CHAPTER 18 Radiology of the Spine
Technical Considerations
Radiographs
The usefulness of conventional radiographs is primarily in the extradural space. Conventional radiographs are relatively inexpensive and easy to perform. They provide a convenient means of assessing alignment and gross bony integrity and can also be used for purposes of localization and evaluation of movement. They are capable of demonstrating the general changes involving various types of arthritis and disk space narrowing. According to recent appropriateness criteria, radiographs are considered sufficient for the initial evaluation of recent significant trauma, osteoporosis, or back pain in individuals older than 70 years.1 Substitution of digital radiography for conventional film-screen techniques has provided more flexible handling of images (e.g., duplication, transmission, postprocessing), as well as expedited cycle time. This latter advantage is extremely useful in the intraoperative environment because the results are available immediately after exposure without the need for traditional processing.2 The disadvantages of conventional radiographic techniques are that they are able to visualize only bony structures and are relatively insensitive to soft tissue changes within the disk, ligaments, nerves, and paraspinal tissues. Bone marrow involvement must be significant and far advanced before it is demonstrable on conventional radiographs.
CT Myelography
The introduction of contrast material into the intrathecal space provides both extradural and intradural information. Myelography is able to outline the nerve roots, spinal cord, and its coverings. Intradural and extradural disease can be identified and characterized on the basis of the character and morphology of its effect on the contrast column (Fig. 18-1). Plain-film contrast-enhanced myelography is rarely performed on its own and is almost always used in conjunction with CT. Nonionic contrast material has completely replaced its oil-based predecessor and is usually introduced into the lumbar region at L3 or L4 or cervically at C1-2. Plain-film conventional myelography may be useful for general anatomic appraisal in patients with complex deformities or when implanted hardware or electronic devices preclude the use of MRI or CT. CT myelography provides excellent delineation of intradural structures such as the spinal cord and nerve roots and their relationship to the soft tissue and bony surroundings. It is useful in distinguishing bone from soft tissue changes (e.g., osteophytes and disk herniations), which may be inseparable on plain-film myelography (Fig. 18-2). Multidetector CT of the entire spinal axis can be performed in 20 to 30 seconds. With intrathecal contrast media, subsequent multiplanar reformatted images can provide complex 3D assessment of anatomic and pathologic abnormalities with myelographic-like images. More recently, various forms of dual-energy multidetector CT have been introduced that can subtract out bone in both single slices and 3D multiplanar reformatted images (Fig. 18-3).
Magnetic Resonance Imaging
Traditional clinical imaging has emphasized orthogonal T1- and T2-weighted imaging for morphologic assessment of the diskovertebral complex. These sequences also provide an evaluation of the changes in signal intensity associated with degenerative disk disease. Fast spin echo (SE) T2-weighted images have replaced conventional T2-weighted images because of their shorter acquisition times, but they provide no increased diagnostic advantage. Short tau inversion recovery (STIR) or fat-suppressed T2-weighted images have been added because they are more sensitive to marrow and soft tissue changes (Fig. 18-4). Although these standard sequences remain the mainstay of diagnostic MRI of the spine, new techniques continue to be evaluated in the hope of providing stronger correlation between imaging findings and patient symptoms. The utility of many of these techniques for the routine evaluation of degenerative disk disease remains unknown, and the number of patients in whom they have been evaluated remains small. Nevertheless, these approaches may be important for redefining the direction of spinal imaging from strictly anatomic imaging to imaging that combines more physiologic and functional information.3 Techniques that have been evaluated include assessment of spinal motion (dynamic imaging, kinetic assessment, or axial loading), diffusion imaging (water or contrast agents), MR neurography, spectroscopy, functional MRI of the spinal cord, and ultrashort–echo time imaging. Of the variety of techniques available, only MR neurography and dynamic imaging have expanded beyond the experimental phase to demonstrate specific clinical utility (albeit in niche populations).
