Sagittal T1-weighted MRI scans demonstrating progressive atrophy of the corpus callosum in an individual with MS (courtesy of John R. Corboy, MD).
Brain and cognitive reserve
In 1937, the noted British neurologist Sir Charles Symonds proposed the notion that the effects of brain lesions were determined not only by the insult sustained but also by the preexisting condition of the brain. “In other words,” Symonds wrote in a discussion of the mental sequelae of head injury, “it is not only the kind of injury that matters, but the kind of head” (Symonds, 1937). This idea reflects a general understanding among clinicians that any brain affliction can be seen as producing its effects on cognition not only by virtue of the specific location and severity of neuropathology, but also because of the state of the brain at the time the disorder begins. In an effort to develop this concept in more detail, recent studies in clinical neuroscience have proposed the idea of a reserve capacity that functions to modify, delay, or even prevent the onset of neurobehavioral disorders (Stern, 2002, 2009). Following Symonds’s lead, the concept has since been applied not only to TBI but to vascular injury, MS, HIV infection, Parkinson’s Disease, schizophrenia and other neuropsychiatric disorders, aging, and the degenerative dementias (Satz, 1993; Allen, Bruss, and Damasio, 2005; Stern, 2012).
The reserve concept posits that the brain may be protected against the ravages of cognitive disorders by either genetic endowment or environmental influences that can mitigate the effect of neuropathology. A distinction has been drawn between brain reserve, the physical attributes of an individual’s brain – mainly its size and number of neurons – that may reduce the individual’s susceptibility to the effects of brain disease (Satz, 1993, 2012), and cognitive reserve, the previously acquired neural resources of an individual brain that allow effective task performance even in the presence of brain disease (Stern, 2009). Brain reserve is determined by the innate, congenital, and developmental aspects of brain organization, and thus invokes many genetic factors as well as a range of environmental variables such as prenatal care, nutrition, and the characteristics of child rearing. Cognitive reserve is a function of the extent of engagement in cognitive stimulation, which largely involves educational attainment, occupational accomplishment, and socialization across the life span. Brain reserve can therefore be considered a passive phenomenon, based essentially on the general health of the brain being affected by acquired insult, whereas cognitive reserve implicates an active process that increases brain capabilities by the individual’s frequent engagement in cognitive activities (Stern, 2002, 2009). Another implication of this distinction is that brain reserve involves the advantages conferred by the premorbid structure of the brain, while cognitive reserve implies that premorbid brain function has been enhanced by a cognitively stimulating lifestyle (Stern, 2009, 2012).
In the dementias, brain and cognitive reserve have been most investigated in the cortical dementias, notably Alzheimer’s Disease (AD), in which it has become clear that these advantages do indeed offer some degree of protection. Brain reserve, indexed by brain size, appears by itself to be protective, as the appearance of dementia related to AD neuropathology is decreased in people with larger brains (Katzman et al., 1988; Schofield et al., 1997). While an overly large brain can of course be associated with cognitive impairment or dementia because of neuropathology related to excessive and thus dysfunctional brain mass (Miles et al., 2009), a larger brain in normal adults, within the standard range of brain size, appears to be mildly protective against AD.
Cognitive reserve also seems to be of benefit, as educational and occupational attainment can also provide some degree of protection from AD (Stern, 2012). This phenomenon first became clear in epidemiological studies from China examining the effect of education on the prevalence of AD (Zhang et al., 1990). The disease cannot be completely prevented by such factors, as the application of demanding mental tasks does not reverse the neuropathology, and indeed the tempo of cognitive decline may be similar in all cases once the reserve capacity of the brain is exhausted (Filley and Cullum, 1997). However, it is generally thought that intellectually stimulating activities offer a salutary means of increasing the integrity of the cortex as it copes with the onset of AD neuropathology. Given that patients with AD have reduced synaptic density that correlates with cognitive impairment (DeKosky and Scheff, 1990), the demonstration of increased synaptic density in laboratory animals exposed to enriched environments (Greenough and Bailey, 1988) assumes special relevance. Experimental animal evidence continues to support the notion that increased synaptic density plays an important role in the mediation of cognitive reserve (Petrosini et al., 2009), and the contribution of neurogenesis is increasingly seen as likely to be important as well (Brown et al., 2003). Thus the number of both synapses and neurons in the cerebral cortices may be important as protective advantages in AD that are enhanced by environmental stimulation.
