Seizures and Epilepsy in Infants to Adolescents
Douglas R. Nordli Jr.
James J. Riviello Jr.
Ernst Niedermeyer
BASIC CONSIDERATIONS
Definition and Prevalence
There is no disease named “epilepsy.” Rather, epileptic seizures are abnormal reactions of the brain caused by a large number of diseases. The entire brain or parts of it may be involved; the extent of involvement largely determines the seizure type. Epilepsy originates in the brain, but systemic disorders and resulting metabolic or toxic effects may cause secondary encephalopathies, and thus, secondary epileptic seizures. Genetic predisposition also plays a very important role.
The prevalence of epileptic seizures has been estimated to be between 0.5% (core group) and 5% (fringe group with at least one seizure during life). More precise figures of Juul-Jensen and Foldspang (1) lie between 1.27% and 2.44%. Gumnit (2) estimates that core and fringe group may add up to 9% of the population.
An overview of various epilepsy prevalence studies (3) shows fairly consistent figures: Mumbai, India 3.7; Rochester, Minnesota, 6.66; Denmark, 6.9; Poland, 7.8; Nigeria, 5.3; Mississippi, 6.78; and China, 4.57 (prevalence per 1000). Similar figures were presented by Greulich and Gerber (4) and Hauser (5).
Introduction and Historical Remarks
Electroencephalography (EEG) has revolutionized the entire field of epileptology. No one can deny that ingenious and even great concepts arose in the pre-EEG era; clinical acumen and keen analysis of the observed phenomena resulted in remarkable descriptions that have stood the test of time. Indeed, focal motor seizures, infantile spasms, rolandic seizures, and absence seizures, to list but a few, were all recognized prior to the development of EEG. Conventional EEG has opened new vistas and modern EEG techniques, especially the prolonged EEG monitoring of people with epilepsy, have made further important epileptologic contributions. These noninvasive techniques are complemented by invasive recordings from cortex and deep structures that continue to provide insights into fundamentals of epilepsy.
We review the role of the EEG in the epilepsies, including the correlation of the semiology with the electrographic findings (electroclinical correlation) that are contributory to the EEG diagnosis of epileptic phenomena in ictal states or the interictal period in various epileptic conditions.
The interictal paroxysmal phenomena are discussed first. These discharges represent the basic elements of more complex ictal patterns.
Principal Differences between Interictal and Ictal Discharges
A distinction is made between ictal and interictal paroxysmal activity. This logical differentiation, however, is beset with confusion. It is sometimes difficult or even impossible to distinguish between ictal-clinical, ictal-subclinical, and interictal paroxysmal EEG activity, even using neuropsychological tests. Computerized testing demonstrates the existence of a gray zone between interictal and ictal paroxysmal activities (e.g., transient cognitive impairment).
Problems of Terminology
Definitions of EEG events are indispensable, but they are also quite difficult to arrive at and are often unsatisfactory. Attempts to reassess EEG terminology have been made periodically; they often result in neologistic construction of terms that fail to receive general acceptance. EEG terminology is filled with widely used, popular, and, often quite sloppy and inaccurate terms. Nevertheless, most electroencephalographers know what is meant by such expressions in the professional jargon.
One could argue that the busy electroencephalographer will always be satisfied with the nomenclature learned during the training period, whereas the academicians among electroencephalographers constantly strive for an unobjectionable and crystal clear terminology free from linguistic flaws and capable of defining an EEG event from a variety of aspects. This view, however, is oversimplified. Academia sometimes has a vested interest in certain terms; it may bitterly resent new terms recommended by international committees.
A preliminary proposal by the International Federation of Societies for Electroencephalography and Clinical Neurophysiology (6) was followed by a definitive proposal (7) and a glossary of terms used by clinical electroencephalographers (8). This glossary was updated in 1999 (9). The handbook catalogue of Dutertre (10) deserves special admiration. Unfortunately, the definition of electrophysiologic events can never do justice to all facets of the phenomenon. The question then arises as to whether we must rigidly follow the new nomenclature. A negativistic attitude must be castigated, but electroencephalographers should not be pressured to strictly adhere to newly proposed terms as time may be needed for these terms to achieve consensus.
As to epileptic discharges, there has been considerable disunity in the ranks of electroencephalographers. The term epileptic discharge has been criticized because such discharges
may occur in the absence of clinical seizure manifestations or in individuals who have never had seizures. The same is true for the widely used term seizure discharge; even paroxysmal discharge, certainly a more cautious and unassuming term, has not found general acceptance. The term epileptiform discharge is an elegant solution since it addresses the critical issue: an epileptiform discharge demonstrates an underlying epileptic pathophysiology but its presence alone does not make the diagnosis of epilepsy. The diagnosis of epilepsy remains a clinical one, which must be based on actual clinical events.
may occur in the absence of clinical seizure manifestations or in individuals who have never had seizures. The same is true for the widely used term seizure discharge; even paroxysmal discharge, certainly a more cautious and unassuming term, has not found general acceptance. The term epileptiform discharge is an elegant solution since it addresses the critical issue: an epileptiform discharge demonstrates an underlying epileptic pathophysiology but its presence alone does not make the diagnosis of epilepsy. The diagnosis of epilepsy remains a clinical one, which must be based on actual clinical events.
INTERICTAL EPILEPTIFORM DISCHARGES
Spike (Single or Random Spike)
According to IFSECN (8), a spike is a transient, clearly distinguished from the background activity, with pointed peak at conventional paper speed and a duration from 20 to under 70 msec; the main component is generally negative and the amplitude is variable.
The distinction from the background activity is based on wave morphology and amplitude. In many cases, spikes stand out against the background because of their high voltage. If the voltage of spikes and background activity is approximately equal, the faster character (i.e., the shorter duration) of the spike is its distinctive feature. Is it possible that a spike of 50 msec duration and moderate amplitude may be embedded in 20 Hz activity (50 msec wave duration), for instance, in an epileptic with considerable drug-induced fast activity? Under these circumstances, the spike activity may be undetectable. In such a case, a fast paper speed could demonstrate the morphologic features of the spike (multiphasic and more pointed character with a dominant negative phase) in contrast with the more monotonous appearance of the fast waves. In the digital era, paper speed is reflected in the sweep time.
Spikes have many characteristics in common and yet there are also remarkable inter- and intraindividual variations (Fig. 26.1) and inconsistencies between one spike and the next in the same lead (11). Spikes appear to be hypersynchronous events due to excessive simultaneous neuronal discharge. This view, however, is not congruent with the desynchronized (low voltage) EEG aspect of “electrodecremental seizures” or the electroincremental initiation of focal or generalized attacks. Definitions such as “epilepsy is paroxysmal hypersynchrony” (12) may be correct on the neuronal level but partly incorrect from the viewpoint of the “macro-EEG.”
Basic neurophysiologists have been cautioning against the casual use of terms such as synchronization and desynchronization. Low-voltage fast rhythms are not necessarily desynchronized and may be associated with enhancement and synchronization within intracortical networks.
On the basis of long-term monitoring and computer analysis, Gotman (13) has raised the question as to whether interictal spikes are in reality postictal. Gotman (13) and Gotman and Marciani (14) observed marked and prolonged increase of interictal spikes in a patient after a seizure (awake as well as asleep). Drug levels had little influence on the rate of spike activity, and there was no increase of spiking prior to a seizure.
The multiphasic character of a single spike warrants particular emphasis. A sequence of a minor positive (the “positive prespike”), a major negative, and a second minor positive component (Fig. 26.1) is typical in most instances. A slow negative component may trail the spike discharge and often attain about the same amplitude as the negative main component of the spike. This trailing slow component of a single spike should not be regarded as evidence of a spike-wave complex. Dutertre (10) in his handbook catalogue shows examples of single spike-wave complexes that one could just as well describe as compounded or multiphasic single spikes. From the vantage point of basic neurophysiology, there is some reason to presume that the trailing slow wave is caused by the same type of hyperpolarization demonstrated by Pollen et al. (15) in the experimental analysis of the spike-wave discharge.
The electroencephalographer very seldom finds single spikes on the scalp with predominant positive component (16,17). Positive single spikes are more common in electroencephalographic and depth recordings; on the scalp, predominant positivity of single spikes raises the question of defective superficial cortical laminae. Following surgery of cortical arteriovenous (AV) malformations or after traumatic laceration (18), such positive spikes may be present occasionally. With dipole formation (especially in benign rolandic epilepsy), a positive spike discharge may be found in a moderate distance from the negative spike focus (19,20).
Spikes represent the basic element of epileptiform activity in the EEG. A unitarian view that all spikes mean a hidden or overt epileptic event would be erroneous. The fine semiology of spikes is extremely important and the EEG interpreter must consider the following questions:
What is the precise wave morphology?
Where do the spikes occur?
What is the patient’s age?
What is the state of awareness?
Is there any possibility of an artifact of similar appearance?
Is there any possibility of a physiologic potential of similar
appearance? Discrepancies between spikes and behavioral epileptic events
are not uncommon at all. This highly complex situation has
been reinvestigated by Niedermeyer (21).
Wave Morphology
The largest and most pronounced spikes are not necessarily associated with more serious epileptic seizure disorders. On the contrary, rolandic spikes in a child age 4 to 10 years are very prominent; however, the seizure disorder itself is usually quite benign or there may be no clinical seizures at all. Alternatively, “small sharp spikes” are indeed marginal spike discharges and will be discussed separately.
Spatial Distribution
In childhood, occipital spikes are, in general, the most benign spike discharges, with less than 50% having clinical seizures; rolandic central-midtemporal-parietal spikes are also quite benign, whereas frontal spikes or multifocal spikes are more epileptogenic.
Age
The significance of the age factor is enormous. From the spikes of an epileptic newborn to a seizure focus of old age, age-determined varieties of spikes can be distinguished.
Level of Awareness
Random spikes may occur at any state of waking-sleeping cycle and occur even in rapid eye movement (REM) sleep when bilateral synchronous bursts of spikes or spike waves are usually suppressed.