Dynamic Imaging
The utility of dynamic spine MRI remains unclear, in part because of the varied methods used. Methods used to date include axial loading in the supine position by means of a harness attached to a nonmagnetic compression footplate with nylon straps that can be tightened or the use of an upright open MRI system that allows flexion-extension imaging (Fig. 18-5).4,5 Dynamic MRI has been used to evaluate for the presence of occult herniations, which may not be visible or be less visible when the patient is supine, to measure motion between spinal segments, and to measure canal or foraminal diameter when subjected to axial loading.3,5–8 Hiwatashi and colleagues evaluated 200 patients with clinical symptoms of spinal stenosis and found 20 with detectable differences in caliber of the dural sac on routine and axial-loaded studies.7 In 5 of these selected patients, all three neurosurgeons involved in the clinical evaluation changed their treatment recommendation from conservative management to decompressive surgery. Although a subset of patients may benefit from this type of evaluation, the benefit appears to be small for the added machine time and patient discomfort.
Neurography
A large and varied literature exists concerning the use of MR neurography for the evaluation of peripheral nerves, including the brachial and lumbar plexuses. Thin-section MR neurography uses high-resolution T1-weighted imaging for anatomic detail and fat-suppressed T2-weighted or STIR imaging to show abnormal nerve hyperintensity. Several reviews exist on this subject.9–11 The technique is capable of depicting a wide variety of pathologic conditions involving the sciatic nerve, such as compression, trauma, hypertrophy, neuroma, and tumor infiltration.12,13 MR neurography has 93% specificity and 64% sensitivity for diagnosis of the piriformis syndrome (piriformis muscle asymmetry and sciatic nerve hyperintensity).14
Ultrashort–Echo Time Imaging
Routine clinical MRI does not allow evaluation of tissues with very short relaxation times because echo times are on the order of 8 to 15 msec. Ultrashort–echo time sequences have been preliminarily evaluated for a number of tissues, including the spine. These sequences have echo times as short as 0.08 msec. The images show normal contrast enhancement, with high signal intensity from the longitudinal ligaments, end plates, and interspinous ligaments.15–17
Diffusion
Several authors have evaluated the apparent diffusion coefficient (ADC) in normal and degenerated intervertebral disks. Antoniou and associates evaluated the ADC in cadaveric human disks in relation to matrix composition and matrix integrity by using a stimulated echo sequence.18 They found that the ADC in healthy subjects was significantly greater in the nucleus pulposus than in the annulus fibrosus. The ADC in the nucleus was noted to generally decrease with degeneration grade and age. A similar correlation of ADC measurements and annular degeneration was not found. The most notable correlations were observed between the ADC of the nucleus pulposus and the water and glycosaminoglycan content. Kealey and coworkers evaluated 39 patients with a multishot SE echo planar technique.19 They found a significant decrease in the ADC of degenerated disks in comparison to normal disks. Kurunlahti and colleagues evaluated the ADC of disk and lumbar magnetic resonance angiograms in 37 asymptomatic volunteers.20 Lumbar artery status correlated with diffusion values within the disks, thus suggesting that impaired blood flow may play an important role in disk degeneration.
Kerttula and collaborators compared disk ADC values in normal controls with those in patients with prior compression fractures (at least 1 year previously) and found that ADC values in the x and y directions decreased in degenerated disks and in disks of normal signal intensity in the area of trauma.21 Diffusion tensor imaging has been evaluated for imaging of the annulus fibrosus22 and for imaging defects or disruptions within the annulus.3 Differences in diffusion have been demonstrated for the intervertebral disk in compressed versus uncompressed states.23 Intravenous contrast enhancement may also be used to assess diffusion into the intervertebral disk. Normal disks enhance slowly after the injection of contrast material, which may be as much as 36% in animal models. This enhancement is modified by the type of contrast agent (ionic versus nonionic and molecular weight).24,25 Ionic material diffuses less rapidly than nonionic media into the disk. Degenerated disks with decreased glycosaminoglycan exhibit more intense and rapid enhancement.26 Disk enhancement has been documented in normal and degenerated human lumbar disks.27
Cerebrospinal Fluid
CSF flow studies with cine MRI provide cardiac-gated gradient phase-contrast images that are displayed qualitatively as CSF flow images in a closed loop kinematic format. This same data set can be displayed quantitatively on a graph or in numerical format as analysis of values of flow velocity and volume flow rate. These images demonstrate the pulsatile motion of CSF, not the bulk flow. Pulsatile flow is a result of expansion of the brain during systole and relaxation during diastole and is therefore bidirectional, with CSF flowing caudally during systole and cranially during diastole. Typically, the signal intensity of normal CSF flow in these studies is demonstrated as hyperintense during systole, where there is caudal (downward) flow, and hypointense during diastole, where there is cranial (upward) flow. These images are usually displayed in the sagittal and axial planes. In general, the degree of pulsatile flow diminishes as it proceeds caudally. Clinically, these studies are most often used for the evaluation of CSF flow in patients with Chiari I and II malformations and for the assessment of syrinx cavities (Fig. 18-6).
