CHAPTER 261 Radiosurgery for Functional Disorders
The origin of stereotactic radiosurgery parallels the developmental history of functional neurosurgery. Leksell initially conceived the idea of closed-skull, single-session irradiation of a precisely defined intracranial target in 1951. He applied this concept immediately to functional neurosurgery.1 At that time, functional destruction of normal brain required thermal energy or injection of a chemical such as phenol. Leksell cross-fired photon or proton radiation beams to achieve a similar goal. The initial radiosurgical concept was to create a small, precisely defined focal lesion that was defined by image guidance. Contrast-enhanced encephalography provided relative identification of such targets. Even though the ganglionic portion of the trigeminal nerve could be indirectly located with plain radiographs or cisternograms, deep brain targets required air or positive contrast-enhanced ventriculography; direct visualization of the target for functional radiosurgery required the later development of computed imaging technology. Thus, radiosurgical techniques were used to create image-guided physiologic inactivity or focally destructive brain lesions without neurophysiologic guidance. This concept was controversial, and the lack of neurophysiologic guidance remained the greatest argument against the use of radiosurgery for selected disorders. Nevertheless, the use of radiosurgery as a “lesion generator” is based on extensive animal studies that defined the dose, volume, and temporal response of the irradiated tissue. The utility of radiosurgery has now been compared with that of the microsurgical, percutaneous, and electrode-based techniques used for functional neurologic disorders. Current anatomic targets include the trigeminal nerve (for trigeminal neuralgia), the thalamus (for tremor or pain), the cingulate gyrus or anterior internal capsule (for pain or psychiatric illness), the hypothalamus (for cancer pain), and the hippocampus (for epilepsy).2,3 Current indications and expected outcomes after radiosurgery are discussed in this chapter.
Leksell first coupled an orthovoltage x-ray tube to his early-generation stereotactic frame.1 Later, he worked with physicist Borje Larsson to cross-fire proton beams5 and subsequently used a modified linear accelerator. His decision to build the first Gamma Knife in 1967 reflected his frustration with particle beam technology, which required that the patient travel to a special cyclotron center. The first Gamma Knife collimator helmets created a discoid volume of focal irradiation that could “section” white matter tracts or brain tissue in a manner similar to a leukotome or other instrument. Later models of the Gamma Knife have provided more flexibility in the creation of lesions or effects of different volumes, together with precise robotic capability.
The Early Years of Functional Radiosurgery
Before 1978, all uses of radiosurgery remained limited because of the lack of high-resolution neuroimaging techniques to identify brain lesions or functional brain regions. Angiographic targeting of arteriovenous malformations proved successful but was limited by the two-dimensional estimates of complex three-dimensional target volumes. Functional radiosurgery was performed for a limited number of patients with intractable pain related to malignancy,6,7 movement disorders,8 psychiatric dysfunction,9,10 and trigeminal neuralgia.4 On the sidelines, percutaneous retrogasserian glycerol rhizotomy was developed from an observation made during refinement of the Gamma Knife technique for trigeminal neuralgia. After injection of the glycerol to localize the trigeminal nerve before radiosurgery, the trigeminal neuralgia pain was relieved.
For intractable pain related to malignancy, radiosurgery was used both for hypophysectomy and for medial thalamotomy. Although the procedure was noninvasive, the latency interval for lesion generation and pain relief was one obvious drawback. Steiner and colleagues presented results from an autopsy study after radiosurgery for cancer pain in 1980.7 The ablative dose for tissue volumes had been estimated during animal experiments through the 1960s involving the use of protons and photons.5,11,12 The initial patients who underwent radiosurgery for tissue ablation received maximum doses of 100 to 250 Gy. With small volumes, doses in excess of 150 Gy provided consistent tissue necrosis in animal models. Because these first patients were treated for pain from a terminal malignancy, they did not live long enough to sustain a potential complication from such high doses. The clinical use of such doses proved to be the foundation for later use in tremor management.
Dose Selection for Parenchymal Functional Radiosurgery
Early animal experiments showed consistent creation of lesions with doses at or higher than 150 Gy.11,12 Clinical data showed that pain relief usually occurred within 3 weeks after radiosurgery.7 In rat experiments at 200 Gy with a single 4-mm isocenter, we found a consistent relationship for lesion generation that substantiated observations from that human study.13 Doses of 200 Gy were delivered to the rat frontal brain, and then the brain was studied at 1, 7, 14, 21, 60, and 90 days after irradiation. At 1 and 7 days, the brain continued to appear normal. By 14 days, the parenchyma appeared slightly edematous within the target volume. However, by 21 days, a completely circumscribed volume of necrosis was identified within the radiation volume (4-mm diameter). This remained consistent thereafter. Thus, the clinical observation of pain relief at 21 days noted by Steiner and colleagues was correlated with laboratory findings at the 200-Gy dose.
