CHAPTER 259 Radiosurgery of Benign Intracranial Tumors
Radiosurgery has become one of the primary modalities used for the management of intracranial tumors.1–3 The extensive radiosurgical experience that has developed worldwide over the years has allowed refinement of technique and a better understanding of radiobiologic concepts.4–7 Additionally, improvement in patient selection, advances in magnetic resonance imaging (MRI) technology, and access to powerful computer-based navigation systems have led to very significant improvements in clinical radiosurgical results. Globally, radiosurgery has revolutionized neurosurgery by providing neurosurgeons with a novel approach that allows safe and efficient treatment of small, deeply seated lesions that would normally be associated with a high risk for functional deterioration with microsurgery. The remarkable safety-efficacy ratio of radiosurgery is especially valuable for patients with benign intracranial lesions that may not have an impact on life expectancy.
History
After its creation by Leksell in the 1950s and introduction of the Gamma Knife in the 1970s, radiosurgery was initially dedicated to functional neurosurgery.8,9 However, with the discovery of new drugs effective in the treatment of functional disorders and with its proven efficacy in treating the nidus of arteriovascular malformations (AVMs), radiosurgery was used mainly for the treatment of AVMs. Even though the first vestibular schwannoma (VS) was treated in 1968 by Leksell and Noren,10 it was not until the 1980s that sufficiently accurate neuroradiologic targeting devices were available to allow the development of Gamma Knife surgery (GKS) as a modern, high-accuracy, image-guided surgical option for skull base surgery. In the 1980s, VSs and meningiomas became prominent indications for radiosurgery.
Since 1960, roughly 400,000 patients have been treated worldwide with GKS. Thus, GKS is no longer an experimental method, and sufficient evidence is available for us to draw a precise picture of the potential role of radiosurgery in the neurosurgical management of benign intracranial tumors.3
Definition
Radiosurgery is a neurosurgical procedure that uses convergent narrow ionizing beams, delivered in a single session with stereotactic accuracy and precision, to destroy or modify a biologically predefined target without injuring critical surrounding structures.11 Radiotherapy was already being used for the treatment of intracranial tumors when Leksell developed the concept of radiosurgery in the 1950s. Although ionizing radiation is used for both conventional radiotherapy and radiosurgery, it is the physical properties inherent in each technique that make the radiobiologic effects of these two methods dramatically different. Thus, the clinical effects, radiologic effects, indications, risks, complications, and requirements for use will be very much different for both techniques.
Theoretically, radiotherapy is poorly adapted to the treatment of brain lesions because of the ordinarily high level of radioresistance of these tumors and the high sensitivity to radiation of precious neural structures. Radiotherapy, when delivered by fractionation, attempts to attenuate this effect through biologic selectivity. To adhere to the philosophy of treating intracranial lesions while sparing and avoiding any form of physical aggression involving normal neurological structures, neurosurgeons developed GKS, a topologic selectivity instrument that delivers the vast majority of the energy to the target and very little to the surrounding structures.11
Radiobiology
Radiobiology is teaching us that with a single dose, the radiobiologic effect on the targeted structure is much more important than when the same total dose is spread over several fractions. Calculation of the biologic equivalent dose of radiosurgery to determine what dose should be used instead of fractionated treatment (e.g., 2 Gy per fraction) for the same radiobiologic effect11 requires that the nature of the targeted tissue be taken into account (linear quadratic formula). Such calculation indicates that there is a strong radiobiologic benefit of using radiosurgery instead of radiotherapy when treating benign intracranial tumors (slow-reacting tissue). This is also a demonstration that the radiobiology of radiosurgery and radiotherapy is extremely different.11 Craniopharyngiomas are among the tumors traditionally requiring high radiotherapy doses because of their well-known radioresistance, in contrast to their high radiosensitivity to radiosurgery, which allows safe and effective radiosurgical treatment of small residual craniopharyngiomas even when they are very close to the optic pathways.