Artifacts and Contraindications
Instrumentation, metal used as part of surgical procedures, and implanted electronic devices represent challenges for both CT and MRI. In the case of CT, metal can create beam-hardening artifacts that obscure adjacent soft tissue and osseous structures (Fig. 18-7). Multidetector studies with isotropic voxels and software used to decrease beam-hardening artifact can often decrease the image degradation caused by implanted metal.28 Different types of metal produce different types of artifacts on MRI that may make the examination uninterpretable. The term magnetic susceptibility describes the manner and amount by which a material becomes magnetized in a magnetic field. Nonferrous magnetic metals may produce local electrical currents induced by the changing field, which causes distortion of the field and artifacts. These artifacts take two main forms: geometric distortion and signal loss secondary to dephasing (Fig. 18-8). Different techniques are more susceptible to these artifacts, and gradient echo images in particular are especially sensitive to differences in magnetic susceptibility and field homogeneity. Although metals can cause artifacts that render the examination uninterpretable, certain indwelling devices may be a contraindication to the entire examination. Certain types of aneurysm clips, cardiac valves, and vascular devices can result in harm to patients during an MRI examination. Some implantable devices may cease to function properly after an MRI study, and others may be a source of heating with deleterious biologic effects and burns. Readers are referred to publications and websites that track information related to contraindications.28
Intravenous contrast agents are commonly used with both CT and MRI examinations. Both iodinated (CT) and paramagnetic substances (MRI) are cleared by the kidney and should be used with caution or not at all in patients with impaired renal function. Although this has been common knowledge with the iodinated contrast media used for CT, recent evidence has also documented various complications related to the use of paramagnetic contrast agents in patients with impaired renal function. Despite being uncommon, nephrogenic systemic fibrosis, a late serious adverse reaction to gadolinium, has been well documented.28,29
Thermography, Diskography, CT Diskography
Most expert panels believe that thermography is too nonspecific to be of significant value for the evaluation of spinal disorders. Diskography is a long-standing provocative technique that by nature of direct stimulation, is thought by some to be able to identify painful and concordant disks.1,30 Diskography and CT diskography are still used, especially when other imaging modalities have failed to localize the cause of pain. The morphologic information is not as critical as reproduction of a patient’s characteristic pain. Although some data support its use, prospective well-controlled trials in support of its prognostic value remain absent from the literature.