An ablative radiosurgical lesion appears as a punched-out, circumscribed volume of complete parenchymal necrosis with cavitation. Within a 1- to 3-mm rim that characterizes the steep falloff in radiation dose, normalization of tissue appearance is found. In this zone, blood vessels appear thickened and hyalinized, and protein extravasation can often be identified. The brain is edematous in this region, either from an increase in extracellular fluid or from intracellular swelling secondary to gliosis. Acute or chronic inflammatory cells are present. Magnetic resonance imaging (MRI) demonstrates all these features after radiosurgical thalamotomy—a sharply defined, contrast-enhanced rim that defines the low-signal lesion (on short TR images) surrounded by a zone of high-signal brain tissue (on long TR images).14 Friehs and colleagues collected imaging data from four centers that created functional radiosurgical lesions (N = 56). They found that maximum doses in excess of 160 Gy were more likely to produce lesions larger than expected and recommended single 4-mm-isocenter lesions at doses below 160 Gy.15
Studies at the University of Pittsburgh found that in both large- and small-animal models, doses of 100 Gy or higher caused necrosis but the delay until necrosis was longer.13,16,17 To identify the effect of increasing volume, we used an 8-mm collimator in a baboon model and found that an 8-mm-diameter necrotic lesion developed in half the animals at doses as low as 50 Gy.18 Dose, volume, and time are the three key factors that determine the nature of the functional ablative lesion. Once created, this lesion remains stable for years.11
The limitation of radiosurgical technology as a lesion generator stems from the inability to reliably control the effects of dose and volume. When a larger brain target may be desirable, the sharp falloff in dose outside the target becomes less steep with increasing volume. The risk for an adverse radiation effect outside the target volume becomes problematic.19 At small volumes (i.e., single 4-mm collimator), the radiosurgery-created lesion appears more consistent.
Imaging in Functional Surgery
Because physiologic information is excluded from the targeting component of a functional radiosurgical procedure, high-quality stereotactic neuroimaging must be performed. The imaging must be accurate because small volumes are irradiated. In addition, the imaging must have sufficient resolution to identify the target structure but also show important regional tissues. MRI is the preferred imaging tool for functional radiosurgery.20–23
Accurate stereotactic MRI-based localization should be confirmed at each institution.24 The use of fast inversion recovery or other long–relaxation time MRI sequences helps separate gray and white matter structures. However, to this day, targeting of physiologically abnormal brain regions such as groups of kinesthetic thalamic tremor cells or epileptic foci with imaging alone remains indirect.
Trigeminal Neuralgia
Leksell first used radiosurgical techniques for trigeminal nerve irradiation in 1953.4 He later stated that, “from these observations no definite conclusion should be drawn concerning the optimal dose of radiation or the exact mechanism in site of action in the route or ganglion, or even the general applicability of the method.”4 In 1983, Leksell reported that 63 patients had undergone Gamma Knife radiosurgery for trigeminal neuralgia. The use of gasserian ganglion radiosurgery was reported by Lindquist and coauthors (46 patients) and Rand and associates (12 patients), but inconsistent results were obtained.2,25 These authors concluded that the ganglion was probably not appropriate as the primary radiosurgical target.
Initially, the Pittsburgh group sought to reevaluate the early anecdotal success of trigeminal neuralgia radiosurgery reported by Leksell. They reported a multicenter study in which Gamma Knife radiosurgery was used for trigeminal nerve radiosurgery.21 Targeting the nerve at this location was suggested by Hakänsson and Lindquist as one potential way to improve outcomes. At this location, the nerve could be imaged with high-resolution MRI and was suitable for radiosurgical targeting with a 4-mm collimator (Fig. 261-1). This location offered a great advantage over ganglion irradiation in Meckel’s cave, where the nerve proper could not be imaged readily. In an initial multicenter 50-patient study with 18-month median follow-up, 58% became pain free, 36% had significant improvement (50% to 90% relief), and 6% failed.21 Patients with significant improvement were satisfied, especially in view of the fact that in most patients the pain had been recurrent after multiple previous surgeries. A maximum radiosurgical dose higher than 70 Gy (70 to 90 Gy) was associated with a greater chance of complete pain relief than was 60 or 65 Gy (P = .0003). After publication of this study, centers worldwide have performed trigeminal neuralgia radiosurgery, and there are many reports that have documented the results.