Repercussions on Perceptual Function
Shewmon and Erwin (22,23) investigated the impact of spikes located over the occipital region and its vicinity on visual perception and reaction time in three subjects with posterior spikes. Their work shows that the visuomotor reaction time was prolonged (or the response was absent) when the task was time locked to the spike. Furthermore, “spike-induced dysfunction was most pronounced when either response hand or visual field of stimulus was contralateral to the spike” (23). In a further study, Shewmon and Erwin (24) emphasized the significance of the trailing slow wave that follows a single spike discharge. The perceptive disturbance was particularly pronounced when the task was presented during the slow wave. These fascinating findings indicate that even single spikes of rapid duration may be capable of momentary impairment of the cortical visual function.
Distinction from Similar Physiologic Patterns
This differentiation is particularly important in the case of vertex sharp waves during deep drowsiness and stage 2 of light non-REM sleep. In childhood (after age 4), these waves may have a particularly spiky appearance and may be misinterpreted as paroxysmal spikes.
Physiologic sharp or spiky vertex waves are usually quite easily distinguished from rolandic spikes in sleep (Fig. 26.2). Even in the more uncommon case of epileptiform spike discharges over the vertex, the differentiation from physiologic vertex sharp waves is not difficult.
The physiologic nature of the occipital lambda waves and “lambdoid” activity (positive occipital transients of sleep) must also be considered. The main component of this pattern is positive.
Distinction from Artifacts of Similar Appearance
This distinction depends on the electroencephalographer’s experience and is usually an easy one.
The interpretation of the clinical significance of spikes can be extremely difficult and depends on the electroencephalographer’s experience in the art of reading the EEG recording and also on the clinical understanding of epileptologic problems. Extensive personal laboratory experience is just as essential as scientific knowledge in interpreting the EEG.
Are Interictal Spikes Nothing but EEG Signals?
Our earlier view (found in this chapter in previous editions)— namely that single spikes are an EEG signal and nothing else— is in need of some correction. This seems to be particularly true for “negative myoclonus” (ictally suppressed motor activity), examples of which have been presented by Gambardella et al. (25) and Werhahn and Noachtar (26) (also see Ref. 27). A single
spike may also cause changes in cerebral blood flow and increase of metabolic demands (28), demonstrated with the simultaneous use of EEG and functional magnetic resonance imaging (fMRI).
spike may also cause changes in cerebral blood flow and increase of metabolic demands (28), demonstrated with the simultaneous use of EEG and functional magnetic resonance imaging (fMRI).
Sharp Waves
According to IFSECN (8), a sharp wave is a transient, clearly distinguished from background activity, with pointed peak at conventional paper speeds and duration of 70 to 200 msec, that is, more than approximately 1/14 to 1/5 sec. The main component is generally negative relative to the other components. The term is restricted to epileptiform discharges and does not apply to distinctive physiologic waveforms, such as vertex sharp transients, lambda waves, and POSTS.
Jasper (31) pointed out that the rising phase of the sharp wave is of the same order of magnitude as in spikes, but the descending phase is prolonged. The configuration with a steeper ascending phase, however, is not always present. Examples of sharp waves are shown in Figure 26.3.
A very unusual type of repetitive slow sharp wave activity has been reported in prematurely born infants with intraventricular hemorrhage; these discharges show predominantly positive polarity and are recorded mainly over the rolandic region (32,33). The maximum of this activity is sometimes found over the vertex.
Spikes and sharp waves are neurophysiologically closely related phenomena; both of them are typical epileptiform discharges and highly suggestive of an epileptic seizure disorder, although both phenomena may occur in patients without a history of seizure disorder.
Sharp waves are usually found as random focal discharges; most anterior temporal spikes are, in a strict sense, sharp waves. This is also true for most benign rolandic spikes of childhood. Sharp waves are more seldom found in generalized synchronous bursts where single spikes, spike waves, and polyspikes predominate.
It has been contemplated that sharp waves on the scalp correspond with spikes in the depth or on the cortex. Combined depth and scalp recording clearly refutes this view (34). One can detect spikes as well as sharp waves in depth recordings; a deep sharp wave usually corresponds with a sharp wave on the scalp if there is any corresponding scalp activity at all.
The long duration of a sharp wave permits better insight into the multiphasic character of this potential. A small preceding positive component may be very fast and qualify as a spike; even a small biphasic spike discharge may precede the much larger sharp wave. Some sharp waves even exceed the maximum length of 200 msec of the IFSECN definition (8) (Fig. 26.4), also named “slow sharp waves” (35) or in cases of particularly long duration, “blunted sharp waves.” Others become very complex. They consist of a constantly varying number of components; such compounded sharp waves may occur as the periodic discharges (periodic lateralized epileptiform discharges) described by Chatrian et al. (36).
![]() Figure 26.4 An example of a very slow sharp wave (blunted sharp wave), maximal over T6, obtained from a 6-year-old boy with craniocerebral trauma at age 4 followed by seizures. |
It is certainly not incorrect to use the terms spikes and sharp waves synonymously when discussing the clinical significance of a focal transient.
Multiple Spike Complex or Polyspike Complex
This discharge type represents a complex of spikes and may also be called a polyspike complex. In modern terminology (8), the term multiple spike complex is preferred because “polyspikes” is an etymologic hybrid. It has been defined as a complex paroxysmal EEG pattern with close association of two or more diphasic spikes occurring more or less rhythmically in bursts of variable duration, generally with large amplitudes (10) (Figs. 26.5 and 26.6).
Polyspike bursts are readily elicited by electrical stimulation of single depth leads, especially in limbic regions. On the scalp, however, polyspikes occur mostly as bilateral or generalized synchronous discharges. Exceptional focal polyspikes are occasionally encountered; these usually have a frontal maximum, except for occipital accentuation in hypsarrhythmia. Polyspikes and also polyspike-wave complexes are sometimes associated with concomitant myoclonus, especially in primary generalized epilepsy and in photosensitive individuals with this type of
seizure disorder. Children with Lennox-Gastaut syndrome may also show association of polyspikes and myoclonus.
seizure disorder. Children with Lennox-Gastaut syndrome may also show association of polyspikes and myoclonus.
Runs of Rapid Spikes
This pattern has been described as “grand mal discharge” (37,38), “fast paroxysmal rhythms” (39), “rhythmic spikes” (40), and “generalized paroxysmal fast activity” (41). It is seen only in sleep and occurs in older children, adolescents, and younger adults (Fig. 26.7). It consists of bursts of spike discharges at a rate from 10 to 25 Hz, usually generalized but with a well-defined maximum over frontal regions; it may even be confined to the frontal leads. The voltage is in the medium to high range, often exceeding 100 or even 200 µV. The discharge rate is in most cases somewhat irregular. The bursts last for about 2 to 10 seconds; bursts of more than 5-second duration are usually associated with tonic seizures and thus represent an ictal pattern.
The obvious misnomer “grand mal discharges” is based on certain similarities with the ictal EEG of a generalized tonic-clonic seizure. In a patient population with generalized tonic-clonic seizures, runs of rapid spikes as an interictal pattern are a very rare finding. This has been confirmed by the data of Chayasirisobhon et al. (42). The inappropriateness of the term grand mal discharge was stressed by Rodin et al. (43), who thought that this pattern occurred in patients with primary generalized epilepsy who suffered from more than one type of seizure and especially from akinetic seizures. As already noted by Gastaut et al. (40), this discharge is very typical in patients with Lennox-Gastaut syndrome; it is hardly ever found outside
this clinical entity (44, 45 and 46). It may be found in very rare instances of posttraumatic epilepsy with EEG traits of the Lennox-Gastaut syndrome (47). Brenner and Atkinson (41), however, insist that runs of rapid spikes (“generalized paroxysmal fast activity”) may be found outside the Lennox-Gastaut syndrome. In our experience, runs of rapid spikes may occur in all “imitators” of the Lennox-Gastaut syndrome (48,49).
this clinical entity (44, 45 and 46). It may be found in very rare instances of posttraumatic epilepsy with EEG traits of the Lennox-Gastaut syndrome (47). Brenner and Atkinson (41), however, insist that runs of rapid spikes (“generalized paroxysmal fast activity”) may be found outside the Lennox-Gastaut syndrome. In our experience, runs of rapid spikes may occur in all “imitators” of the Lennox-Gastaut syndrome (48,49).
Little is known about the underlying neurophysiologic mechanisms of this pattern. It is clearly a frontal lobe discharge, and the associated tonic seizures are probably arising from the premotor and supplementary motor portions of the frontal lobes. There could be certain similarities to the electrodecremental seizure patterns, which also originate most commonly from the frontal lobe (50). This electrodecremental pattern may actually be associated with high-frequency discharges (high-frequency oscillations) that are not detectable at the scalp, giving the false impression of a decrement in cortical activity (51).
Unfortunately, those electroencephalographers who never use sleep portions in routine records will never see this fascinating pattern, which is definitely in need of further basic research.
Spike-and-Slow-Wave Complex (Classical 3 Hz Spike Wave)
Classical 3 Hz spike-wave complexes are widely known even outside the community of electroencephalographers. It is officially termed spike-and-slow-wave complex (8); this term comprises all types of spike-wave complexes, which are listed separately because of markedly differing associated clinical-epileptologic conditions. The official definition (8) is quite simple: “A pattern consisting of a spike followed by a slow wave.” Older terms such as spike-and-dome complex, wave-and-spike complex, and dart-and-dome complex are seldom used these days and should be discouraged. The abridged term 3 Hz spike wave is certainly acceptable, because the omitted word complex is automatically implied.
Spike-wave complexes of the classical variety (3 Hz) have been described as the EEG pattern of absence seizures (38). Since that time, the 3 Hz spike-wave discharge has been equated with absence epilepsy. Regrettable mistakes have occurred due to lack of communication between electroencephalographers and clinicians who initiated treatment with anticonvulsants specific for an absence seizure due to the use of the term 3 Hz spike-wave complexes alone. Unfortunately, the term petit mal discharge (37) has reinforced this type of reflex thinking. Fortunately, electroencephalographers and epileptologists have always cautioned against the misinterpretation of the term petit mal discharges; this should be interpreted as “discharges that occur in absences but also in other conditions.” The work of Silverman (52), Clark and Knott (53), and Lundervold et al. (54) has clearly shown that the spike-wave discharge as an interictal event correlates with the occurrence of petit mal absences only in moderate percentage of patients (16% according to Clark and Knott).