Degenerative Disk Disease and Stenosis
Degenerative Disk Changes
The major cartilaginous joint (amphiarthrosis) of the vertebral column is the intervertebral disk. Each disk consists of an inner portion, the nucleus pulposus, surrounded by a peripheral portion, the annulus fibrosus. The nucleus pulposus is eccentrically located and is closer to the posterior surface of the intervertebral disk. With degeneration and aging, type II collagen increases outwardly in the annulus, and there is greater loss of water from the nucleus pulposus than from the annulus. This results in a loss of the hydrostatic properties of the disk, with an overall reduction in hydration in both areas to about 70%. In addition to water and collagen, the other important biochemical constituents of the intervertebral disk are the proteoglycans. The individual chemical structures of the proteoglycans are not changed with degeneration, but their relative composition is. The ratio of keratin sulfate to chondroitin sulfate increases, and there is a diminished association with collagen, which may reduce the tensile strength of the disk. The decrease in water-binding capacity of the nucleus pulposus is thought to be related to the decreased molecular weight of its nuclear proteoglycan complexes (aggregates). The disk becomes progressively more fibrous and disorganized, with the end stage represented by amorphous fibrocartilage and no clear distinction between the nucleus and annulus.31–33 On T2-weighted images, central disk signal intensity is usually markedly decreased and at distinct variance to that seen in unaffected disks of the same individual. Work with T2-weighed SE sequences34 suggests that MRI is capable of depicting changes in the nucleus pulposus and annulus fibrosus associated with degeneration and aging based on the loss of signal intensity (Fig. 18-9). In work with cadaver spines of various ages, absolute T2 measurements correlated more closely with the glycosaminoglycan concentration than with the absolute water content. Thus, the signal intensity may not be related to the total amount of water but rather the state of the water. At present, the role that specific biochemical changes (proteoglycan ratios, aggregation of complexes) plays in the altered signal intensity is not well understood. Given that the T2 signal intensity in the disk appears to track the concentration and regions with a high percentage of glycosaminoglycan more than the absolute water content, it seems likely that the health and status of the proteoglycans are major determinants of signal intensity.35 It has been proposed that annular disruption is the critical factor in degeneration and that when a radial tear develops in the annulus, there is shrinkage with disorganization of the fibrous cartilage of the nucleus pulposus and replacement of the disk by dense fibrous tissue with cystic spaces (Fig. 18-10).36–40 Annular tears, also properly called annular fissures, are separations between annular fibers, avulsion of fibers from their insertions on the vertebral body, or breaks through fibers that extend radially, transversely, or concentrically and involve one or many layers of the annular lamellae. The term tear or fissure describes the spectrum of such lesions and does not imply that the lesion was caused by trauma (see Fig. 18-1). Although it is established that annular disruption is a sequela of degeneration and is often associated with it, its role as the causal agent of disk degeneration has not been proved. MRI is the most accurate anatomic method for assessing intervertebral disk disease. The signal intensity characteristics of the disk on T2-weighted images reflect changes caused by aging or degeneration. A classification scheme for lumbar intervertebral disk degeneration has been proposed that has reasonable intraobserver and interobserver agreement.41 To date, however, there has been no correlation between disk changes on MRI and a patient’s symptoms. With loss of water and proteoglycans, the nucleus pulposus is desiccated and friable with yellow-brown discoloration. Its onion skin appearance begins to unravel, and cracks, clefts, or crevices appear within the nucleus and extend into the annulus fibrosus. Fissuring, chondrocyte generation, and formation of granulation tissue may be noted within the end plate, annulus fibrosus, and nucleus pulposus of degenerative disks and are indicative of attempts at healing.40 Radiolucent collections (vacuum disk phenomena) representing gas, principally nitrogen, occur at sites of negative pressure produced by the abnormal spaces.42 The vacuum phenomenon within a degenerated disk is represented on SE images as areas of signal void (Fig. 18-11).43 Although the presence of gas within the disk is usually suggestive of degenerative disease, spinal infection may (rarely) be accompanied by intradiscal or intraosseous gas.44 As intervertebral osteochondrosis progresses, there may be calcification of the disk. Calcification has usually been described on MRI as a region of decreased or absent signal intensity. The loss of signal is attributed to a low mobile proton density, as well as, in the case of gradient echo imaging, to its sensitivity to the heterogeneous magnetic susceptibility found in calcified tissue. There is, however, variability in the signal intensity of calcium with various sequences, and the type and concentration of calcification are important factors. Hyperintense disks on T1-weighted MRI may be secondary to calcification (Fig. 18-12).45 For concentrations of calcium particulates of up to 30% by weight, the signal intensity on standard T1-weighted images increases but then subsequently decreases.46,47 These data probably reflect particulate calcium reducing T1 relaxation times by a surface relaxation mechanism. Hyperintensities that are affected by fat suppression techniques have also been noted within intervertebral disks and are thought to be related to ossification with lipid marrow formation in severely degenerated or fused disks.