Long-Term Complications
Long-term complications must be scrutinized because of the extensive use of stereotactic radiosurgery (SRS) for many benign tumors in young patients over the past 2 decades. The potential long-term carcinogenic risk associated with SRS was not evaluated until recently. The definition of radioinduced tumors is based on the following criteria proposed by Cahan and colleagues: the tumor must occur in a previously irradiated field after a long interval from the time of irradiation and must be pathologically different from the primary tumor and not present at the time of irradiation.12 In addition, the patient must not have a genetic predisposition for the tumor. A low dose of radiation, such as 1 Gy, has been associated with second tumor formation at a relative risk of 1.57 to 8.75. This relative risk increases to 18.4 for an interval of time between 20 and 25 years. The radiation-associated incidence of tumor is linked to different factors such as age and individual genetic susceptibility. At this time, three radiation-associated gliomas and five malignant VSs have been reported in the literature. Moreover, these second tumors do not meet all the criteria of Cahan and coworkers.13 Long-term follow-up ranging from 5 to 30 years is needed to observe the crude incidence of radiation-induced tumors. The relative risk is estimated at less than 1 per 1000 and must be reported to each patient before any radiosurgical procedure.13
Vestibular Schwannomas
Gamma Knife SRS was first used in 1969 by Leksell to treat VS.10 Lunsford’s group has established the optimal treatment parameters for control of VSs in conjunction with preservation of hearing and facial nerve function. Lunsford and associates have confirmed the importance of Noren’s policy of administering the “lowest irradiation doses that are therapeutically effective.”14 They demonstrated the impact of technical advances on the improvement in clinical results.15 Flickinger and colleagues recently reviewed their series of 313 patients with previously untreated unilateral VS who underwent SRS (a marginal dose of 12 to 13 Gy) between 1991 and 2001.16 The actuarial 6-year resection-free tumor control rate was 98.6%. The 6-year actuarial rates for preservation of facial nerve function, trigeminal nerve function, and hearing were 100%, 95.6 ± 1.8%, and 78.6 ± 5.1%, respectively. Using outcomes analysis and retrospective review, Lunsford’s team suggested an advantage (in terms of functional outcome) of SRS over microsurgery in the management of VS.17
In the past 3 decades, microsurgery and SRS have become well-established management options for VS. The Marseille SRS experience includes 2000 patients, with more than 1000 patients having follow-up longer than 3 years.18 A long-term tumor control rate of 97%, an incidence of transient facial palsy of lower than 1%, and a probability of preservation of functional hearing of between 50% and 95% were achieved in this large series of patients treated by state-of-the-art SRS (Fig. 259-1). Between 1973 and 2004, a total of 2577 VSs were surgically resected or treated with SRS by the Marseille group. Surgical resections were performed from the translabyrinthine, middle fossa, and retrosigmoid/suboccipital approaches. Approximately 1500 patients have been treated with Gamma Knife SRS, and all were evaluated prospectively. All patients with VS treated by SRS at Marseille receive a 12-Gy dose to the tumor margin. Three major technical advances have clearly influenced this practice. The availability of high-resolution stereotactic MRI,14 workstations for selecting the dose and treatment plan (Gamma Plan),19 and installation of the robotic Automatic Positioning System (APS) have allowed our group to achieve more conformal and selective dose planning. The average number of isocenters used in 1992 was less than 5 but increased to more than 15 in 2003 with the APS system. Consequently, if we consider the first 100 patients to represent the learning curve, four treatment periods can be defined. The rate of transient facial palsy and hemifacial spasm in patients with VS treated by our group was 3% and 3% during the first phase (June 1992 to December 1994, 100 patients), 1.4% and 2.8% during the second period (December 1994 to July 1997, 212 patients), 0.55% and 0.83% during the third period (July 1997 to May 2000, 360 patients), and 0% and 0% during the last period (May 2000 to January 2002, 258 patients), respectively.18
Comparison to Microsurgery
In a comparison of 110 VSs resected surgically and 97 treated by SRS, a lower rate of facial palsy and a higher probability of preservation of functional hearing were both achieved after SRS.20 All patients had Koos stage II-III tumors with a minimum follow-up of 4 years. (Koos grading is as follows21: stage I, small intracanalicular tumor; stage II, small tumor with protrusion into the cerebellopontine angle and no contact with the brainstem; stage III, tumor occupying the cerebellopontine cistern with no brainstem displacement; and stage IV, large tumor with brainstem and cranial nerve displacement.) The three other studies in which the safety and efficacy of microsurgery and radiosurgery were compared are consistent with these results.17,18,22
