6 Repetitive Nerve Stimulation
Normal Neuromuscular Junction Physiology
The NMJ essentially forms an electrical–chemical–electrical link between nerve and muscle (Figure 6–1). The chemical neurotransmitter at the NMJ is acetylcholine (ACH). ACH molecules are packaged as vesicles in the presynaptic terminal in discrete units known as quanta; each quantum contains approximately 10,000 molecules of ACH. The quanta are located in three separate stores. The primary, or immediately available store consists of approximately 1000 quanta located just beneath the presynaptic nerve terminal membrane. This store is immediately available for release. The secondary, or mobilization store consists of approximately 10,000 quanta that can resupply the primary store after a few seconds. Finally, a tertiary, or reserve store of more than 100,000 quanta exists far from the NMJ in the axon and cell body.
Physiologic Modeling of Repetitive Nerve Stimulation
1. m = pn, where m represents the number of quanta released during each stimulation; p is the probability of release (effectively proportional to the concentration of calcium), typically approximately 0.2 in normal subjects; and n represents the number of quanta in the immediately available store (at baseline, approximately 1000 in normal subjects).
2. The mobilization store starts to replenish the immediately available store after 1 to 2 seconds.
3. Approximately 100 ms is required to pump calcium out of the presynaptic terminal. If stimulation occurs again sooner than 100 ms (i.e., stimulation rate >10 Hz), the calcium concentration increases, the probability of release of ACH quanta increases, and more quanta are released.
Modeling Slow Repetitive Nerve Stimulation
The effects of slow RNS on the EPP, the muscle fiber action potential (MFAP), and the CMAP can best be illustrated with the following three examples (Figure 6–2A–C):
In this first example, initially there are 1000 quanta in the immediately available store (n), and with each stimulation, 20% of the quanta are released. If the EPP is >15 mV (threshold in this example), a muscle fiber action potential is generated. Note the normal depletion of the immediately available store (n), the subsequent decline in the number of quanta released (m), and the corresponding fall in the EPP from the first to the fourth stimulation. During the second stimulation, only 160 quanta are released instead of the initial 200 because the number of quanta in the immediately available store has dropped to 800 (1000 minus the 200 released during the first stimulation), and subsequently 20% of the 800 is released. At the fifth stimulus, however, sufficient time has elapsed for the secondary or mobilization store to begin to resupply the primary store. The number of quanta in the immediately available store increases, with a corresponding increase in the number of ACH quanta released, resulting in a higher EPP. Note that at all times the EPP stays above threshold (15 mV), resulting in the consistent generation of a muscle fiber action potential (Figure 6–2A). In the EDX laboratory, these findings translate to normal baseline CMAPs with no change in amplitude, because action potentials are generated in all muscle fibers.
In this next example, the number of quanta in the immediately available store (n), the number of quanta released (m), and the depletion of quanta with slow RNS all are normal. The response to the quanta (i.e., the EPP) is abnormal, however. Whereas in normal subjects the release of 200 quanta generated an EPP of 40 mV, in this case the same number of quanta generates an EPP of only 20 mV. Accordingly, the safety factor is reduced. In myasthenia gravis, this occurs as a result of fewer ACHRs and, accordingly, less binding of ACH. The reduced safety factor, in conjunction with normal depletion of quanta, results in subsequent EPPs falling below threshold and their corresponding muscle fiber action potentials not being generated (Figure 6–2B). As the number of individual MFAPs declines, a decrement of CMAP amplitude and area occurs. This decrement reflects fewer EPPs reaching threshold and fewer individual MFAPs contributing to the CMAP. Often, after the fifth or sixth stimulus, the secondary stores are mobilized and no further loss of MFAPs occurs. This results in stabilization or sometimes slight improvement or repair of the CMAP decrement after the fifth or sixth stimulus, giving the characteristic “U-shaped” decrement (see later).
In this next example, the number of quanta in the immediately available store (n) is normal, and the EPP is normal for the number of quanta released (m). What is abnormal is the number of ACH quanta released (m) and the baseline EPP. In Lambert–Eaton myasthenic syndrome, the calcium concentration in the presynaptic terminal is reduced, due to an antibody attack on the voltage-gated calcium channels. Thus, the probability of release (p) falls dramatically, along with a decrease in the number of quanta released. There still is depletion, although it is not as marked as in normal or postsynaptic disorders. Simply because so few quanta are released, the subsequent amount of depletion cannot be as great. In this example, because the EPP is below threshold at baseline, a muscle fiber action potential is never generated (Figure 6–2C). Thus, the baseline CMAP is low in amplitude because many muscle fibers do not reach threshold due to inadequate release of quanta after a single stimulus. With slow RNS, there is also further decrement of the CMAP because subsequent stimuli result in further loss of MFAPs. Just as in postsynaptic disorders, after the fifth or sixth stimulus, the secondary stores are mobilized and no further loss of MFAPs occurs. This results in stabilization or sometimes slight improvement or repair of the CMAP decrement after the fifth or sixth stimulus, giving the characteristic “U-shaped” decrement (see later). Note that in some presynaptic disorders, the baseline EPP may be low but still above threshold, resulting in a reduced safety factor. In this situation, a muscle fiber action potential initially may be generated but then fails to be generated as the EPP falls below threshold with slow RNS.