Degenerative Marrow Changes
Changes in signal intensity of the vertebral body marrow adjacent to the end plates of degenerated disks are a long recognized and common observation on MRI of the lumbar spine.48,49 However, despite a growing body of literature on this subject, their clinical importance and relationship to symptoms remain unclear.50
These marrow changes appear to take three main forms. Type I changes consist of decreased signal intensity on T1-weighted images and increased signal intensity on T2-weighted images (Fig. 18-13). They have been identified in approximately 4% of patients scanned for lumbar disease,49 in approximately 8% of patients after diskectomy,51 and in 40% to 50% of chymopapain-treated disks, which may be viewed as a model of acute disk degeneration.52 Histopathologic sections of disks with type I changes show disruption and fissuring of the end plate and vascularized fibrous tissue within the adjacent marrow that prolongs T1 and T2 times. Enhancement of type I vertebral body marrow changes is seen with the administration of gadolinium, and at times the changes extend to involve the disk itself and are presumably related to the vascularized fibrous tissue within the adjacent marrow. Type II changes are represented by increased signal intensity on T1-weighted images and isointense or slightly hyperintense signal on T2-weighted images (Fig. 18-14). They have been identified in approximately 16% of patients on MRI. Disks with type II changes also show evidence of end plate disruption, with yellow (lipid) marrow replacement in the adjacent vertebral body resulting in a shorter T1 time. Type III changes are represented by decreased signal intensity on both T1- and T2-weighted images and correlate with extensive bony sclerosis on plain radiographs. The lack of signal in type III changes no doubt reflects the relative absence of marrow in areas of advanced sclerosis (Fig. 18-15). Unlike types III, types I and II changes show no definite correlation with sclerosis on radiography.53 This is not surprising when one considers the histology; the sclerosis seen on plain radiographs is a reflection of dense woven bone within the vertebral body, whereas the MRI changes are more a reflection of the intervening marrow elements.
Similar marrow changes have also been noted in the pedicles (Fig. 18-16). Although originally described as being associated with spondylolysis, they have also been noted in patients with degenerative facet disease and pedicle fractures.54,55 The exact mechanism by which these marrow changes occur is not known. The association of these marrow changes with degenerative disk disease, facet changes, and pars and pedicle fractures suggest that they are a response to biomechanical stress.
Of these three types, type I changes appear to be more fluid and variable, a reflection of ongoing underlying pathologic processes such as continuing degeneration with associated changing biomechanical stresses. Of the three types, type I is most often associated with ongoing low back symptoms.56–60 In a longitudinal study, the incidence of new degenerative marrow changes was 6% over a 3-year period, with most being type I.58 In a study of nonoperated patients with low back pain, Mitra and associates found that 92% of type I changes converted either wholly or partially to type II (52%), became more extensive (40%), or remained unchanged (8%).57 There was an improvement in symptoms in patients in whom type I changes converted to type II.
Some diskography studies in patients with degenerative marrow changes have suggested that type I marrow changes are invariably associated with painful disks.61,62 Others have failed to reproduce this association,63,64 and thus the relationship between degenerative marrow changes and diskogenic pain remains unproved.
Multiple authors have observed a variety of inflammatory mediators in association with degenerative marrow changes. Burke and colleagues observed an increase in proinflammatory mediators such as interleukin-6, interleukin-8, and prostaglandin E2 in the disks of patients with type I marrow changes who were undergoing fusion for low back pain.64 Ohtori and coworkers found that the cartilaginous end plates of patients with type I marrow changes had more protein gene product (PGP) 9.5 immunoreactive nerve fibers and cells immunoreactive for tumor necrosis factor (TNF) than did patients with normal end plates.65 PGP 9.5 immunoreactivity was seen exclusively in patients with diskogenic low back pain. TNF immunoreactivity in end plates with type I marrow changes was higher than in those with type II marrow changes. The authors concluded that type I marrow changes represent a more active inflammation mediated by proinflammatory cytokines whereas type II and type III changes are more quiescent.50 In a study of infliximab, a monoclonal antibody against TNF-α, Korhonen and associates found that it was most effective when there were degenerative type I marrow changes at the symptomatic level.66 Nevertheless, the relationship of degenerative marrow changes to immunobiologic and cellular response mechanisms, although probably important, remains unclear.