Radiosurgery Technique for Trigeminal Neuralgia
Stereotactic radiosurgery is performed under local anesthesia. High-resolution trigeminal nerve imaging is necessary for accurate radiosurgical targeting. After a sagittal scout sequence, spoiled GRASS (gradient recalled acquisition in a steady state) gradient recombinant (SPGR) axial volume acquisition sequences using 512 × 512 matrices are targeted to the pons and midbrain level. They are divided into 1-mm image slices to provide graphic depiction of the trigeminal nerve (Fig. 261-1). The nerve can be identified in its course from the brainstem to Meckel’s cave. Some patients who have previously undergone surgery (microvascular decompression or percutaneous surgery) present greater imaging challenges because the nerve may be more difficult to identify (as a result of either nerve atrophy or regional fibrosis). In such patients long TR MRI sequences are added to contrast the nerve against the high-signal background of cerebrospinal fluid. An initial concept was to place a 4-mm isocenter 2 to 4 mm anterior to the junction of the trigeminal nerve and pons so that the brainstem surface was usually irradiated at no more than the 30% isodose. Other centers chose a midnerve or anterior nerve target. Computed tomography–based targeting can be performed, with or without contrast-enhanced cisternography, in patients who cannot undergo MRI. The range of the maximum radiosurgical dose is typically 75 to 90 Gy.26 De Salles and coworkers used a modified linear accelerator to irradiate the trigeminal nerve in patients with neuropathic pain related to skull base cancer and for trigeminal neuralgia.27
Clinical Response
Improvement after radiosurgery has been defined as a reduction in both the frequency and severity of trigeminal neuralgia attacks. Although some physicians believe that only total pain relief with no further need for medication should be considered management success, many of our patients believe otherwise. Because so many patients have failed other treatments, many were grateful for any significant relief even if occasional pain persisted. Numerous studies have reported outcomes after radiosurgery for trigeminal neuralgia; however, the definition of improvement in pain is not uniform.8,21,28–33,35–41 Pain relief (according to the patient) can be generally coded into three categories, including poor response or treatment failure (<50% pain relief), good pain response (>50% pain relief), and complete pain relief with or without medications.
The maximum level of pain relief is typically achieved within 1 month after radiosurgery.21,38,40,42–46 Disappearance of the trigger areas or frank pain relief occurs within 24 hours of treatment in up to a third of treated patients.8 Complete pain relief within 1 week of treatment is reported in over 40% of eventual responders.46 More than three quarters of partial and complete responders will have responded within 3 months of treatment and more than 90% of responders by 6 months.43 Kondziolka and coauthors reported in their multi-institutional studies that initial improvement in trigeminal neuralgia was noted in 91 patients (86%).21,26 There were 64 patients who had complete pain relief without medication, 18 who had a good pain response, 9 who were slightly improved, and 15 with no improvement. At last follow-up, significant pain relief was noted by 77% of patients (good response plus complete pain relief). Young and associates reported a 74% rate of complete pain relief with cessation of medical therapy in their 60-patient series, with better results seen in patients with no previous surgical history.47
However, approximately 15% of patients do not experience greater than 50% pain relief. If treatment failure is defined as failure to obtain complete pain relief, the failure rate is doubled.38,46,48,49 We believe that it is safe to consider the treatment a failure if no response has taken place after 3 to 6 months.8 In addition, recurrences are most common in the first year. Rates of recurrence ranged from 5% to 42% in the studies.21,28,31,35–38,40,42,43,49,78 Kondziolka and coauthors reported that relapse of pain was noted in just 6 of 64 patients who attained complete relief (10%) and that this relapse occurred within 10 months after the onset of complete relief.21 Maesawa and colleagues stated that 71% of patients achieved or maintained complete or greater than 50% relief at 2 years and 56% maintained the relief 5 years after initial response; the rate of achieving and maintaining complete pain relief was 59% at 2 years and 38% at 5 years.43 The number of patients with good or complete pain relief has decreased over years, indicative of some lessening of the effect over time. More series with extended follow-up past 5 years are required to study this issue more definitively.
Prognostic Factors
Different investigators have tried to determine specific patient factors related to relief of pain. The absence of previous surgery was suggested as a significant factor associated with achieving and maintaining complete pain relief.38,39,43 However, one study found no differences with respect to previous surgery.51 Atypical features of pain may be associated with a poorer response to treatment and a greater probability of pain recurrence.40,43,46 Rogers and associates reported complete pain relief rates of 49% in patients with typical pain and 9% in patients with atypical features of pain.40 However, Pollock and colleagues did not find any differences in these groups, but the development of new facial sensory loss was found to correlate with sustained complete pain relief in their study.38 In addition, they found two factors at or near statistical significance for achieving and maintaining an excellent outcome: younger patient age and longer length of the irradiated portion of the nerve; however, Régis and coworkers noted that the probability of being pain free was lower in patients younger than 60 years (55.7% versus 90.9%, P = .01).78 To date, increasing the radiosurgical dose from 70 to 90 Gy or the number of lesions (from one to two isocenters) has not produced a definite improvement in pain outcomes, although there is a trend to more relief with higher doses. Nonetheless, a higher dose or increase in the volume of irradiated nerve has led to an increase in the rate of sensory complications.
