphone, fentanyl, local anesthetics such as bupivacaine, the alpha-2 agonist clonidine, and ziconotide, a ligand of the N-type voltage-sensitive calcium channel ( ).
Spinal cord stimulation (SCS) also mediates its primary effects at the level of the DH, though supraspinal mechanisms have also been described. Current understanding of its efficacy is that stimulation attenuates the hyperactivity of sensitized DH second-order neurons, possibly through increased GABA release, which acts both presynaptically and postsynaptically. Furthermore, SCS may result in release of acetylcholine, adenosine, and serotonin, each of which act in various ways to exert analgesic effects ( ). Though more recently established, stimulation can also be applied directly to the DRG, which is postulated to modulate firing of cell bodies of Aδ- and C-fiber terminals ( ). Whereas SCS acts on the dorsal columns, leading to broad coverage of dermatomes, DRG stimulation provides more precise dermatomal coverage. SCS stimulation may also be attenuated by the flow of cerebrospinal fluid, movement of the spinal cord during normal activity, and migration of the leads. When leads are placed over the specific DRGs responsible for signal transmission, the result is a more predictable and focal pattern of stimulation. As a testament to its efficacy, a recent randomized controlled trial (the ACCURATE study) found that in complex regional pain syndromes DRG stimulation was overall successful in 81% of patients, compared to approximately 57% of those receiving SCS ( ).
In addition to modulating spinal cord circuits, these pathways can be ablated, interrupting transmission of noxious stimulation directly. One example of this is dorsal root entry zone lesioning, the clinical indication of which is most commonly deafferentation pain after spinal root avulsion, such as occurs with brachial plexus injury. An incision is made into the posterolateral sulcus where dorsal rootlets enter the spinal cord, directed towards the medial aspect of Lissauer’s tract and ideally extending deep to lamina V ( ). Rhizotomy and ganglionectomy are additional ablative procedures, though the indications for these are limited to cancer pain in specific thoracic or pelvic dermatomes, or occipital neuralgia, in which the C2 and C3 ganglia or roots are transected ( ). Alternatively, occipital stimulation can be performed by subcutaneous insertion of leads over the occipital area ( ).
Spinal and Subcortical Pain Signaling
Following propagation of signal transmission to the spinal cord, second-order projection neurons send axons rostrally to areas of the brainstem and thalamus. The primary systems for signal transmission include a direct anterolateral pathway, the spinothalamic tract, ascending directly to the thalamus, and an indirect anterolateral pathway comprising the spinoreticular tract, which synapses in the reticular formation, and the collateral spinomesencephalic, spinotectal, and spinohypothalamic tracts ( ).
Neurons of the spinothalamic tract are located in laminae I, IV, and V, with less prominent aggregates being found in deeper laminae of the spinal cord. Axons initially cross the midline in the anterior (ventral) white commissure before ascending in the lateral and ventral funiculi to the various rostral structures via the lateral and ventral spinothalamic tracts ( ). Most axons from the body and face project to the contralateral ventral posterolateral (VPL) and ventral posteromedial thalamus (VPM), respectively ( ). Neurons from laminae II and III ascend in the spinoreticular tract, with most traveling ipsilaterally and a smaller proportion decussating in the anterior white commissure. After synapsing in the medullary reticular formation, just ventral to the cuneate nucleus, they travel to the intralaminar nuclei of the thalamus via reticulothalamic fibers ( ). Fibers in the spinomesencephalic tract ascend to the periaqueductal gray (PAG), raphe nuclei, and parabrachial nucleus, and then send projections to the amygdala. Spinotectal fibers ascend to the superior colliculi, which play a role in head and eye version toward a stimulus. A spinohypothalamic tract ascends from neurons primarily in laminae I and X, and synapses in the lateral hypothalamus and central amygdala. In addition to contributing to the descending control of pain, these collateral pathways are implicated in the emotional aspect of pain perception ( ).
Descending modulation of pain transmission plays a central role in the ability to suppress pain states. Perhaps the most important descending pathway is from the PAG and rostral ventromedial medulla (RVM). The PAG sends axons to the RVM, where they synapse on cells of the raphe nuclei and nucleus gigantocellularis. Axons from these serotonergic cells descend bilaterally in the spinopetal tract, located anatomically in the lateral funiculi of the spinal cord at all spinal levels. The axons synapse on second-order neurons in the DH, releasing serotonin, leading to inhibition of pain transmission ( ). A second major descending modulatory pathway originates at the dorsolateral pontine reticular formation, where neurons releasing norepinephrine send axons to the SG via the dorsolateral funiculus. In the SG they synapse on interneurons that release GABA, leading to inhibition of second-order neurons ( ).
While descending pathways provide a mechanism of endogenous pain suppression, alterations in their activity are implicated in the development of chronic pain. “Descending facilitation” is a broad term referring to the increased activity of the PAG and RVM and their tracts that results in hyperalgesia, allodynia, and dysesthesias experienced in chronic pain. Various studies have shown the mediators of facilitation to be cholecystokinin, opioids, and dynorphins.
Another mechanism of chronic pain development attributable to descending pathways is loss of diffuse noxious inhibitory control (DNIC). In physiologic states, DNIC refers to the theory that peripheral noxious stimulation transmits painful sensation from one body area while invoking inhibition of pain sensation at a different site. The underlying mechanism is proposed to be a center–surround concept in which “on cells” are activated in response to painful stimulation, while the same stimulation activates adjacent inhibitory “off cells,” leading to analgesia in areas not directly affected. Loss of this DNIC has been postulated to explain aberrant pain sensations ( ).