In a study by Toyone and colleagues, 70% of patients with type I marrow changes had segmental hypermobility versus 16% with type II changes.56 Probably the greatest indication that these marrow changes, particularly type I, are related to biomechanical instability is based on observations after fusion. Chataigner and collaborators suggested that patients with type I marrow changes have much better outcomes with surgery than do those with isolated degenerative disk disease and normal or type II marrow changes.67 In addition, conversion of type I marrow changes to either normal or type II was associated with higher fusion rates and better outcomes. Other studies support the contention that persistence of type I changes after fusion suggests pseudarthrosis and is associated with persistent symptoms. Conversely, conversion of type I marrow changes to either normal or type II is associated with higher fusion rates and better outcomes (Fig. 18-17).68–70 The conclusion is that fusion produces greater stability, reduces biomechanical stress, and accelerates the course of type I marrow changes toward improvement.
As further support that these fluid marrow changes reflect biomechanical stress, we have seen similar marrow conversion in the pedicles of vertebral bodies associated with symptomatic pars and pedicle fractures, as well as in those with severe degenerative facet joint disease. Pedicle marrow change to a normal or type II appearance is associated with improvement in symptoms.71
Degenerative Facet and Ligamentous Changes
The superior articulating process of one vertebra is separated from the inferior articulating process of the vertebra above by a synovium-lined articulation, the zygapophyseal joint. Like all diarthrodial synovium-lined joints, the lumbar facet joints are predisposed to arthropathy with alterations of the articular cartilage (Fig. 18-18). With disk degeneration and loss of disk space height, the increased stress on the facet joints with craniocaudal subluxation results in arthrosis and osteophytosis. The superior articular facet is usually more substantially involved. Facet arthrosis can result in narrowing of the central canal, lateral recesses, and foramina and is an important component of lumbar stenosis. However, it has been proposed that facet arthrosis may occur independently and be a source of symptoms on its own.72,73 Synovial villi may become entrapped within the joint with resulting joint effusions. The mechanism of pain may be related to nerve root compression from degenerative changes of the facets or direct irritation of pain fibers from the innervated synovial linings and joint capsule.73 Osteophytosis and herniation of synovium through the facet joint capsule may result in synovial cysts, although the cause of these facet joint cysts is unclear. There is a more straightforward relationship of synovial cysts with osteoarthritis and instability of the facet joints than with degeneration of the intervertebral disk alone. In a review of patients with degenerative facet disease, synovial cysts occurred at anterior or intraspinal locations in 2.3% of patients and at posterior or extraspinal locations in 7.3% (Fig. 18-19).74 The important ligaments of the spine include the anterior longitudinal ligament, the posterior longitudinal ligament, the paired sets of ligamenta flava (connecting the laminae of adjacent vertebrae), the intertransverse ligaments (extending between transverse processes), and the unpaired supraspinous ligament (along the tips of the spinous processes). Because these ligaments normally provide stability, any alteration in the vertebral articulations can lead to ligamentous laxity with subsequent deterioration. Loss of elastic tissue, calcification and ossification, and bone proliferation at sites of ligamentous attachment to bone are recognized manifestations of such degeneration. Excessive lordosis or extensive disk space loss in the lumbar spine leads to close approximation and contact of the spinous processes and to degeneration of intervening ligaments.75,76 Histologically, granulomatous reaction and perivascular cellular infiltration characterize the condition.
Morphologic and Functional Sequelae
Instability
Segmental instability can result from degenerative changes involving the intervertebral disk, vertebral bodies, and facet joints that impair the usual pattern of spinal movement and produce motion that is irregular, excessive, or restricted. It can be translational or angular. Spondylolisthesis results when one vertebral body becomes displaced relative to the next most inferior vertebral body. The most common types are classified as degenerative, isthmic, iatrogenic, and traumatic. Degenerative spondylolisthesis is seen usually with an intact pars interarticularis, is related primarily to degenerative changes of the apophyseal joints, and is most common at the L4-5 vertebral level (Fig. 18-20). The predilection for degenerative spondylolisthesis at that level is thought to be related to the more sagittal orientation of the facet joints, which makes them increasingly prone to anterior displacement. Degenerative disk disease may predispose to or exacerbate this condition secondary to narrowing of the disk space, which can produce subsequent malalignment of the articular processes and lead to rostrocaudal subluxation.