The 3 Hz spike-wave discharge is a worthwhile subject for more detailed discussion. As to wave morphology, there is good evidence that the spike-wave complex is not simply an association of a spike and a slow wave, although the above-mentioned definition is justifiable as a simplification. The spike-wave complex contains hidden components that can be seen even with
simple visual analysis at 30 mm/sec paper speed; special recording methods will better reveal these extra components. Gastaut and Hunter (55) and Cohn (56) and Weir (57) have elucidated the components of this pattern. Most important is a spike of positive polarity during the descending portion of the slow wave (57), becoming evident as notching under usual recording conditions.
On the basis of experimental studies of cortical faradization, Jung and Toennies (58) stressed the inhibitory character of the slow wave following a spike discharge (“Bremswelle”). Experimentally induced spike-wave-like activity in cats, elicited by electrical stimulation of thalamic intralaminar structures, was studied with intracellular cortical microelectrodes (15,59,60). According to Pollen (59), all components of the spike-wave complex were the “consequence of postsynaptic activity.” This author points out that “the initial positivity which may precede a negative spike (depending on the intensity of the stimulation) results from depolarization of middle and deep lying neurons, whereas the surface negative spike is the result of EPSP (excitatory postsynaptic potential) generation primarily upon apical dendrites of vertically oriented pyramidal type neurons.” According to Pollen (59), the long surface negative wave is generated by inhibitory postsynaptic potentials (IPSPs) predominantly on or near the soma of similar pyramidal cells. Thus, the slow wave indeed appears to be related to inhibitory impulses.
In this view, the spike-wave discharge apparently represents an alternating succession of excitation and inhibition. The clinical ictal activities are thus constantly curbed by intervening inhibitory impulses that prevent the discharge from progressing into massive downward discharges with motor effects (polyspikes with massive myoclonus) or to a full-blown generalized tonic-clonic seizure. For this reason, motor manifestations of ictal episodes characterized by spike waves are almost always inconspicuous or modest. Furthermore, spike-wave bursts rarely proceed into generalized tonic-clonic seizures; such rare exceptions have been demonstrated by Halász (61) and Niedermeyer (62).
The distinction between classical (3 Hz), slow (1 to 2.5 Hz), and fast (4 to 5 Hz) spike-wave complexes and the smaller 6 Hz spike-wave discharge is justified on the basis of different clinical-epileptologic correlates of each spike-wave type; it is not an example of electroencephalographic hair splitting. The classical 3 Hz spike-wave discharge is most typical and most pronounced in children with absence seizures. Clinical absences are usually present when the burst duration is greater than 5 sec. Thus, shorter bursts are usually subclinical, but numerous computerized neuropsychological studies have been employed to demonstrate a clinical component (fluctuations of level of awareness) during an apparent subclinical spike-wave bursts. The classical spike-wave complex is, in most cases, easily activated by hyperventilation, whereas the slow and the fast forms of this discharge show little or no enhancement.
A classical 3 Hz spike-wave burst does not run exactly at a rate of 3 Hz. The complexes are faster at the onset of the burst (mostly around 4 Hz), then slow down to 3.5 and 3 Hz for the main portion, and eventually slow down to 2.5 Hz at the end of the burst. During a burst, the spike discharges become gradually smaller, often shrinking to insignificance (Fig. 26.8) as in drowsiness and sleep.
The spatial distribution of the bursts is very typical; the maximum almost always lies over the frontal midline region, whereas a minimum is found over temporal and occipital areas. The different conclusion by Dondey (63) was based on EEG recordings in which no midline electrodes were used. Rodin and Ancheta (64) have confirmed the concept of a frontal midline maximum using brain mapping. Almost all spike-wave bursts are bilateral synchronous or generalized synchronous; the synchrony, however, is not perfect (56,65). The age of distribution lies mainly in the range from 4 to 16 years.
Computerized analysis by Lemieux and Blume (66) has also shed light on the spatial distribution of the spike-wave complex: the field distribution of spikes differed from that of the ensuing slow waves. Spikes “arose in one frontal region and propagated to the homologous part of the other frontal region with a peak-to-peak interval less than 15 msec. Less commonly, spikes first moved anteriorly within the initiating frontal region before contralateral propagation occurred.” By contrast, negative slow waves were more diffuse, more symmetrical in evolution, and more posteriorly centered than the spikes.
The onset of the spike component in one frontal lobe is congruent with the earlier findings of Cohn (56) and Lüders et al. (67), who noted asynchronies ranging from 5 to 20 msec. The frontal lobe is a large structure and subdivisions are necessary. Onset of spike-wave activity (and, in particular, of the spike component) over the frontal midline means in reality that a frontal portion in the immediate vicinity of the interhemispheric fissure is the origin of the discharge; this area belongs to the supplementary motor zone.
What are the neuropsychological correlates of subclinical generalized spike-wave bursts and clinical absences with spike waves? In this context, it should be pointed out that the impact of apparently subclinical 3 Hz spike-wave bursts might be stronger than one would expect from routine clinical observation. With its maximum over the frontal midline (frontal lobe close to interhemispheric fissure), there might be some impact on prefrontal lobe functions and, in particular, on the working memory. This concept of working memory, introduced by Baddeley (68) and enormously refined by Fuster (69,70), implies a system of constant perceptory afferences to the prefrontal dorsolateral region whence motor impulses emanate, resulting in readiness (set) for motor action. This system could
be blocked for a moment by a few (even by a single) spike waves and interfere with the working memory. In a clinical 3 Hz spike-wave absence, blocking of the working memory might be the most likely explanation for the mild disturbance of consciousness and its immediate full recovery with the termination of the attack (71). No “true disturbance of consciousness”— epileptic or nonepileptic—can recover immediately. In other words, the function of working memory is simply suspended for the duration of the spike waves.
Due to the uniqueness of primary generalized epilepsy with 3 Hz spike waves in the human (72), animal models for this response have great limitations. The arrest reaction of the monkey (73) produced by alumina cream injection into the fronto-orbital cortex has some resemblance to the human absence epilepsy; this cannot be said about the sudden freeze of motility noted in the cat stimulation of the cat’s mesial anterior thalamus (74); there is no spike-wave activity associated with this type of arrest reaction.
According to Sperling and Skolnick (75), there is a general decrease of cerebral blood flow (133 xenon method) during human generalized spike-wave activity, which, however, is less pronounced in frontal regions than in the parietal lobes. Transcranial magnetic stimulation of the motor cortex in patients with primary generalized epilepsy and 3 Hz spike waves produced motor evoked potentials of significantly reduced size when the cortical stimulus was time locked to the slow wave component of the spike-wave complex (but slightly reduced or unchanged when the stimulus was time locked with the spike) (76). This underscores the special inhibitory character of the slow wave component, which has been known since the work of Jung and Toennies (58).
Spike-Wave Complex (Slow 1 to 2.5 Hz)
After the demonstration of the 3 Hz spike-wave complex and its relationship to the absence seizure (38), the Gibbses and William G. Lennox were struck by the occurrence of slow spike-wave complexes in a much different type of patients with seizures other than the petit mal absence. The classical 3 Hz spike-wave complex was termed petit mal discharge. Consequently, the slow spike-wave complex was termed petit mal variant discharge. This term was used first by Gibbs et al. (77). In that study, hypoglycemia was induced in order to demonstrate an increase of classical 3 Hz spike-wave activity in the hypoglycemic state; an
increase of slow spike-wave activity, however, was not elicited with this activation method.
increase of slow spike-wave activity, however, was not elicited with this activation method.
![]() Figure 26.9 Generalized slow spike-wave complexes (mostly around 2 Hz) in a child with severe epileptic seizure disorder (Lennox-Gastaut syndrome). |
The wave morphology of this pattern varies considerably. In the majority, the complex consists of a rather slow spike (according to the definition, a sharp wave, lasting 70 msec or longer) and a slow wave. In a sizable number of cases, true spikes (60 msec or less duration) are followed by a slow wave (Fig. 26.9).
The spatial distribution is, in most instances, quite similar to that of the 3 Hz spike-wave complex. In the vast majority of the cases, the bursts are bilateral or generalized synchronous with imperfect synchrony and a frontal midline maximum is the rule in these discharges. Lateralization or occasional focal occurrence is sometimes observed, usually in children with severe residual brain damage in certain areas where parenchymatous destruction abolishes the spike-wave discharge.
Generalized slow spike-wave discharges are often quite prolonged. In some children or adolescents, the entire sleep portion (light and moderately deep non-REM sleep) consists of unabated generalized slow spike-wave activity. The diagnosis of an “electrical status epilepticus” may or may not be justified in such cases; there are usually no behavioral or polygraphic characteristics to suggest an ongoing ictal event. In some cases, the slow spike-wave activity is found only in non-REM sleep.
Although the classical 3 Hz spike-wave complex is seldom seen before the age of 4 and almost never before 3.5 years, its slow counterpart appears much earlier, sometimes before the age of 6 months. At this early age, the frontal maximum may not be readily demonstrable.
The slow spike-wave complex is almost always associated with a severe and often uncontrollable type of childhood epilepsy (seldom with onset between ages 11 and 20 years) called Lennox-Gastaut syndrome (40,44,46,77,78). Most of these children have a mixed seizure disorder and mental retardation.
Thus, the distinction between slow (1 to 2.5 Hz) and classical (3 Hz) spike waves is of great clinical significance. One has to
keep in mind, however, that many children or adolescents with slow spike waves also show series of 3 Hz or even 4 Hz spikewave complexes; the presence of these spike-wave types is obviously of no significance in such cases and what counts is the slow type. On the other hand, patients with classical 3 Hz spike-wave complexes and a usually benign type of epileptic seizure disorder almost never show slow spike waves except when a 3 Hz or 4 Hz spike-wave burst may slow down to 2.5 Hz at the end.