Treatment of chronic pain directed at the ascending and descending pathways can be pharmacologic, modulatory, or ablative. Pharmacologic treatment primarily involves administration of serotonin and adrenergic analogs that facilitate descending inhibitory pathways. Cannabinoids have also been used, and may exert their effects at both the PAG and the RVM. Opioids bind to receptors throughout the neuraxis to activate descending pathways ( ). Stimulation of the PAG ( ) is based on observations that in rats stimulation of this area produces profound antinociception ( ). However, it can lead to side-effects of anxiety and distress ( ), which has contributed to its lack of widespread adoption.
Ablative techniques that target ascending tracts include cordotomy and myelotomy. Cordotomy is used primarily for unilateral cancer pain, and has evolved from an open procedure to a percutaneous image-guided and minimally invasive operation that can be performed under local anesthetic and light sedation ( ). The lesion is directed at the anterolateral spinothalamic tract in the lateral funiculus for disruption of pain transmission. Likewise, a myelotomy creates a lesion in the midline dorsal column that is known to convey visceral pain sensation from neurons, originating primarily in laminae III and IV ( ). Unfortunately, due to the large number of collateral projects via indirect pathways, these procedures are not durable methods of pain control and are primarily used in terminal patients ( ).
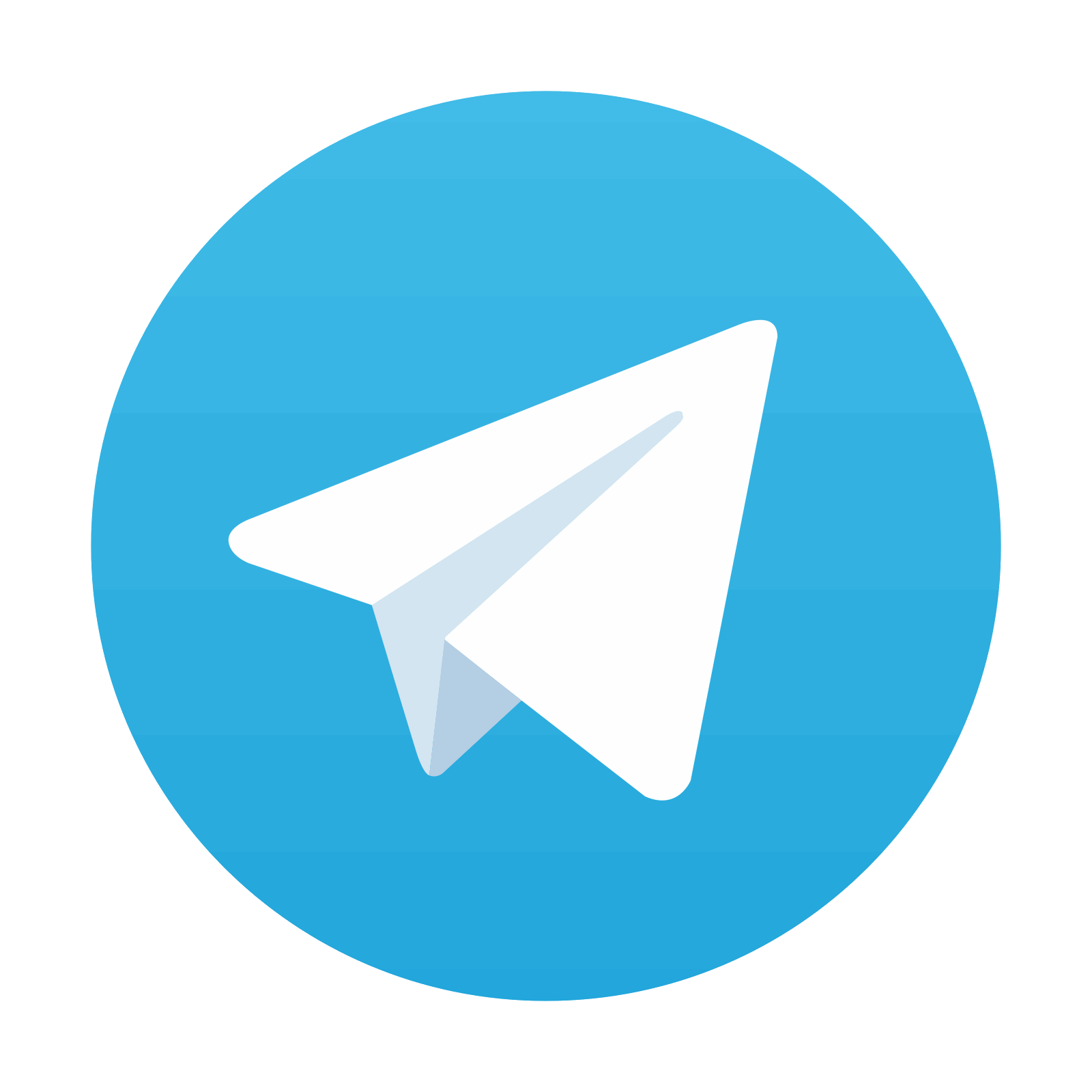
Stay updated, free articles. Join our Telegram channel

Full access? Get Clinical Tree
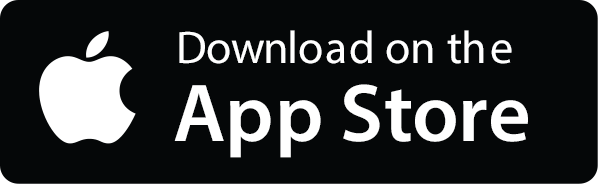
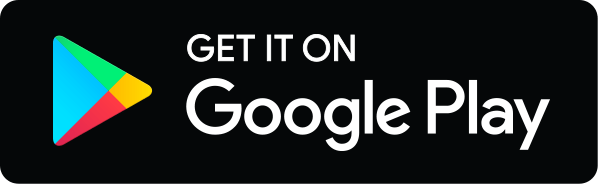