An intriguing study of normal aging recently appeared that supports the role of brain and cognitive reserve in mitigating the cognitive effects of age-related white matter loss (Daselaar et al., 2015). In older adults whose cognitive function was examined together with functional MRI (fMRI) and diffusion tensor imaging (DTI) data, cortical activity was found to be inversely related to lower white matter integrity in closely related cerebral areas (Daselaar et al., 2015). This finding was interpreted as evidence of the concept of “less wiring, more firing,” implying that compensatory activity of cortical regions is recruited to accomplish a relevant cognitive task in the setting of less efficient white matter (Daselaar et al., 2015). The mechanism of such a phenomenon is almost certainly increased synaptic activity.
Among the white matter disorders, MS has been best studied, and neuropsychological data suggest that brain and cognitive reserve can indeed protect against cognitive dysfunction in this disease (Sumowski, Chiaravalloti, and DeLuca, 2009; Langdon, 2011; Sumowski et al., 2014). The mechanism may be similar to that postulated in AD; that is, cortical synaptic density and perhaps neuronal numbers are increased in MS patients who have experienced greater intellectual stimulation, and this advantage may help compensate for the white matter (and to some extent gray matter) neuropathology of the disease. Studies with fMRI have shown that cognitive reserve in MS may rely on the activity of the default mode network, which includes the anterior and posterior cingulate cortices, medial frontal regions, precuneus, and medial and lateral temporal lobes (Sumowski et al., 2010). Patients with MS display considerable variability in their cognitive impairment, and in part this observation may be due to the individuals’ premorbid intellectual ability, which in turn relates to both maximal lifetime brain growth and educational and occupational enrichment that mitigates the impact of the neuropathology (Sumowski et al., 2014). Studies in other white matter disorders, including TBI (Kesler et al., 2003), ischemic white matter disease (Dufouil, Alperovitch, and Tzourio, 2003), and HIV infection (Farinpour et al., 2003), also support the notion that reserve can be beneficial in modifying the impact of neuropathology. Thus evidence is accumulating that the cognitive burden imposed by white matter neuropathology may be modified by the capacity of the cerebral cortex to compensate because of an increase in the number of its synapses and neurons.
While these data are of considerable interest in the process of assessing the prognosis of potentially any dementing disease, they apply largely to the notion of plasticity in the cerebral cortex. More intriguing still is the recent idea that experience-dependent neural changes may also occur in the white matter, and have important effects on the cognitive prognosis of white matter disorders.
Plasticity
A fascinating idea pertinent to the recovery of damaged white matter is the notion of plasticity, more generally known as neural plasticity. Operationally defined as the ability of the central nervous system to adapt to environmental changes or acquired lesions (Sharma, Classen, and Cohen, 2013), neural plasticity has recently drawn considerable neuroscientific attention in light of emerging evidence that the brain may possess more adaptive capacity than formerly thought. Long known to be a property of gray matter related to synaptic function, particularly in the cerebral cortex (Majewska and Sur, 2006), plasticity in this conventional sense likely plays a critical role in recovery after many neurologic and neuropsychiatric disorders (Ward and Frackowiak, 2004). However, plasticity has also recently been observed to occur in the cerebral white matter (Fields, 2010; Zatorre, Fields, and Johansen-Berg, 2012; Boulanger and Messier, 2014). This rather arresting notion immediately seems counterintuitive to most neurologists and neuroscientists, accustomed as they have become to a corticocentric perspective. The white matter is often regarded as providing little more than structural connectivity between gray matter regions, acting only to transfer information, and the remarkable capacity of the brain to exhibit plastic changes in response to experience has been seen by most as an exclusive property of gray matter. But this view requires reconsideration.