Efficacy of Radiosurgery
To better define the accuracy and efficacy of SRS, the imaging-determined morphologic changes of 1000 tumors treated between July 1992 and January 200223 by the otoneurosurgical group of the Timone Hospital in Marseille were evaluated systematically. Evaluation with MRI before and after SRS (intervals of 6 months and 1, 2, 3, 5, 7, and 10 years) was reviewed. Systematic measurements have been performed on all tumors treated. Preoperatively, 129 patients had progressive tumors. At the time of SRS, the median tumor volume was 732 mm3 (mean, 1346; range, 20 to 14,405). According to the Koos topographic classification, among the 996 patients with complete follow-up, there were 80 stage I, 538 stage II, 322 stage III, and 56 stage IV tumors. Loss of central enhancement was noted on MRI at 6 months and/or 1 year postoperatively in 45.5% of patients. In 64% of these patients, loss of central contrast enhancement occurred. A significant increase in tumor size was recorded in 15% of the patients (Figs. 259-2 and 259-3). In 3% of the patients, progression led to a second procedure, either resection or repeated SRS. Failure is defined as continuous tumor progression 3 years after SRS.18 Tumor control was achieved in 97% of the patients. Because the natural history of VS includes growth of 2 mm/yr, these results confirm the efficacy of SRS.
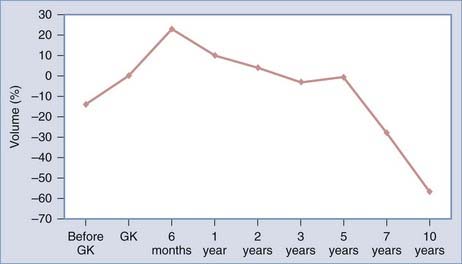
FIGURE 259-2 Overall evolution of the volume of treated vestibular schwannomas. GK, Gamma Knife radiosurgery.
Hearing Preservation
In the SRS experience of the Marseille group, 175 patients with VS and functional preoperative hearing (Gardner-Robertson stage 1 or 2) were initially treated with SRS and have a follow-up of longer than 3 years.23 Hearing was preserved in 60% of all patients after SRS. Univariate and multivariate analysis has revealed parameters that influence the probability of preservation of functional hearing at 3 years, including limited hearing loss (Gardner-Robertson stage 1), the presence of tinnitus, younger age, and small lesion size. The rate of preservation of functional hearing at 3 years was 77.8% in patients with stage 1 hearing, 80% in patients with tinnitus as a first symptom, and 95% when the patient had both stage 1 hearing and tinnitus. In these patients, the probability of preservation of functional hearing at 5 years was 84%.24
Facial Nerve Preservation
Facial nerve palsy is very rare (<1%) in the series from the three centers with the largest experience (Marseille, Pittsburgh, and Rhode Island).18 Schwannomas originating from the facial nerve itself are more inclined to interfere with facial motor function. Because of the paucity of facial palsy after SRS for VS, the use of SRS may be reasonable in this difficult group of patients.25 Among the 1000 schwannomas of the cerebellopontine angle treated by SRS in Marseille between July 1992 and March 2003, 9 were diagnosed as originating from cranial nerve VII. The criterion for diagnosis was involvement of the second or third portion of cranial nerve VII (seven patients) or intraoperative demonstration during a previous surgical resection (two patients). Facial palsy occurring within 18 months of radiosurgery for VS was determined in patients with more than 2 years of follow-up (eight patients). Four patients had previous facial paresis. Normal facial motor function was observed in two patients before SRS (House-Brackmann grade 2 in six patients and grade 3 in one patient). Follow-up ranged from 2 to 7 years in all patients. Worsened facial palsy did not occur in any patient, whereas two patients had improvement in their preoperative facial palsy. Our results and those from the main series in the literature confirm the important role of SRS in the management of VS and preservation of facial nerve function (Table 259-1).26–36
The best candidates for radiosurgery are young patients with small and medium VSs and few symptoms. Patients with Koos stage II and III tumors are good candidates as well. In addition, patients with intracanalicular, cystic, previously resected, and Koos stage IV tumors may be candidates under certain conditions. Originally, until 1999, Koos stage I tumors were considered for radiosurgery at numerous institutions only in patients with tumor progression. Retrospective analysis of the tumor’s growth rate and preservation of functional hearing and requests for radiosurgery by patients have led to modification of this practice. According to the Marseille group, patients treated with SRS have a higher probability of preservation of functional hearing.37 Consequently, patients with a stage I lesion and functional hearing may be considered for radiosurgery in the absence of tumor progression.
