Fig. 11.1
Schematic outline of the central autonomic network model
11.4 HRV Measures of Autonomic Function
The heart rate variations around the mean value over the time sample epoch mirror to a substantial extent of the cardiorespiratory control system and is now regarded as a reliable descriptor of the sympathetic/parasympathetic functional interplay and influence on heart function [57–59]. HRV measures are thought to reflect both ANS dynamics and heart–brain interaction and – to some extent and possibly only indirectly – also higher brain functions [3, 6, 60].
HRV recording techniques are noninvasive; HRV signals have an excellent signal-to-noise ratio compared to those investigated in neuroscience or clinical neurophysiology [61, 62]. However, the HRV signal is not periodic; its stimulus- or condition-related changes are within the physiological range in the absence of cardiac disorders and are seldom detectable without appropriate data treatment. To this purpose, the tachogram needs processing in the time or frequency domains or by geometric or nonlinear methods [59]. The HRV descriptors derived in the time domain quantify the variance during the recording window. The fast Fourier transform (FFT) or autoregressive models are of common use for analyses in the frequency domain. The signal spectral profile is characterized by three main components: (1) the high-frequency interval (0.15–0.5 Hz; HF) is mainly associated with activation of the parasympathetic nervous system; (2) the low-frequency interval (0.04–0.15 Hz; LF) is thought to reflect contributions from both the parasympathetic and sympathetic systems; and (3) the very-low-frequency bandwidth (<0.04 Hz; VLF) is thought to reflect temperature, vasomotor, hormonal, and metabolic regulation. Methods to describe the complexity, irregularity, or randomness of HRV and its changes (e.g., the approximate entropy (ApEn) or sample entropy (SampEn)) are available and in use [63, 64]. Stimulus- or condition-related changes occurring in selected frequency intervals of the HRV frequency spectrum are deemed expressive of specific functional changes [52].
11.5 Autonomic Control and Responsiveness in the Disorders of Consciousness
HRV has been successfully applied to study posttraumatic patients and subjects in VS/UWS or minimally conscious state. Improved HRV within 3 months from TBI was correlated with recovery of autonomic functions in a prospective study [65]. Sympathetic activation in response to auditory stimuli was reduced in acute VS/UWS even in subjects with a relatively good recovery [66]. Increased sympathetic response and recovery of the sympathovagal balance were correlated with recovery of consciousness [67]. In contrast, a severe sympathetic and motor overactivity syndrome indicative of over-responsiveness to afferent stimuli was associated with poor outcome [68].
VS/UWS subjects can process complex stimulus conditions, with greater responsiveness to emotional than nonemotional stimulus conditions [53]. Music appears peculiarly efficient in promoting arousal and responsiveness in DoC [69], with activation of the superior temporal gyri by music possibly predicting the evolution from VS/UWS [70]. Autonomic activation and the EEG amplitude reportedly increase in response to preferred music [69]. Changes in the HRV patterns of response indicative of enhanced activity of the cardiovascular system were observed after 14-day music therapy [71]. Replicable changes in the sympathovagal balance have been identified in VS/UWS during passive listening to symphonic music of different authorships by means of advanced data mining analyses of HRV descriptors. These descriptors allowed cluster of the autonomic responses as indicative of positive or negative emotions in both VS/UWS and awake posttraumatic subjects; the patterns of change were comparable across clinical conditions and among subjects for each music sample [56]. Electrodermal and HRV changes induced by the presence or voice of relatives (the mom effect) have been described in VS/UWS [72, 73]. The observation of autonomic changes in response to complex stimulus conditions with emotional value adds to the evidence from neuroimaging studies and has comparable implications as to the strategies and criteria of evaluation of the residual responsiveness of these subjects, their diagnosis and prognosis, and the planning of neurorehabilitation strategies [54].