Spike-Wave Complex (Fast 4 Hz, 4 to 5 Hz)
This pattern is closely related to the classical 3 Hz spike-wave complex; both discharge types are most commonly lumped together. A differentiation, however, is justified on clinical grounds.
Gastaut (79) deserves credit for an outline of the distinctive features between the 3 Hz and the 4 Hz (or 4 to 5 Hz) spikewave discharge. According to his investigations, the fast spikewave burst is usually of shorter duration (1 to 3 sec), it occurs in patients older than 15 years, the bursts are always subclinical, and the associated seizure disorder is usually characterized by myoclonic jerking, grand mal attacks, or a combination of both seizure types, whereas absence seizures are quite uncommon. Paroxysmal flicker responses are common in such patients. A positive family history of epileptic seizures disorder is frequently found in this group of patients.
The 4 Hz spike-wave discharge is spatially distributed in the same manner as the 3 Hz spike-wave discharge; the frontal midline maximum is quite prominent.
The 6 Hz Spike-and-Slow-Wave Complex (Phantom Spike and Wave, Wave and Spike Phantom)
This is now considered a pattern of uncertain clinical significance, or a normal variant (marginal paroxysmal pattern), described by Gibbs and Gibbs (37): “The spike in this pattern has a strong positive component but the entire wave complex looks in general like a miniature reproduction of the 3 per second spike and wave of petit mal.” Because of the positive spike component, Silverman (80) stressed the similarity to the 6 Hz component of the 14 and 6 Hz positive spike burst and reported a transition from the former to the latter with deepening drowsiness. Marshall (81) introduced the term wave and spike phantom, which has found but limited acceptance.
The clinical correlates of 6 Hz spike-wave complex have been elucidated by Thomas (82), Gibbs and Gibbs (83), Hughes et al. (84), and Thomas and Klass (85). It is usually a pattern of adulthood but may also occur in adolescents and children. About 50% to 60% of the patients suffer from indubitable epileptic seizures, mostly generalized tonic-clonic seizures; the remainder show a history of syncopal attacks, posttraumatic states, or (as emphasized by Small in Ref. 86) psychiatric problems.
The 6 Hz spike-wave complex is an uncommon but not rare pattern (about 0.5% to 1% in a central EEG laboratory).
The discharge may be recorded in waking state, drowsiness, and light non-REM sleep; light drowsiness appears to be the optimal recording condition (Fig. 26.10).
Investigations regarding the spatial distribution of this pattern have led to an important dichotomy. Hecker et al. (87)
pointed out a distinction between a frontal versus an occipital accentuation. The frontal type is most commonly associated with epileptic seizure disorders and sometimes found in combination with other epileptiform discharges. The occipital types are found predominantly in patients with no evidence of epileptic seizure disorder.
pointed out a distinction between a frontal versus an occipital accentuation. The frontal type is most commonly associated with epileptic seizure disorders and sometimes found in combination with other epileptiform discharges. The occipital types are found predominantly in patients with no evidence of epileptic seizure disorder.
In a study of 839 patients with 6 Hz spike-wave complexes, Hughes (88) placed particular emphasis on the distinction between frontal and occipital maximum. He summarized the principal features of both forms by the acronyms WHAM and FOLD:
W: waking record | F: females |
H: high in amplitude | O: occipital |
A: anterior | L: low in amplitude |
M: males | D: drowsy record |
An interesting sidelight of this observation is the uneven gender distribution, which is rather unique; no other EEG abnormalities show any gender preference.
According to Hughes (89), patients with anterior 6 Hz spike waves are more likely to have epileptic seizures, and those with posterior discharges tend to have neuroautonomic disturbances.
Kocher et al. (90) were struck by the occurrence of 6 Hz spike-wave complexes in the abstinence or withdrawal phase of drug-dependent individuals. Tharp (91) produced the discharge by intravenous diphenhydramine (50 mg) in normal subjects. Hecker et al. (87) found that the occipital form of the 6 Hz spike-wave complex is often related to drug dependence (hypoanalgesics, barbiturates) and withdrawal. All this underscores the need for a distinction of the two forms. Thus, the frontal form will have to be regarded as a truly epilepsy-related paroxysmal pattern, whereas the occipital form finds itself on the fringe of paroxysmal discharge types.
Patterns of Uncertain Clinical Significance, or Benign EEG Variants, Benign Epileptiform Variants, and Marginal Patterns
The just-mentioned 6 Hz spike-wave discharge is considered a “benign EEG variant and pattern,” which include patterns with an “epileptiform morphology.” According to Westmoreland (92), the 6 Hz spike-wave discharge is not a reliable indicator of seizures (also see Refs. 85,93). Other patterns listed under the heading of benign patterns are small sharp spikes, 14 and 6 Hz positive bursts, wicket spikes, and the rhythmic temporal theta bursts of drowsiness (psychomotor variant pattern).
According to Westmoreland, these patterns “have an epileptiform appearance but are considered normal or benign variants of EEG activity or activity of uncertain significance.” These patterns occur in the healthy individual and there is no doubt that various EEG abnormalities may occur in someone who functions normally (48,49,94). Although these may be associated with potential epileptogenicity, they should be interpreted with caution, especially if the only finding on the EEG. Westmoreland (92) suggests that “critical evaluation of the various types of EEG activity is important.” From a single laboratory in London, Ontario, benign epileptiform variants occurred in 3.4% of 1183/35,249 recordings (95).
Rudimentary Spike-Wave Complex (“Pseudo Petit Mal Discharge”)
Gibbs and Gibbs (83) described a paroxysmal discharge that consists of generalized or nearly generalized high-voltage 3 to 4 Hz waves “with a poorly developed spike in the positive trough between the slow waves, occurring in drowsiness only.” This pattern is most prominent over parietal areas and was found only in infancy and early childhood with hypnagogic hypersynchrony. This hypogognic hypersynchrony can occur in generalized bursts rather than in prolonged stretches. These bursts, sometimes with a frequency as slow as 3 to 4 Hz, may contain small spike elements that justify a term like rudimentary spike waves. The Gibbsian term pseudo petit mal discharge suggests a relationship to spike-wave discharges and absence epilepsy. However, this pattern should not be equated to a specific epileptiform discharge and a transition into a classical or other spike-wave pattern does not occur (83). A history of febrile illness is very often found in these children with rudimentary spike waves and a history of febrile convulsions is also quite common (83,96).
Small Sharp Spikes
Small sharp spikes, also known as benign epileptiform transients of sleep (BETS), were delineated by Gibbs and Gibbs (37,83). It is the most inconspicuous paroxysmal discharge and hence is easily overlooked. The main negative and positive components are of about equally spiky character (Fig. 26.11), it is fairly widespread but seen chiefly over temporal and frontal areas, either shifting from side to side or synchronously firing. The pattern is almost exclusively found in drowsiness and/or light non-REM sleep. It is essentially a pattern of adulthood with a peak between ages 30 to 60 years; occurrence in adolescence and old age is somewhat less common. It is virtually absent in the first 10 years of life.
Koshino and Niedermeyer (97) found a prevalence of 1.36% with a peak of 2.9% between ages 30 and 40. In this study, two thirds (67.4%) of the patients had a history of epileptic seizures; this underscores the paroxysmal character of the discharge. From Ontario, small sharp spikes occurred in 1.85% of EEG recordings (95).
Small sharp spikes sometimes are precursors of typical anterior temporal spikes or sharp waves, which, in such patients, simply occur somewhat later in drowsiness or sleep. This association was also noted by Gibbs and Gibbs (83). In a depth EEG study by Westmoreland et al. (98), an extensive generator area of small sharp spikes was found. Among people without epilepsy, the discharges may occur in patients with cerebrovascular disorder, syncopal attacks, and psychiatric problems. Small (86) and Small et al. (99) have placed special emphasis on the occurrence of this spike discharge in a psychiatric population and especially in patients with manic-depressive illness.
It is interesting to note that Gibbs and Gibbs (83) found a rather high prevalence of small sharp spikes in presumed normal adult control subjects, reaching 7.9% in the range from 40 to 49 years. Reiher and Klass (100), Lebel et al. (101), White et al. (102), Klass and Westmoreland (93), and Gutrecht (103) have stressed the nonspecific nature of this pattern and do not regard its occurrence as an abnormality. By contrast, Low et al. (104) as well as Hughes and Gruener (105) have pointed out that small sharp spikes are not a normal or meaningless pattern. The data of these authors clearly show that this pattern indicates “a moderate degree of epileptogenicity” (105). This has been strongly confirmed by Saito et al. (106) and also by Molaie et al. (107). This is another indication of the inherent epileptogenic potentialities in so-called benign patterns.
Needlelike Occipital Spikes of the Blind
Spike discharges of a particularly fast and needlelike character develop over the occipital region in most congenitally blind children (83,108,109). These spikes are completely innocuous, unrelated to epileptic seizure disorder, and probably due to a state of functional deafferentation (110). The discharges disappear during childhood or adolescence.
The 14 and 6 Hz Positive Bursts (Fourteen and Six Positive Bursts)
In the IFSECN (8), “Fourteen and Six Hz Positive Bursts,” are defined as: “Bursts of arch shaped waves at 13 to 17 Hz and/or 5 to 7 Hz, most commonly at 14 and/or 6 Hz, seen generally over the posterior temporal and adjacent areas of one or both sides of the head during sleep. The sharp peaks of its component are
positive with respect to other regions.” For these bursts, the amplitudes are generally below 75 µV, the pattern is best demonstrated by referential recording using contralateral ear lobes or other remote reference leads, and the clinical significance is controversial. The synonymous term ctenoids was introduced by Lombroso et al. (111) with regard to the comblike shape. Contrary to Dutertre’s (10) statement, “mitten” is not a synonymous term.
positive with respect to other regions.” For these bursts, the amplitudes are generally below 75 µV, the pattern is best demonstrated by referential recording using contralateral ear lobes or other remote reference leads, and the clinical significance is controversial. The synonymous term ctenoids was introduced by Lombroso et al. (111) with regard to the comblike shape. Contrary to Dutertre’s (10) statement, “mitten” is not a synonymous term.