Large Vestibular Schwannomas: Combined Microsurgical and Radiosurgical Treatment
To reduce the incidence of this unacceptable complication, several centers have adopted a combined approach in which optimal subtotal microsurgical resection is followed by radiosurgical treatment of the residual tumor.38–40 In our own otoneurosurgical group, a series of 51 patients (16 males, 35 females) harboring LVSs were managed between January 2003 and January 2008 with an intentional combined approach. The initial symptoms were hearing deterioration, imbalance, ataxia, and hydrocephalus. Our treatment strategy was to perform optimal resection of the tumor with preservation of facial nerve integrity. Radiologically, 51 patients had Koos stage IV LVSs.21 The median tumor diameter in the cerebellopontine angle was 30 mm (range, 25 to 35 mm). Microsurgically, a widened translabyrinthine approach was used in 38 patients, whereas 13 underwent a retrosigmoid transmeatal approach. Postoperative evaluation of patient status and facial nerve function was determined at 3 and 6 months and 1, 2, 3, 5, 7, and 9 years after surgery, including assessment of the intermedius nerve (the smaller root of cranial nerve VII). Three months after surgery, gadolinium-enhanced MRI of the posterior fossa was performed to assess the size of the tumor remnant.
The need for radiosurgical treatment of the remnant tumor was determined postoperatively and depended on the size of the tumor remnant and facial nerve status. In patients with grade I and II postoperative facial motion, radiosurgery was performed in the 3- to 6-month period after surgery (Fig. 259-4). However, GKS was postponed to 9 to 12 months if facial nerve motion was worse than House-Brackmann grade 2 because recovery could reasonably be expected. Total removal was achieved in 9 patients, nearly total removal (>95% tumor resection) in 7 patients, subtotal removal (95% to 80% tumor resection) in 34 patients, and partial removal (<80% tumor resection) in 1 patient. Postoperative facial nerve preservation (House-Brackmann grade 1 or 2) was achieved in 66% of the patients immediately and in 82.2% at last follow-up, as shown in Table 259-1. The functional status of the intermedius nerve was able to be assessed in 39 patients at longer than 6 months after surgery. The nerve was normal in 20 patients, partially impaired in 13, and totally deficient in 6. Twenty-four patients underwent GKS at a 4- to 13-month interval after subtotal resection of their tumor. Thus far, no complications have been observed after radiosurgery, even with particular attention directed to facial and the intermedius nerve status, which remained unchanged. The median radiologic and clinical follow-up after microsurgery was 24 months (range, 8 to 68 months). Permanent tumor control was achieved in all patients with no need to reoperate or offer another GKS procedure.