11.6 Responsiveness in the Disorders of Consciousness and Variability
Diagnosis, monitoring of evolution, and early prognosis in DoC still rest mostly on the clinical evaluation of responsiveness [74]. However, the probability of observing an established clinical indicator can vary also in single subjects [75, 76]. For instance, the incidence of a visual pursuit response (a marker of evolution into the minimally conscious state also observed in about 20 % of VS/UWS subjects) was higher in the morning than in the afternoon testing during a 13-week neurorehabilitation protocol in both VS/UWS and minimally conscious state [77]. When subjects were repeatedly tested during the day, a response was most frequently detected at about 10.30 am and 3.00 pm and never at postprandial time [76]. The overall probability of observing a visual pursuit response at least once over the course of a single day was 30–40 % [76], in agreement with the reported rate of misdiagnosis between VS/UWS and the minimally conscious state [78, 79]. This variability of response could be accounted for by residual fragments of circadian/ultradian cycles asynchronous among subjects or by the fluctuations of the brain functional state that are observed in healthy humans and animals. Although apparently spontaneous, these fluctuations correlate with neuronal and non-neuronal biological parameters that vary within the physiological range depending on the momentary functional or homeostatic requirements and interact with each other [80]. Among these parameters are the spontaneous or environment-related changes in temperature, metabolism (e.g., glucose, ammonia), blood flow, oxygen extraction, pO2, pCO2, blood availability of ferritin, hormones (thyroxine, sexual hormones, cortisol, steroids, ATCH) and neurohormonal interaction, light–dark regulation of serotonin, histamine and dopamine/melatonin/vitamin B12 secretion and interaction, etc. These parameters can individually or collectively account for unexplained or underestimated individual variability and may become clinically relevant on occasions. The incidence of a pursuit response in VS/UWS subjects was found to correlate with and to be predicted by the HRV measures of sympathovagal balance and autonomic functional state. The HRV descriptor values at which the observation of a response was most frequent or unusual have been identified and the predictability of the model has been validated [81].
11.7 Comment
Extensive monitoring of the autonomic nervous system is advisable in the disorders of consciousness, and HRV techniques appear suitable of application in this field. HRV methodologies benefit from being noninvasive and with positive benefit/cost ratios, and measures are obtained at limited costs, labor, and accuracy of recording. Albeit indirect, information on the ANS functional state or responsiveness can be obtained also in absence of the subject’s collaboration and when sophisticated experimental designs and data recording procedures are impracticable or difficult as is the case with the severe disorders of consciousness. Specifically, monitoring by HRV techniques is practicable with the same methodological approach in early phases at the ICU or hospital, during the neurorehabilitation procedures, and with remote control at home. The CAN model provides neuroscientists and clinicians with an independent approach to be used both in the understanding of the HRV descriptors of the autonomic–CNS interaction and supplementing clinical or neuroimaging observations.
Functional neuroimaging has identified brain activation suggesting awareness in only a small percentage of subjects [82]. The residual functions were otherwise regarded as resulting from intact but functionally disconnected cortical modules [74, 82], while the preservation of specific neural structures and residual resources has been suggested to vary with the heterogeneity of etiology and pathophysiology or extension and severity of brain damage [83]. The current clinical criteria for diagnosis and prognosis of the disorders of consciousness based on neurological signs of responsiveness should be reconsidered. The criteria should include the variability of responsiveness over time, and multiple testing should be mandatory to reduce the risks of misclassification. The correlation with the functional state of the ANS should be regarded as an independent indicator for diagnosis and prognosis and taken into proper account; monitoring should be extensive and focus also on non-neuronal factors. Reclassification of some patients or classes of patients may prove appropriate if based on systematic investigation.
References
1.
2.
Posner J, Russell JA, Peterson BS (2005) The circumplex model of affect: an integrative approach to affective neuroscience, cognitive development, and psychopathology. Dev Psychopathol 17:715–734PubMedCentralCrossRefPubMed
3.
Appelhans BM, Luecken LJ (2006) Heart rate variability as an index of regulated emotional responding. Rev Gen Psychol 10:229–240CrossRef
4.
5.
6.
Mashin VA, Mashina MN (2000) Analysis of the heart rate variability in negative functional states in the course of psychological relaxation sessions. Hum Physiol 26:420–425CrossRef
7.
Napadow V, Dhond R, Conti G, Makris N, Brown EN, Barbieri R (2008) Brain correlates of autonomic modulation: combining heart rate variability with fMRI. Neuroimage 42:169–177PubMedCentralCrossRefPubMed
8.
9.
10.
11.
12.
13.
14.