White matter plasticity is a measurable phenomenon that has been shown both in normal individuals, such as piano players whose pyramidal tract integrity correlated with number of hours practiced (Bengtsson et al., 2005), and in neurologic patients, such as those with Broca’s aphasia in whom the right arcuate fasciculus volume increased as melodic intonation therapy improved language performance (Schlaug, Marchina, and Norton, 2009). A recent review of the DTI literature on the effects of musical training in normal people concluded that, among many white matter tracts that may be potentially altered by musical training, the corpus callosum is the one where a beneficial effect of music on white matter connectivity is most compelling (Moore et al., 2014). Equally intriguing are findings indicating that bilingualism is associated with the maintenance of white matter integrity (Luk et al., 2011; Li, Legault, and Litcofsky, 2014), implying that the regular use of more than one language may recruit mechanisms of plasticity within myelinated tracts. Furthermore, DTI data gathered from the fornix of both rats and humans have suggested that structural changes indicative of plasticity may occur after as little as 2 hours of engagement in a learning task (Hofstetter et al., 2013).
These observations suggest that individuals with WMD and MCD may benefit from engagement in cognitive activity that helps restore white matter integrity. But there is also reason to think that physical activity may have similar effects. Emerging neuroimaging evidence, for example, has shown that lifelong physical exercise not only can reduce the number of MRI white matter hyperintensities, but can also increase white matter microstructural integrity (Tseng et al., 2013; Herting et al., 2014).
Laboratory observations have helped elucidate the means by which such changes occur, and the discovery of white matter synapses has been pivotal in this work. White matter plasticity may be mediated by novel glutamatergic axo-oligodendroglial synapses lying within the white matter, which appear to be capable of inducing oligodendrocytes to myelinate distal axonal segments upon stimulation of the proximal axon (Alix and Domingues, 2011; Wake, Lee, and Fields, 2011; Boulanger and Messier, 2014). Thus an increase in the electrical activity coursing through an axon leads to greater distal myelination. In a white matter tract relevant to the task involved, the result of this phenomenon is an expansion of tract volume, and a concomitant increase in white matter integrity.
The specific impact of cognitive engagement and physical activity on white matter is thus becoming clear, and the clinical benefits of this form of plasticity are likely to be substantial. While changes in gray matter would also be expected with such regular habits, the effects on white matter may be equally or perhaps even more apparent. The prognosis of WMD and MCD may thus be meaningfully improved by such activities as playing a musical instrument, learning another language, and staying fit.
More study is needed to understand the application of white matter plasticity to clinical populations, and an obvious setting where this work could be readily accomplished is in the area of stroke rehabilitation (Johansson, 2011; Cumming, Marshall, and Lazar, 2013; Chen et al., 2014). Patients with a single ischemic stroke, featuring both a quantifiable cognitive deficit and a discrete white matter lesion, present a valuable opportunity to assess the impact of various rehabilitation strategies on the role of white matter plasticity in cognitive recovery. But the implications for recovery after any white matter lesion may be profound, and many opportunities for investigation can be imagined. These ideas naturally blend in with the treatment of white matter disorders affecting cognition, and will be further considered in the chapter to follow.
On a larger scale, data on white matter plasticity such as these may inform decision making in the arena of public policy. Whereas these concerns extend beyond the scope of routine medical practice, the promotion of healthy lifestyles that support and maintain white matter integrity under the assault of accumulating insults such as ischemic, traumatic, and leukotoxic injury may warrant focused attention as a public health objective. Societal policies aimed at improving the capacity of the brain to endure the effects of acquired insults, and then to respond more effectively when they occur, have the potential to offer significant benefits to the population as a whole.