Justification of the necessity for additional radiosurgery in the event of a tumor remnant is an important issue. In actuality, we have few data concerning the fate of conservatively treated residual tumor. Before the modern era of neuroimaging, reports indicated a 40% rate of clinical regrowth after incomplete surgery.41 W. F. House had to perform reoperations on 10 of 22 patients 4 to 7 years after initial partial resection, with increased morbidity.42 More recently, Silverstein reported a 20% rate of regrowth in 15 patients older than 65 years who initially underwent subtotal resection.43 In studies in which computed tomography (CT) or MRI was available, rates of regrowth and reoperation after incomplete removal were 40% and 20%, respectively.44 Therefore, we consider it warrented to offer adjunctive GKS when a clear target is identified on postoperative MRI. This proactive strategy is also justified by the necessity of reoperating on patients with a high risk for damage to the facial nerve.45
Meningiomas
First-line radiosurgery is recommended for meningiomas in the cavernous sinus when they are small enough and sufficiently far from the optic pathways.46 When the lesion is too big or too close to the optic pathway, a combined approach (resection of the portion of the lesion out of the cavernous sinus) or conventional radiotherapy is advocated (Fig. 259-5).47,48
Petroclival meningiomas may be an excellent indication for radiosurgery, especially when they are growing, causing few clinical signs, and small enough that they can be treated with lower morbidity than possible with microsurgery.49
Parasagittal meningiomas have been demonstrated to correlate with the highest rate of brain edema, especially in patients previously operated on or with a neurological deficit at the time of radiosurgery.50
Rational for Radiosurgery
Meningiomas account for about 20% of intracranial tumors, with an incidence of 1 to 6 per 100,000 persons. Surgical resection is historically the treatment of reference. In many instances, meningiomas cannot be completely excised as a result of unacceptable risks of morbidity because of their crucial neurovascular environment. Analysis of the modern literature indicates that radical microsurgical resection of skull base meningiomas is achieved in 50% to 60% of patients, with permanent morbidity rates reaching 20%, a mortality rate of 5% to 15%,51,52 and a recurrence rate of 10% at 10 years after surgery.53 Additionally, because 90% to 95% of diagnosed meningiomas are benign (World Health Organization [WHO] grade I), preservation of quality of life together with control of tumor growth should be the major goals of treatment of this select group of meningiomas. In this scenario, SRS has become an effective alternative to microsurgery.
Patient Selection
A selective review of published series is outlined in Table 259-2.54–60 The target can be situated anywhere, but skull base locations are predominant. In a recent series published by the Pittsburgh team,61 tumor was localized to the middle fossa in 351 patients, posterior fossa in 307, convexity in 126, anterior fossa in 88, parasagittal region in 113, and other areas in 115 patients.
Technical Considerations
The optimal radiosurgery dose for meningioma is still under debate. Selection of doses depends on tissue response, tumor volume, and the tolerance of neighboring structures. Doses of less than 12 Gy have been reported to be a significant factor in failure to control the growth of meningiomas.62 According to Kondziolka and associates, marginal doses greater than 15 Gy do not provide better tumor control.63 Chin and colleagues recommended 12 to 14 Gy for tumors larger than 3 cm, 16 Gy for tumors 1 to 3 cm, and 18 Gy for tumors smaller than 1 cm.64 Tishler and coworkers investigated the tolerance of the second through sixth cranial nerves to radiosurgery.65 They found a higher incidence of damage to the optic pathway in patients receiving more than 8 Gy to any part of the optic apparatus. Leber and coworkers indicated that no signs of radiation-induced optic neuropathy were observed when the dose to the visual pathway was less than 10 Gy whereas this complication occurred in 26.7% of their patients when the dose varied from 10 to less than 15 Gy and in 77.8% when the dose was 15 Gy or higher.66 In contrast, Morita and colleagues showed that it was possible to safely deliver 12 to 16 Gy to short segments of the visual pathway.66a Tishler and associates indicated that the oculomotor nerves in the cavernous sinus could tolerate doses greater than 20 Gy whereas the trigeminal nerve was potentially at risk with doses beyond 19 Gy.65 Several studies have recommended that the lateral wall of the cavernous sinus, pituitary gland and stalk, hypothalamus, and brainstem not receive more than 15 Gy.