The 14 and the 6 component may be observed independently. According to Gibbs and Gibbs (83), the 6 Hz component prevails in early childhood and adulthood, while the 14 Hz component is more prominent in older children and adolescents. Hughes (112,113) emphasized the harmonic character of the major frequencies and preferred the term 14 and 7 Hz positive spikes (Fig. 26.12). From Ontario, 14 and 6 positive bursts occurred in 0.52% of EEG recordings (95).
This pattern was described first by Gibbs and Gibbs (114) and regarded as “evidence of thalamic and hypothalamic epilepsy.” The following 15 years produced exciting correlations to autonomic nervous system dysfunctions and behavioral disorders (115, 116, 117, 118, 119, 120, 121, 122, 123, 124, 125 and 126). Enthusiasm flagged when it became clear that this pattern is too often found in healthy individuals (111,127). Little (128) even presumed that this pattern “is more likely to be a sign of health than of disease.”
The “14 and 6 pattern” may be confined to the regions lying beneath a skull defect (129) and hence could denote a “hidden pattern” that is not detectable from the scalp unless it reaches major proportions.
The pattern occurs most commonly in children after age 4 and adolescents and declines in adulthood; Gibbs and Gibbs (83,130) recorded the 14 and 6 Hz activity even in infants. Its occurrence in the waking state is exceptional; it typically occurs in deep drowsiness and very light non-REM sleep, although deep sleep might be more conducive in very young children (83). Okada et al. (131) noted 14 and 6 Hz positive bursts in association with simultaneous negative spikes over the frontal area (suggestive of dipole formation).
Little is known about origin and neurophysiologic discharge demonstrated in depth recordings at the thalamic as well as the basal ganglia level (132); the discharge was also repeatedly recorded in the amygdaloid complex.
Much of the controversy about this pattern has arisen from differences of recording techniques and the use of routine sleep tracings and, in particular, the length of the sleep portion. The prevalence used to range from 10% to 30% at the age of 10 to 25 years, but there has been an unexplained marked decrease of the prevalence of “14 and 6” Hz discharge according to Gibbs (133).
We interpret the 14 and 6 Hz positive burst itself as “within normal limits,” unless it is extremely frequent and pronounced; then it is regarded as minimally abnormal. It appears to be a marginal pattern of no or very little epileptologic significance and proven cases of epileptic seizures very seldom have a 14 and 6 Hz positive burst as the only significant finding.
However, the 14 and 6 Hz positive burst may occur in the advanced states of metabolic encephalopathies, especially in hepatic coma (134,135). Drury (136) reported periodic 14 and 6 Hz positive bursts in 7/121 children with hepatic coma, mostly in association with Reye’s syndrome. All cases had a severe background EEG abnormality; these bursts were activated by stimulation in four children, and disappeared on follow-up recordings in six. The “14 and 6” pattern may also occur as a transient toxic effect of diphenhydramine hydrochloride (Benadryl).
Rhythmic Temporal Theta Burst of Drowsiness, Rhythmic Midtemporal Discharges, or Psychomotor Variant
This pattern was widely known as “psychomotor variant discharge” (37,83) because of certain similarities to rhythmical ictal activity occurring in psychomotor seizures (temporal lobe seizures, complex partial seizures). This term has been discouraged by IFSECN (8), which recommended the term rhythmical theta bursts of drowsiness. The term rhythmic midtemporal discharge of Lipman and Hughes (137) is also widely used.
The pattern consists of long runs of rhythmical activity in the range of 5 to 6.5 Hz with a maximum over the midtemporal region, often with considerable spread into posterior temporal, anterior temporal, and occipital areas. The theta activity shows a well-defined negative sharp component (Fig. 26.13) that infers the epileptiform character of the discharge. These trains of sharp theta waves may occur in a unilateral, bilateral shifting, or synchronous distribution type. The duration of a single run usually exceeds 10 seconds and may reach 1 minute or more. Very often, the first run is noted in early drowsiness; in deep drowsiness and light sleep, the pattern tends to disappear. According to Egli et al. (138), patients with rhythmical theta bursts show shortened periods of REM sleep and also, to a lesser degree, of slow sleep in nocturnal sleep studies at the expense of log drowsy periods with the rhythmic theta pattern. Hughes (139) has emphasized that the rhythmic midtemporal discharge is not limited by the drowsy state and may be very active in wakefulness.
This pattern is rare. Egli et al. (138) found a prevalence of 0.1% in a large laboratory, and Santoshkumar et al. (95) in 0.12% of EEG recordings. Although occurring mainly in younger or middle-aged adults, it is also seen in adolescents and children.
The clinical significance is not clear. Despite its strongly epileptiform appearance, its epileptogenic properties seem to be very low; most patients have no history of clinical seizures, although Lipman and Hughes (137) found seizures in 36% of their patients. Personality disorders and some autonomic nervous system dysfunctions are common in patients with this pattern (83,137,140). This view is shared by Eeg-Olofsson and Petersén (1982; cited in Boutros et al. (141)), who also studied the genetic transmission of this pattern and wondered about possible autosomal-dominant inheritance.
The clinical significance is not clear. Despite its strongly epileptiform appearance, its epileptogenic properties seem to be very low; most patients have no history of clinical seizures, although Lipman and Hughes (137) found seizures in 36% of their patients. Personality disorders and some autonomic nervous system dysfunctions are common in patients with this pattern (83,137,140). This view is shared by Eeg-Olofsson and Petersén (1982; cited in Boutros et al. (141)), who also studied the genetic transmission of this pattern and wondered about possible autosomal-dominant inheritance.
Subclinical Rhythmic EEG Discharge of Adults (SREDA)
This pattern was originally described by Naquet et al. (142) as a “paroxysmal discharge of the temporo-parietooccipital junction,” facilitated or triggered by temporary hypoxic conditions. In spite of certain epileptiform features and its rhythmical sharp character, the possibility of an epileptic (ictal) event has been strongly de-emphasized. Herranz and Lopez (143) have described this pattern as “subclinical paroxysmal activity” in 31 patients and stressed its rarity; it has an incidence of 0.02% to 0.045%. From Ontario, SREDA occurred in 0.07% of EEG recordings (95). The authors have seen this pattern once in a child.
Westmoreland and Klass (144,145) and Miller et al. (146) deserve particular credit for the elucidation of this discharge type. These authors disagree with the view of Naquet et al. (142) concerning the major role of cerebrovascular insufficiency and hypoxia, although the average age of their patients was 61 years. The term “SREDA” has been proposed by Miller et al. (146). O’Brien et al. (147) analyzed this pattern in seven recent patients and only one of them showed evidence of cerebrovascular disorder.
This pattern occurs mainly in the waking state or in light drowsiness. The onset may be fairly abrupt with widespread sharp rhythmical theta (4 to 7 Hz) and occasionally with delta activity. In other cases, the onset is gradual with a few single sharp discharges that increasingly develop rhythmical character, first at delta, then at theta frequency. As to the spatial distribution, a
maximum of this discharge is usually found over the centroparietal region and especially over the vertex. Hyperventilation may induce this pattern.
Periodic or Quasiperiodic Discharges
These rhythmically (periodic), or nearly rhythmically (“quasiperiodic”) recurring discharges are of an epileptiform character, but are not usually associated with chronic epilepsy. Periodic discharges occur with a specific interval duration and quaiperiodic discharges occur with a varying interval duration. These are an important EEG feature of a severe ongoing central nervous system (CNS) disorder. They are hence disease-suggestive and sometimes virtually disease-specific, rather than suggestive of epilepsy in general. A review of periodic activities was presented by Gaches (148) as well as by Bauer and Pieber (149). The periodic character of these patterns remains enigmatic. This rhythmical firing is markedly different from the predominantly random character of interictal seizure discharges (spikes, sharp wave, etc.). There is no satisfactory model to explain the periodicity of the discharges.
Periodic discharges are always of large amplitude, mostly in the range of 100 to 300 µV. Morphologically, these may be simple sharp waves, usually with a duration exceeding 150 msec, but may be a compounded waveform. Periodic discharges may be focal, widely scattered, or generalized synchronous. A fine tabulated review of periodic discharges has been presented by Spehlmann (150). Periodic discharges are differentiated by the interval: either long-interval or short-interval periodic discharges.
Periodic Complexes in Subacute Sclerosing Panencephalitis (SSPE)
SSPE is now exceptionally rare in the developed world following measles immunization. Periodic complexes dominate the second state of SSPE, a prolonged phase in which the clinical diagnosis is usually made. This type of complex discharge almost never occurs in other clinical conditions and is hence almost disease-specific. It is a very reliable and highly contributory diagnostic finding in this disease. The discharge duration varies from 0.5 to 3 seconds and are typically formed by two or more waves with mean amplitude of 500 µV (100 to 1000 µV) (150, reaching as high as 1400 µV (11)). The enormous discharge amplitude might be confused with movement artifact, which is made more confusing by the associated myoclonus or sudden loss of tone.
The periodicity develops with disease progression. The earlier stages are usually dominated by diffuse 1 to 3 Hz activity of fairly rhythmical character, but the compounded discharge may be aperiodic. Sometimes, the periodic discharge is present in the earliest stage of the disease and may persist to the fatal outcome; more often, this pattern disappears in the terminal or third stage. The discharge is more impressive in the waking state (Fig. 26.14). The elements of the discharge are variable; a giant slow wave is usually mixed with several sharp waves. The discharge is generalized, with a maximum over the frontocentral areas and vertex. The frequency of the complexes ranges from 4 to 16 Hz (151). This is an example of a long-interval periodic discharge.
Accompanying myoclonus is fairly synchronous with the discharge; the motor activity may precede or trail the discharge
in a range of 200 to 800 msec. There may be minor interhemispheric asynchronies (15 msec) and an earlier appearance in parietooccipital areas than in frontocentral areas, where the discharge shows its most impressive voltage (152).
in a range of 200 to 800 msec. There may be minor interhemispheric asynchronies (15 msec) and an earlier appearance in parietooccipital areas than in frontocentral areas, where the discharge shows its most impressive voltage (152).