Herniation
Herniation refers to localized displacement of nucleus, cartilage, fragmented apophyseal bone, or fragmented annular tissue beyond the intervertebral disk space. The disk space is defined rostrally and caudally by the vertebral body end plates and peripherally by the outer edges of the vertebral ring apophyses, exclusive of osteophytic formations. The term localized contrasts with the term generalized, the latter being arbitrarily defined as greater than 50% (180 degrees) of the periphery of the disk.77 Displacement, therefore, can occur only in association with disruption of the normal annulus or, as in the case of intravertebral herniation (Schmorl’s node), a break in the vertebral body end plate. Because details of the integrity of the annulus are often unknown, the diagnosis of herniation is usually made by observation of localized displacement of disk material beyond the edges of the ring apophyses—that is, less than 50% (180 degrees) of the circumference of the disk (Fig. 18-21). Localized displacement in the axial (horizontal) plane can be focal, or less than 25% of the disk circumference, or broad based, between 25% and 50% of the disk circumference. The presence of disk tissue circumferentially (50% to 100%) beyond the edges of the ring apophyses may be called bulging and is not considered a form of herniation. A disk may have more than one herniation. The term herniated disk does not imply any knowledge of etiology, relationship to symptoms, prognosis, or need for treatment. When data are sufficient to make the distinction, a herniated disk may be more specifically characterized as protruded or extruded. These distinctions are based on the shape of the displaced material. Protrusion is present if the greatest distance, in any plane, between the edges of the disk material beyond the disk space is less than the distance between the edges of the base in the same plane (Fig. 18-22). Extrusion is present when, in at least one plane, any one distance between the edges of the disk material beyond the disk space is greater than the distance between the edges of the base in the same plane or when no continuity exists between the disk material beyond the disk space and that within the disk space (Fig. 18-23). Extrusion may be further specified as sequestration if the displaced disk material has completely lost any continuity with the parent disk. The term migration may be used to signify displacement of disk material away from the site of extrusion, regardless of whether it is sequestrated (Fig. 18-24). Herniated disks in the craniocaudal (vertical) direction through a break in the vertebral body end plate are referred to as intravertebral herniations. Nonacute Schmorl’s node intrabody herniations are common spinal abnormalities regarded as incidental observations. They have been reported in 38% to 75% of the population.78,79 Although intrabody herniations may occur as a result of end plate weakness secondary to bone dysplasia, neoplasms, infections, or any process that weakens the end plate or the underlying bone, most intrabody herniations probably form after axial-loading trauma, with preferential extrusion of nuclear material through the vertebral end plate rather than an intact and normal annulus fibrosus. It has been suggested that asymptomatic intrabody herniations may be traceable to a specific occurrence of acute nonradiating low back pain in the patient’s history, which supports the concept that intrabody herniations (Schmorl’s nodes) occur through sites of end plate fracture. Type I vertebral body marrow changes have been described surrounding acute interbody herniations.80
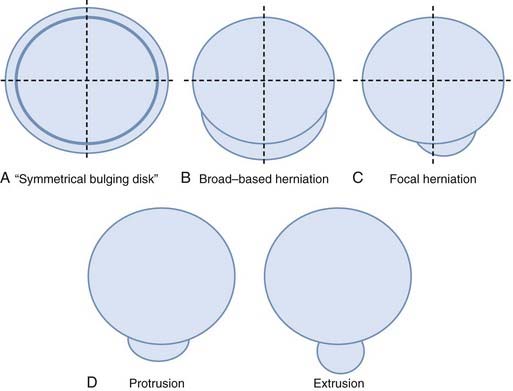
FIGURE 18-21 A, Symmetrical bulging disk. B, Broad-based herniation. C, Focal herniation. D, Protrusion and extrusion.