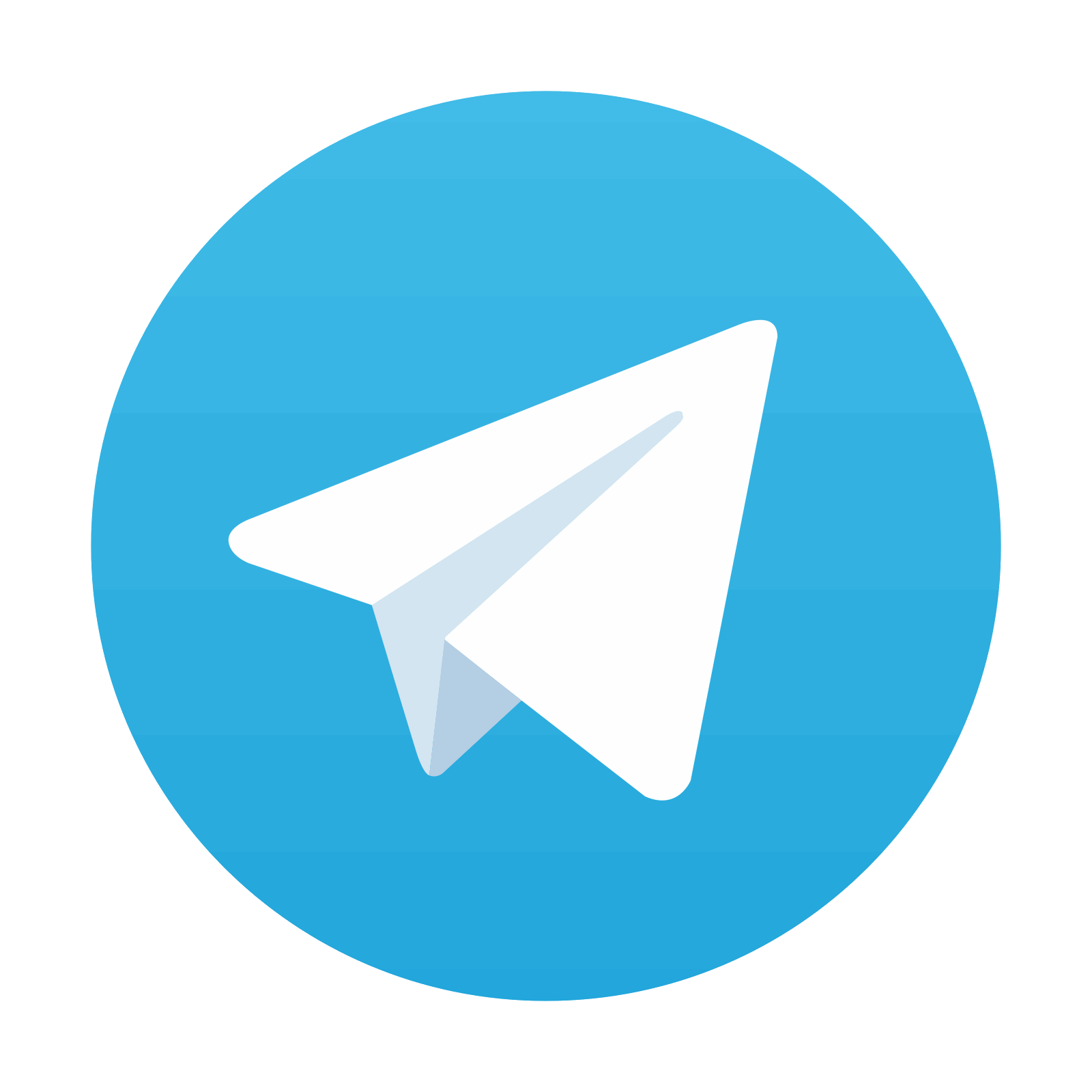
Stay updated, free articles. Join our Telegram channel

Full access? Get Clinical Tree
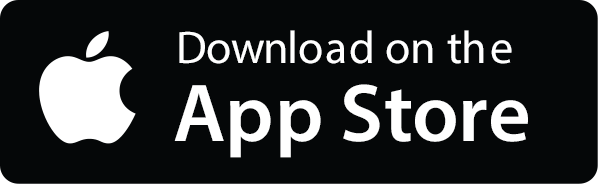
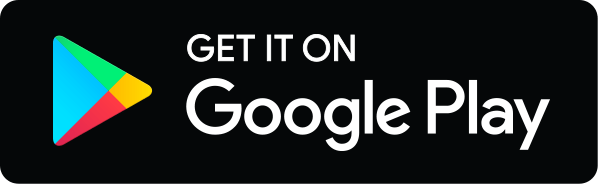