Modeling Rapid Repetitive Nerve Stimulation
The effects of rapid RNS can be deduced from the three basic assumptions (see section on Physiologic Modeling of Repetitive Nerve Stimulation). With rapid RNS, the depletion of quanta is counterbalanced by (1) increased mobilization of quanta from the secondary to the primary store, and (2) calcium accumulation in the presynaptic terminal, which increases p, the probability of release. The sum of these influences usually results in a greater number of quanta released and higher EPPs with rapid RNS.
Presynaptic NMJ disorders are distinctly different. Because the EPP is abnormally low at baseline in those disorders – often below threshold – rapid RNS may increase the EPP above threshold so that a muscle fiber action potential is generated where one had not been present previously (Figure 6–2D).
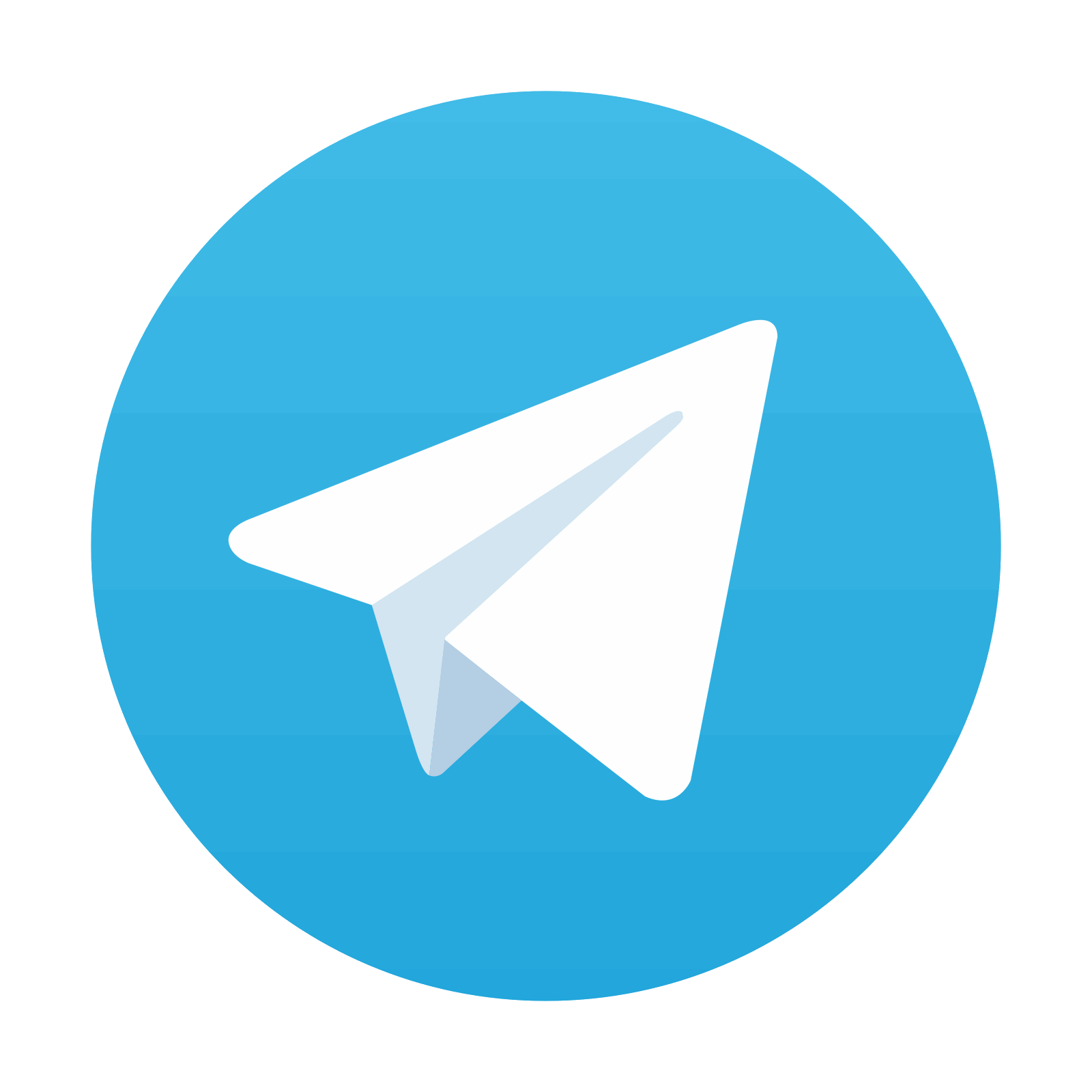
Stay updated, free articles. Join our Telegram channel

Full access? Get Clinical Tree
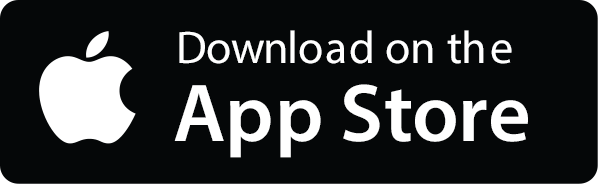
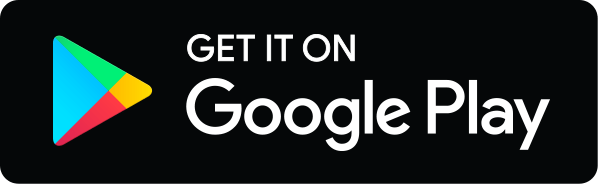