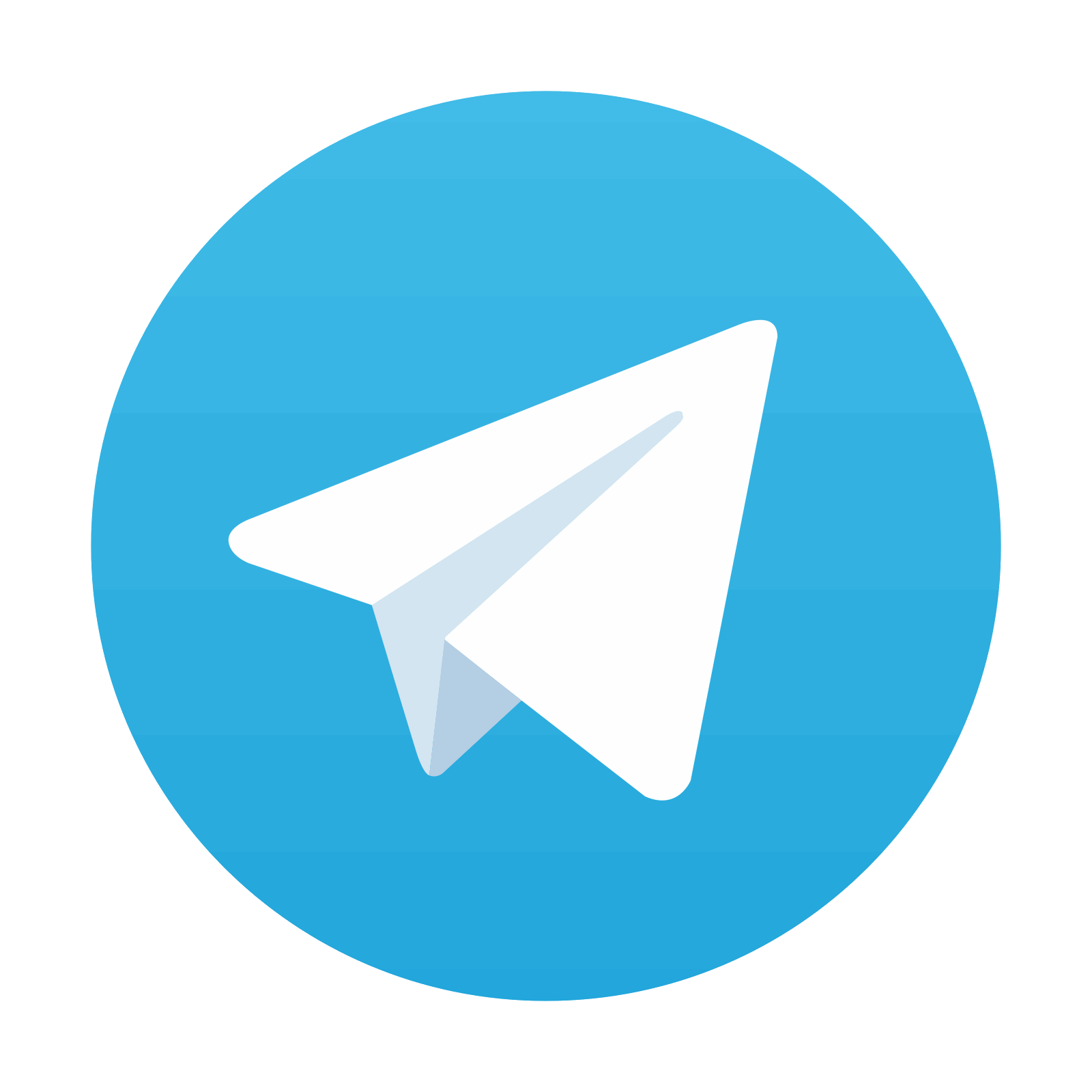
Stay updated, free articles. Join our Telegram channel

Full access? Get Clinical Tree
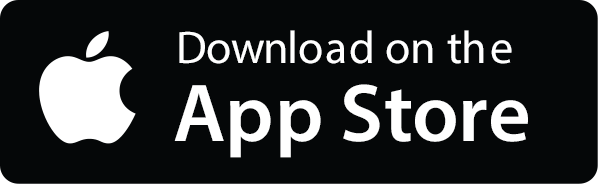
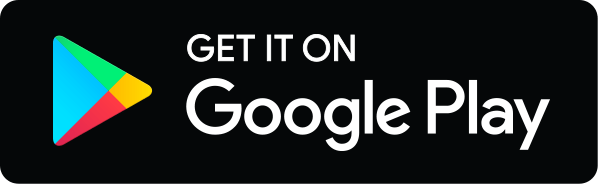