The background activity between the complexes is quite variable. The background may be normal with posterior alpha rhythm; however, this is exceptional; severely disordered background activity is most commonly found. Slow activity prevails and numerous spikes are sometimes recorded, especially over anterior areas. Spike-wave complexes may also be present; even clinical absence seizures with classical 3 Hz spike-wave complexes have been observed (153). A case of acute fulminant SSPE with only 2 months’ duration was reported by Gökcil et al. (154); the EEG showed very pronounced discharges.
Periodic discharges mimicking SSPE have been reported by Giovanardi Rossi et al. (155) in children with myoclonic juvenile epilepsy of nonprogressive character. These discharges, however, were confined to ictal episodes. Most unusual is the observation of Prier et al. (156): convincing periodic complexes of the aforementioned type in an indubitable case of multiple sclerosis (age 15 years).
The origin of the periodic SSPE discharge is controversial. Radermecker and Poser (157) have proposed a deep thalamic or mesencephalic-reticular origin; this view has been supported by Cobb (158,159), who performed depth EEG recordings, and by Lombroso (160), who studied direct current (DC) potential changes during the periodic discharge. Other authors favor the idea of a cortical origin (161, 162 and 163).
In an autopsy-confirmed case of SSPE, Martinovic (164) demonstrated periodically occurring generalized high-voltage bursts firing at a rate of 11 to 13 Hz with a spiky contour. There was a repetition interval of 27 to 28 seconds. This most unusual pattern was gradually replaced by the conventional type of periodic discharges.
Periodic Complexes in Herpes Simplex Encephalitis
In this extremely severe but not invariably fatal necrotizing encephalitis, slow repetitive spikes have been noted by Radermecker (165). These observations were subsequently confirmed by Millar and Coey (166), Perier et al. (167), Carmon et al. (168), Rawls et al. (169), Adams and Jennett (170), Upton and
Gumpert (171), Gaches and Arfel (172), Elian (173), Kugler et al. (174), and Cobb (175). The discharge was also found in experimental disease inoculated rabbits by Gupta and Seth (176).
Gumpert (171), Gaches and Arfel (172), Elian (173), Kugler et al. (174), and Cobb (175). The discharge was also found in experimental disease inoculated rabbits by Gupta and Seth (176).
In an earlier stage, local mostly unilateral temporal polymorphic delta waves are the most striking feature, but soon large sharp waves emerge over the most affected region. These discharges usually fire at intervals from 2 to 4 seconds. This is a short-interval periodic discharge. The discharge may be quite slow and exceed 1000 msec; consider that a sharp wave is defined as having a duration from 70 to 150 msec. The amplitudes are in the range from 100 to about 500 µV. Similar periodic sharp discharges have been demonstrated in neonatal herpes simplex encephalitis (177). In the course of the disease, the originally regional discharge tends to become generalized synchronous; asynchronous bilateral discharges may also occur. Predominantly occipital localization of the periodic discharges has been reported by Bergey et al. (178).
In contrast with the long-interval periodic complexes found in SSPE, the periodic discharge of herpes simplex encephalitis has a short interval, with the longest intervals of 4 seconds or less; according to Gaches (148), this criterion is always met in the herpes simplex discharge. In some cases, the periodic discharge can be detected only when almost daily repeat records are carried out. This explains the fact that periodic discharges are not reported in all cases of herpes simplex encephalitis.
Periodic Discharges in Creutzfeldt-Jakob Disease
The clinical and neuropathologic features of this subacute disease were established by the work of Creutzfeldt (179), Jakob (180), and Kirschbaum (181,182). A very similar syndrome known as spongiform encephalopathy (183) and a special variant described by Heidenhain (184) were eventually found to be the same disease. Although originally presumed to be a degenerative CNS disorder, a slow virus was eventually demonstrated as the causative agent (185,108). These investigators transmitted this virus to the chimpanzee with intracerebral inoculation and produced a similar invariably fatal disease. It is interesting to note that chimpanzees with the full clinical picture of Creutzfeldt-Jakob disease showed prominent delta activity in their EEG but neither spikes nor periodic discharges (186).
The periodic discharge consists of a sharp wave or a sharp triphasic complex of 100 to 300 msec duration with a repetition rate of 0.5 to 2 Hz or intervals of 500 to 2000 msec. This is also a short-interval periodic discharge. The discharges occur against a severely disordered background of activity, mostly in generalized synchrony. Some cases show unilateral onset with discharges over one hemisphere or lateralized to one hemisphere for several days or weeks (187). According to Tariska et al. (188), the periodic discharges may even remain unilateral in the course of the disease.
The periodic activity usually shows a maximum over the anterior region except for the Heidenhain form, which has a posterior maximum (Fig. 26.15); in this special form, cortical blindness is a common feature. In sleep, the periodic discharges tend to disappear. The absence of periodic discharges after 10 weeks of illness militates against Creutzfeldt-Jakob disease (189). EEG studies of Chiofalo et al. (190) are based on the observation of 27 cases. The differential diagnosis of the combined clinical and electroencephalographic picture of this disease is quite complex (191).
Myoclonus is a typical clinical feature of this disease. The periodic patterns may or may not be associated with myoclonus (192).
In addition to periodic discharges, cyclic changes may also occur, and the periodic activity may get temporarily replaced by diffuse slow activity in the delta and theta range (193).
The periodic EEG discharge of Creutzfeldt-Jakob disease may be caused by other conditions in rare instances. Hypothyroidism is mentioned as a treatable cause of this pattern.
It has to be emphasized that the periodic discharges of Creutzfeldt-Jakob disease (i) do not occur in every case of this disease (even with numerous repeat-recordings) (194) and (ii) may occasionally be found in other disorders.
Periodic Lateralized Epileptiform Discharges (PLEDs)
Chatrian et al. (36) gave an extensive account of periodic lateralized or focal discharges that may occur in a variety of acute neurologic conditions. This pattern is most often associated with acute cerebral infarctions but may also occur in neoplastic, inflammatory, and epileptic disorders, especially status epilepticus. Periodic discharges occurring in herpes simplex encephalitis are discussed earlier in this chapter. PLED activity, however, may also occur in other types of encephalitis—among others, in infectious mononucleosis encephalitis, according to Aminoff et al. (195). As to acute vascular pathology, watershed-type
infarctions are the most common structural substratum in patients with PLED. Markand and Daly (196), Dauben and Adams (197), and Bauer et al. (198) presented further important work on this pattern, which also plays a major role in experimental cerebral embolism (199).
infarctions are the most common structural substratum in patients with PLED. Markand and Daly (196), Dauben and Adams (197), and Bauer et al. (198) presented further important work on this pattern, which also plays a major role in experimental cerebral embolism (199).
PLEDs may be simple and large sharp waves or complex (compounded) discharges with mixed spiky and slower elements. The amplitudes are usually around 100 to 300 µV, but may occasionally be much higher. The firing rate may be as fast as 3 Hz or as slow as 12/min (36) (Fig. 26.16).
The discharge is found over the maximally involved area, and the local background activity is almost always severely disordered. The focal maximum is customarily located over the boundary zone between middle and posterior cerebral arteries and thus over the posterior temporal region and its immediate vicinity. Watershed infarctions are seldom found between the territories of middle and anterior cerebral arteries, with a maximum of PLED activity over the superofrontal region.
The term PLED implies lateralization. It is possible, however, that PLED activity is generated independently over both hemispheres. De la Paz and Brenner (200) have investigated the bilateral type of PLED referred to as “BI-PLED” (also “bi-PLED”). Although strokes were found to be the leading cause in the PLED group, CNS infection and epileptic seizure disorders, especially status epilepticus, predominated in the BIPLEDs group. Bilateral synchronous (generalized synchronous) periodic activity should not be listed as BIPLED in view of the original definition of Chatrian et al. (36). This distinction, however, is not generally observed (201). Filley et al. (202) have broken down their large material of PLEDs into typical and “indeterminate” discharges. The term cerebral bigeminy has been used by Aldrich and Pugh (203) for bilaterally independent PLED activity. Even TRI-PLEDs, arising from three different areas, have been reported (left frontal, left posterotemporal, and right posterotemporal) in an 85-year-old patient with renal encephalopathy (204).
PLEDs are often associated with simultaneous focal motor twitching in the contralateral face or fingers, hand, arm, leg, foot, etc. This underscores the paroxysmal character of the pattern. It is usually a temporary pattern that changes into other abnormalities within 1 to 2 days. Patients with PLEDs are in most cases acutely ill and often show a history of a variety of mixed problems, such as cerebral arteriosclerosis plus renal insufficiency or chronic alcoholism or diabetes mellitus. The possibility of an underlying neoplasm must also be considered.
Patients with PLEDs are commonly older adults; this pattern occasionally occurs in young adults and children (205). Andriola (206) noted PLEDs as well as BIPLEDs in 12 children with various types of acute CNS disease; those with bilateral activity (BIPLED) expired in the acute state. Ritaccio and March (207) reported the association of BIPLEDs with complex partial status epilepticus. Silbert et al. (208) described another variant of PLEDs with independent ipsilateral periodic lateralized discharges. A clinical curiosity appeared to be the observation of Chabolla et al. (209): a young man suffering from multiple sclerosis (MS) with exacerbations ushered in by complex partial status epilepticus associated with an enhancing right frontal MRI lesion and PLEDs in the EEG. Another report on PLEDs in MS (right temporal PLEDs and MRI lesion) has been presented by Gandelman-Marton et al. (210). We have seen PLEDs in a child with hemiplegic migraine following a seizure.
A very thoughtful study of PLEDs deserves special attention: a critical review by Pohlmann-Eden et al. (211). These authors consider PLEDs as “an EEG signature of a dynamic pathophysiologic state in which unstable neurobiologic processes create an ictal-interictal continuum, with the nature of the underlying neuronal injury, the patient’s preexisting propensity to have seizures, and the coexistence of any acute metabolic derangements all contributing to whether seizures occur or not.” Pohlmann-Eden et al. have strongly reemphasized the dominant etiologic role of strokes in the generation of PLEDs. From the evidence of functional neuroimaging studies, these authors feel that PLEDs “might reflect a key pattern for focal hyperexcitability in the penumbra zone of ischemic stroke.”