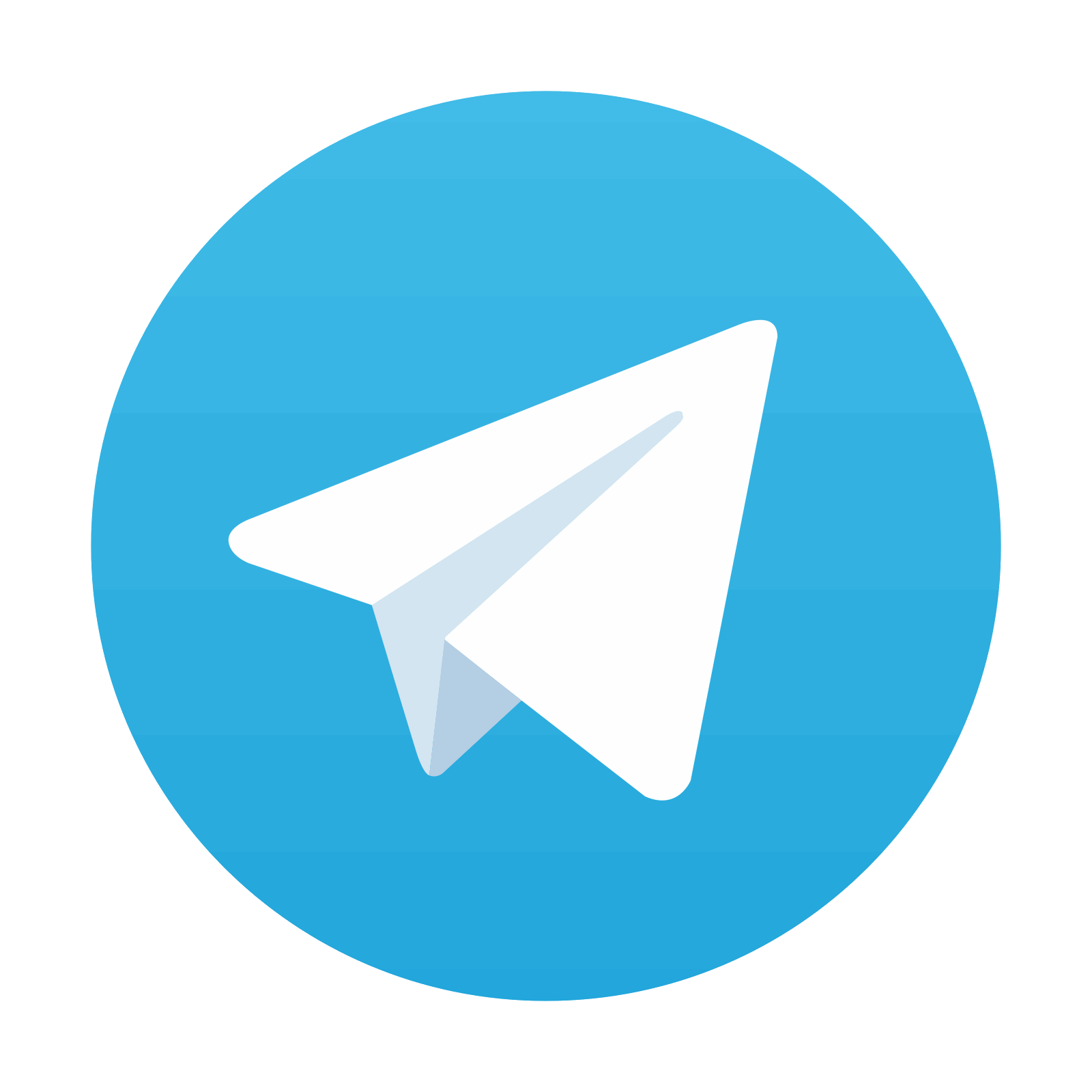
Stay updated, free articles. Join our Telegram channel

Full access? Get Clinical Tree
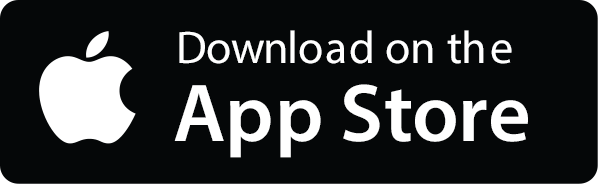
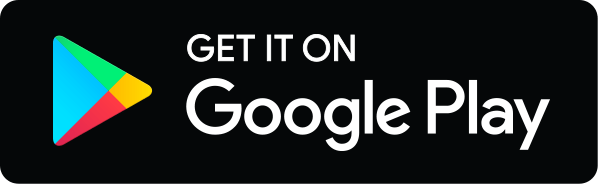