Kuusela TA, Kaila TJ, Kähönen M (2003) Fine structure of the low-frequency spectra of heart rate and blood pressure. BMC Physiol 3:11PubMedCentralCrossRefPubMed
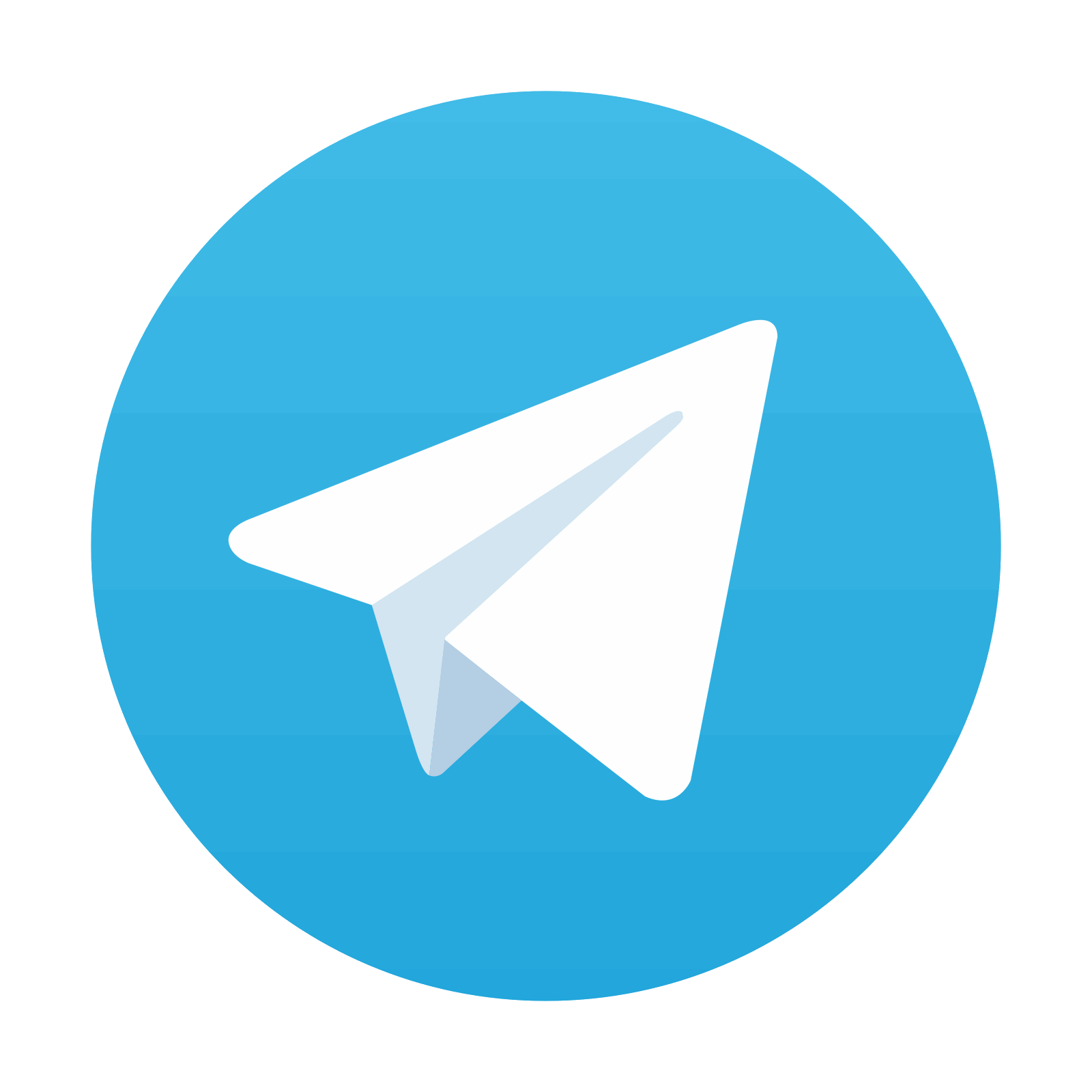
Stay updated, free articles. Join our Telegram channel

Full access? Get Clinical Tree
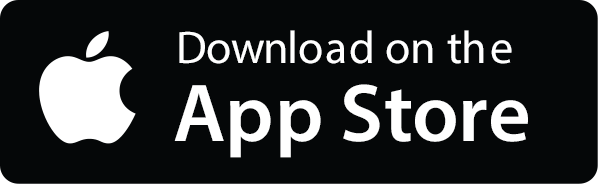
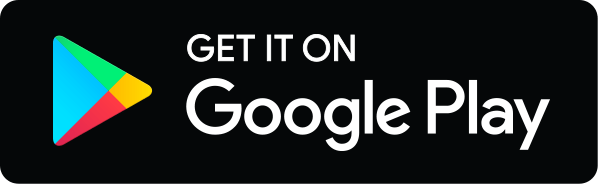