A nonlateralized variant of PLEDs are periodic epileptiform discharges occurring in the midline (PEDIM), as cited by Westmoreland et al. (212). These midline discharges are also presumed to arise from watershed areas: anterior and middle, middle, and posterior cerebral arteries.
Periodic Discharges in Acute Cerebral Anoxia
Repetitive simple or compounded sharp waves in generalized synchrony may occur against a flat (or at least seemingly flat) background of activity in patients with acute cerebral anoxia. Myoclonus is often found in association with this type of activity (213, 214 and 215). In most cases, these discharges are probably aborted bursts in a suppression-burst pattern. True periodic discharges, however, may also occur (Fig. 26.17).
Periodic Discharges of Other Etiologies
Periodic discharges may occasionally occur in other conditions, but such observations are rare. The presence of such discharges, whether regional or generalized, merely underscores the severity of the basic conditions. Figure 26.18 demonstrates periodic discharges over the right posterior quadrant in a 15-year-old girl with a history of Dandy-Walker syndrome, numerous shunt operations, shunt infections, and a severe epileptic seizure disorder. These rhythmical discharges also show the tempo of propagation over the right posterior quadrant of the cerebrum.
SPIKES AND OTHER PAROXYSMAL DISCHARGES IN HEALTHY CHILDREN
Focal or generalized paroxysmal discharges may occur in apparently healthy individuals in all age ranges. These findings may be termed as “false positives,” but the EEG abnormalities are real and such spikes are evidence of an underlying epileptic pathophysiology that may or may not subsequently become manifest. Let us contemplate the indubitable fact that a complete medical evaluation will yield certain abnormalities in almost every healthy individual. What the electroencephalographer needs in such cases is a commonsense philosophy as a basis for a wise interpretation. There are many examples of seemingly irrelevant
and yet unmistakably present abnormalities that the prudent, seasoned physician will integrate into a holistic view of the individual. Similarly, EEG does not differ from the rest of medicine.
and yet unmistakably present abnormalities that the prudent, seasoned physician will integrate into a holistic view of the individual. Similarly, EEG does not differ from the rest of medicine.
The EEG evaluation of comparatively large healthy populations usually shows a certain percentage of abnormalities. Thorner (216) showed paroxysmal discharges in 0.3% of 1100 flying cadets; Gibbs et al. (217) found epileptic abnormalities in 0.9% of normal adult controls; Harty et al. (218) observed such abnormalities in 3% of candidates for medical service; and Williams (219) noted “larval epileptic disturbances” in 9% of healthy controls. A study of 682 Air Force applicants showed paroxysmal changes in 2.6%; about one fourth were focal and the rest were of nonfocal character (220).
Zivin and Ajmone Marsan (221) and Chatrian (222) have contemplated the clinical significance of spikes in healthy persons. Above all, the interpretation must take into consideration age. In childhood, the occurrence of central-midtemporal (also parietal) spikes is associated with overt seizures in only 50% to 70% of the cases; this pertains mainly to the age from 3 to 12 years. In occipital spikes (mainly age 3 to 5 years), the epileptogenicity is even lower. Major studies on this subject based on large healthy populations were performed by Nekhorocheff (223), Kellaway and Fox (224), Brandt and Brandt (225), Corbin and Bickford (226), Trojaborg (227), and Eeg-Olofsson et al. (228). In general, “benign” focal spikes (such as seen in benign rolandic epilepsy) outnumber generalized synchronous bursts of spikes or spike waves by a slight to considerable margin.
The study in children by Cavazzuti et al. (229) demands special attention. EEG recordings were performed on 3726 children from 6 to 13 years of age without evidence of seizure or neurologic deficits. Paroxysmal discharges were found in 131 cases (3.52%). Generalized, mainly polyspike, wave discharges were found in 41 cases, midtemporal spikes in 50, centroparietal spikes in 27, occipital spikes in 2, and bilateral (midtemporal or parietal) spikes in 11 children. Changes in spike location and shifts from focal to generalized discharges were rare. The follow-up period was up to 9 years. The abnormalities disappeared in most, usually during elementary school age or in early adolescence. Only seven children developed clinical epileptic seizures; five had generalized discharges, one had midtemporal, and one had centroparietal spikes. In two subjects there was evidence of epileptic seizures in the family, while six children had siblings with spikes, either generalized or rolandic.
Both generalized synchronous (spike wave, polyspike wave) and rolandic (centroparieto-midtemporal) spikes in nonepileptic children suggest a genetic predisposition if no neurologic deficit and no history of insult to the CNS are present. In children with a history of cerebral palsy and with no seizures but prominent spiking, the spike activity may herald future epileptic seizures (83). Even in perfectly healthy children with spikes, the possibility of future seizures cannot be completely ruled out. In addition, epileptiform abnormalities may be an
epiphenomenon associated with an underlying neurologic disorder, such as attention deficit disorder, headaches, or behavioral problems, and psychiatric disorders, to name a few. This section must be capped by a strong plea to clinicians to refrain from rash and ill-advised statements that a person without seizures has epilepsy and must be treated because of spikes in the EEG. There may be need for further medical attention, and in some circumstances a repeat EEG may be prudent but anticonvulsive treatment is seldom needed and indeed is ill-advised in most cases.
epiphenomenon associated with an underlying neurologic disorder, such as attention deficit disorder, headaches, or behavioral problems, and psychiatric disorders, to name a few. This section must be capped by a strong plea to clinicians to refrain from rash and ill-advised statements that a person without seizures has epilepsy and must be treated because of spikes in the EEG. There may be need for further medical attention, and in some circumstances a repeat EEG may be prudent but anticonvulsive treatment is seldom needed and indeed is ill-advised in most cases.
Types of Epileptic Seizures
The character of an epileptic seizure is more or less strongly determined by the chief cerebral area involved. Another important factor is the underlying basic epileptic condition. As a matter of fact, the underlying basic condition may be of paramount significance, especially in an infant with infantile spasms—hypsarrhythmia and jackknife seizures, which are not found outside this basic epileptic condition, or in a child with Lennox-Gastaut syndrome and atonic drop attacks, which are germane to this condition and alien to others (also see Ref. 230). These basic epileptic conditions are age-determined or agedependant; therefore, age is an important factor that is capable of modifying the character of epileptic seizures. Furthermore, the nature and extent of an underlying cerebral lesion may be a negligible factor in the determination of the seizure type in the group of age-determined epileptic conditions.
Certain types of seizures, such as focal motor and psychomotor seizures, are highly indicative of special cerebral areas, whereas others are of much less localizing value. The localization depends heavily on such confirming EEG evidence as local spikes and recorded ictal episodes and, in some special cases, on the demonstration of regional changes with depth electrodes. Structural neurodiagnostic tests are needed for the demonstration of significant morphologic changes.
Focal seizures may be a prelude to an ensuing grand mal convulsion. The term aura has been used for such initiating focal seizures, especially for attacks impinging on afferent systems (visual, acoustic, olfactory, somatosensory, gustatory, and so forth).
Simultaneous EEG recording and fMRI is beset with problems since the EEG is being obtained in a high-field magnet and during scanning. Baudewig et al. (231) have reported a method demonstrating simultaneous blood-oxygenation-dependent (“BOLD”) MRI sequences and EEG activity with very little artifactual distortion (in epileptic patients).
Classification of Epileptic Seizures
The classification of epileptic seizures is a thankless job. The preferences of “splitters” and “lumpers” must be reconciled in the tedious work of an international committee entrusted with this task by the International League Against Epilepsy, progressing from the older classifications of reputed epileptologists that have sometimes been marred by personal biases. The most commonly used classification had been proposed by the Commission on Classification and Terminology (232). Although widely used and officially recommended (and essen-tially reiterated by Dreifuss (233)), this classification did not find universal acceptance. This may have been due to an excess of terms and the use of cumbersome formulations. Also, in pediatric practice the classification required several modifications for practical application. It was not always possible to differentiate simple from complex focal seizures because immaturity, limited communication skills, and autistic features could prevent the accurate assessment of alteration of consciousness in some children (234). Infantile spasms were missing from the classification, as were myoclonic-astatic or myoclonic-atonic seizures. A breakdown into small ictal behavioral or ictal-EEG detail may render a classification more nebulous. There are, of course, limitations for every attempt at seizure-type classifications. The classification of 1981 could not accommodate a considerable number of seizures, which then were listed as unclassifiable because they did not fit the scheme. A recent recommendation from the ILAE taskforce on classification suggested a marked simplification of the seizure classification and tried to address some of these issues (235) (Table 26.1).
Generalized Tonic-Clonic Seizure (Grand Mal)
Clinical Manifestations
The sequence of clinical manifestations has been masterfully described by Gowers (236, see also republication in 1964) and is given in detail below. Further detailed descriptions were given by Janz (237), Gastaut and Broughton (238), and Karbowski (239). The generalized tonic-clonic (GTC) seizure lasts about 40 to 70 seconds, or sometimes up to 90 seconds. Initial massive
generalized tonic spasm is sometimes initiated with a cry (“a wild, harsh, screaming sound,” 236). It is immediately associated with loss of consciousness (profound coma); the arms are usually in semiflexion and the legs in extension. After about 10 to 20 seconds, it is supplanted by the clonic phase in which “the vibratory phenomenon (noted with palpation of the extremely tense muscles) becomes sufficiently prolonged to interrupt completely the tonic contraction” (238).
generalized tonic spasm is sometimes initiated with a cry (“a wild, harsh, screaming sound,” 236). It is immediately associated with loss of consciousness (profound coma); the arms are usually in semiflexion and the legs in extension. After about 10 to 20 seconds, it is supplanted by the clonic phase in which “the vibratory phenomenon (noted with palpation of the extremely tense muscles) becomes sufficiently prolonged to interrupt completely the tonic contraction” (238).