References
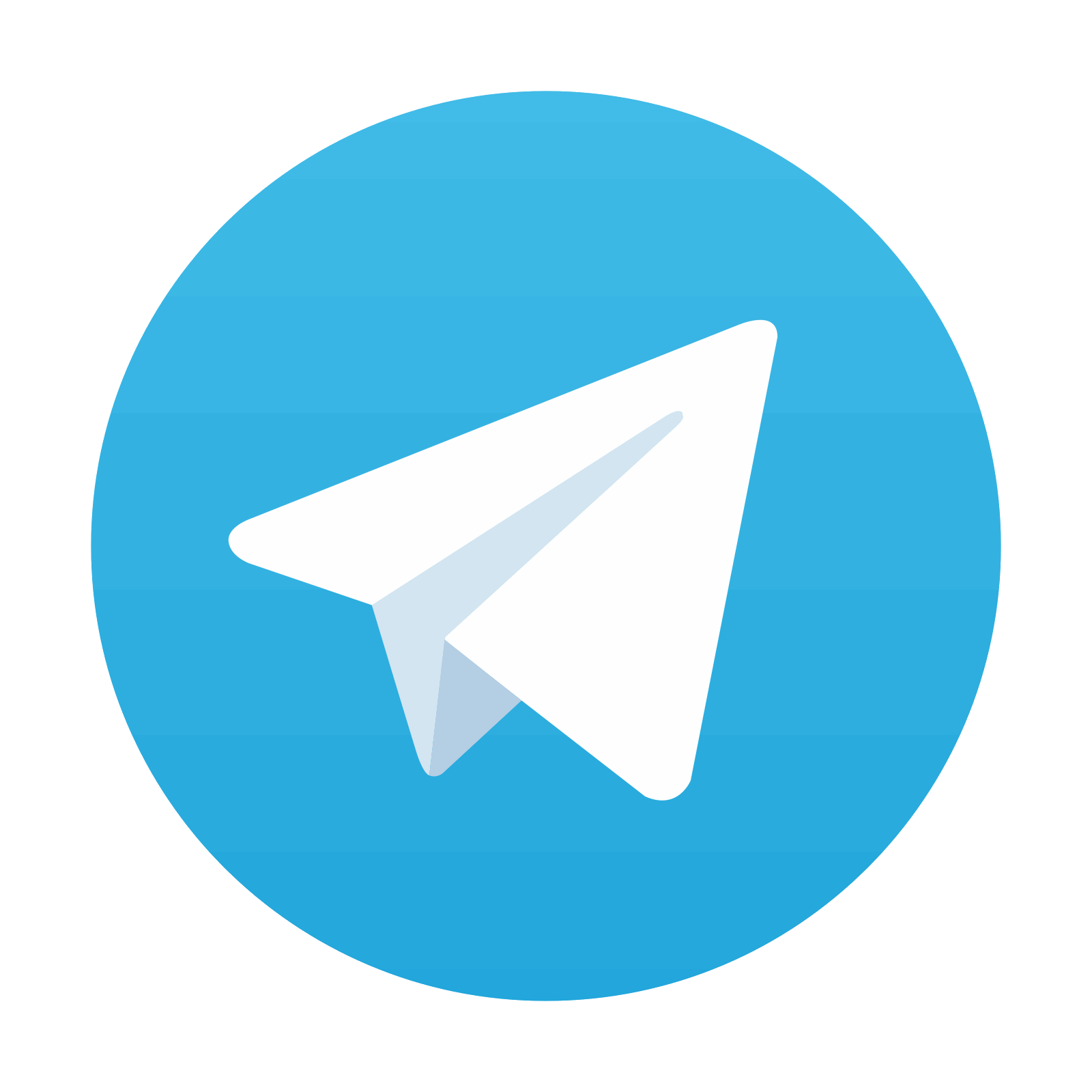
Stay updated, free articles. Join our Telegram channel

Full access? Get Clinical Tree
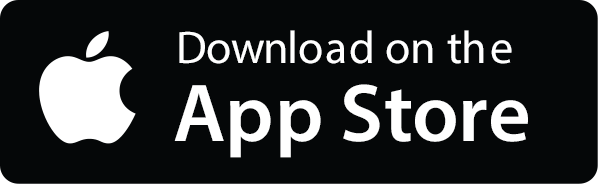
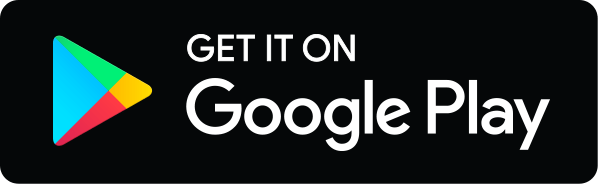