Table 26.1 Classification of Seizure Types | |||||||||||||||||||||||||||||||||||||||||||||||||||||||||||||||||||||||
---|---|---|---|---|---|---|---|---|---|---|---|---|---|---|---|---|---|---|---|---|---|---|---|---|---|---|---|---|---|---|---|---|---|---|---|---|---|---|---|---|---|---|---|---|---|---|---|---|---|---|---|---|---|---|---|---|---|---|---|---|---|---|---|---|---|---|---|---|---|---|---|
|
This leads to a succession of brief and violent flexor spasms of the entire body. Accompanying apnea leads to a grayish livid complexion, while the rhythmical clonic spasms slow down until a final massive myoclonus marks the end of the seizure. According to Gastaut and Broughton (238), tongue biting usually materializes during the clonic phase. Mydriasis, arterial hypertension, and tachycardia accompany the attack. Enuresis usually occurs at the termination of the seizure (238); there may occasionally be fecal incontinence. The patient is completely flaccid after the last clonic jerk, after which respiration returns. Only a few seconds after the beginning of the flaccid, immediate postictal phase, a tonic muscle spasm returns that is most intense and prolonged in the masseter (238). This postictal trismus temporarily blocks respiratory effect. The respiration becomes regular in the ensuing recuperative phase, during which the patient returns to consciousness unless he slips directly into a period of postictal sleep. A fall caused by a GTC can be traumatizing; even epidural hematoma has been reported (240).
Tonic-clonic seizures may occasionally show unilateral predominance of the clinical manifestations. Gastaut and Broughton (238) feel strongly that these attacks (which are more common in children and infants) are in fact generalized seizures with unilateral expression. Do attenuated or mitigated grand mal seizures exist? The answer is affirmative according to Karbowski (239). These attacks are characterized by shorter duration and rudimentary tonic or clonic phases, possibly due to the effect of anticonvulsive medication.
EEG Manifestations
The tonic-clonic seizure is initiated by an abrupt loss of voltage (desynchronization, electrodecremental period) of a few seconds duration; there is evidence of very fast (20 to 40 Hz) activity in all leads. In patients with primary generalized epilepsy, several generalized bursts of polyspike-wave complexes with massive bilateral myoclonus may precede the phase of desynchronization.
Muscle activity rapidly obscures the recording; the vertex derivation, however, may remain artifact-free due to the lack of underlying muscles. Informative recordings can be secured only from patients with muscle relaxation from curarization and artificial respiration. Removal of muscle artifact by means of digital filtering has been achieved by Gotman et al. (241).
After the phase of desynchronization, which may be as short as 1 to 3 seconds, rhythmical activity at about 10 Hz with rapidly increasing amplitude dominates the EEG. Gastaut and Broughton (238) have laid much stress on this frequency (“epileptic recruiting rhythm,” 242), which is better discernible with the use of automatic frequency analysis.
About 10 seconds after the onset of a seizure, slower frequencies are noted, gradually slowing into the theta and delta range. Once the frequency of 4 Hz is reached, “each slow wave interrupts the recruiting rhythm, giving rise to polyspikes and wave complexes, themselves decreasing in frequency” (238). The clonic activity corresponds with generalized polyspike bursts at each myoclonic jerk (Fig. 26.19).
The last clonic contraction is followed by postictal flatness for several seconds (243). Very slow irregular delta activity
(“postseizure stupor,” 37) then dominates the EEG, with gradual frequency increase into the theta and alpha band; the appearance of an organized posterior alpha rhythm signals the return to the waking state.
(“postseizure stupor,” 37) then dominates the EEG, with gradual frequency increase into the theta and alpha band; the appearance of an organized posterior alpha rhythm signals the return to the waking state.
Prevalence and Age Factor
Tonic-clonic seizures are common at any age, except for the first year of life, during which they are largely absent (244). Insufficient myelination of the brain probably precludes them at this early age. In the research of Gibbs and Gibbs (37), 5598 patients of a population of 11,612 people with epilepsy (48.2%) had grand mal only; another 3290 patients (28.3%) had grand mal in combination with other types of seizures.
Neurophysiologic Aspects
One is tempted to regard the tonic-clonic seizure as a standardized maximal and global epileptic response of the brain. Electroconvulsion studies in the cat, however, have shown that the cerebellum and the lower brainstem do not fully participate in the ictal activity (245). The view of a standardized all-out response of the brain requires some correction. Schmidt and Wilder (1968) feel that GTCs “vary in their severity and in the degree to which they incorporate the various tonic and clonic phases.” Variations of the degree of cerebral cortical participation in tonic-clonic seizures have been demonstrated in the cat as well as in the human by Rodin et al. (246). This view has been supported by the experimental findings of Petsche (247).
This implies that tonic-clonic seizures are graded rather than maximal cerebral epileptic responses. These variations could explain differences in the response to electroconvulsive therapy in psychiatric illness; following the electrically induced GTC, the EEG may show little or marked slowing, probably depending on the degree of neuronal participation in the seizure.
Quite different is the view of Gastaut and his coworkers. The emphasis placed on rhythmical 10 Hz activity during a large portion of the grand mal attack has been discussed; this “epileptic recruiting rhythm” will follow stimulation only in nonspecific reticular thalamic structures projecting diffusely to both hemispheres over still uncertain connecting pathways. It was felt that the motor, autonomic, and EEG phenomena of the tonic phase could be explained by massive discharge of the thalamic and subthalamic brainstem reticular structures; this would also account for the loss of consciousness. In the clonic phase, the appearance of slow waves has been attributed to cortical inhibitory systems via thalamic and lower brainstem structures or, more specifically, via a thalamocaudate circuit branched from the thalamocorticospinal system. These views have been laid down by Gastaut et al. (248), Gastaut and Fischer-Williams (242), and, in condensed form, by Gastaut and Broughton (238).
Absences (Petit Mal)
Terminology
The term petit mal arose from the jargon of physicians and attendants in the hospitals of Paris early in the 19th century. According to Temkin (249), Esquirol (250) distinguished more or less severe epileptic attacks as “grand mal” and “petit mal,” but his definition of these two terms “is obviously vague.” The
term absence was introduced by Calmei (251). The modern nomenclature (Gastaut et al., 1970) recommends the term absence, but the term petit mal is deeply rooted. Petit mal absence seems to be an acceptable compromise.
Clinical Manifestations
The petit mal absence occurs mainly in children older than 3 years, with a declining incidence throughout adolescence and early adulthood; persistence beyond middle adult life is very rare.
The attack consists of a sudden lapse of consciousness with impairment of mental functions. Its usual duration ranges from 5 to 20 seconds; longer absences may occur over 1 to 2 minutes, but such an unusual length is a sign of a somewhat complicated epileptic seizure disorder with some degree of automatism-like ictal behavior. The absence is associated with interruption of ongoing activity and, due to moderate impairment of consciousness, the patient is unable to see or hear. There is usually a blank facial expression that contradicts the frequently used term staring spells; a true stare is more likely to occur in temporal lobe epileptics with psychomotor seizures. The eyes drift upward (“star gazing,” 237,252); rhythmical beating of the eyelids at 3 Hz is very common and may be the only apparent motor manifestation of the attack. Marked orofacial movements during the absence are suggestive of a more complex type with poorer response to therapy.
Petit mal absences show a wide variety of mild-to-moderate motor accompaniment; rhythmical eye blinking in synchrony with the spike waves is the most common motor component. Retropulsion of the head is quite common (“retropulsive petit mal,” 237); adversive movements and some rhythmically repetitive oral motions may also occur. Automatisms occurred in 163/405 (40%) of children and were more likely with hyperventilation and with longer seizures, occurring in only 23% of spontaneous awake seizures (253,254). The most common clinical manifestations include swallowing, mouthing, chewing, lip smacking, and lip licking and occurred across all absence syndromes.
There is good reason to assume that the unique nature of loss of consciousness during the classical absence is based on a temporary suspension of the “working memory” due to the powerful accentuation of spike-wave activity in the frontal cortex (255, 256 and 257). This explains the unmatched immediate restoration of the working memory along with consciousness at the end of the absence (no other form of brief loss of consciousness—syncope, for instance—reverses so quickly). As to the crucial function of working memory, see Ref. 69.
The postural control is grossly maintained, but swaying and stumbling movements may be noted in the standing patient (Schmidt and Wilder, 1968). The posture may be altered by sustained or saccadic retroflexion of the head (“retropulsive petit mal,” 258). Autonomic and especially vasomotor changes are common during the petit mal absence (259,260).
Impairment of the level of awareness has been the object of some studies (260, 261, 262, 263, 264 and 265). A variety of tests, such as rhythmically pressing a button, have been used to demonstrate the lapse of consciousness (266). Mirsky and Tecce (267) demonstrated the persistence of visual evoked potentials during spike-wave discharges.
Absence seizures are divided into typical absence and atypical absence seizures. Typical absence seizures are classified as simple or complex; a simple absence has a sudden onset without motor activity; complex absences have associated motor activity or autonomic activity. In a typical absence seizure, it is usually not difficult for an observer to identify the electrographic seizure onset and the seizure cessation by clinical observation.
The atypical absence seizure has a less clearly defined onset or cessation, so that it may be difficult to identify the beginning or the end of the seizure without EEG. The atypical absence seizure may have more pronounced tone changes, a longer duration, and are typically associated with other seizure types and mental retardation. In contrast to the typical absence seizure, it is usually very difficult for an observer to identify the electrographic seizure onset by clinical observation alone.
Penry et al. (268) studied 374 absence seizures with video-EEG in 48 patients; a simple absence occurred in only 9% of patients; automatisms occurred in at least one episode in 88%, clonic components occurred in 41%, and seizures lasted less than 20 seconds in duration in 85%. Automatisms may be perseverative or occurring de novo, and autonomic abnormalities may consist of pupillary dilatation, pallor, flushing, salivation, or incontinence. In a recent study, absence seizures ranged from 1 to 44 seconds in duration, the average duration was 9.4 seconds, and only 65% lasted between 4 and 20 seconds and 66% had major impairment in awareness; no myoclonia, except in the face, occurred in 74%, no photic induction occurred in 83% (269).
The EEG in a typical absence seizure shows a symmetric 3 Hz generalized spike and wave discharge, which has a faster frequency at the beginning and a slower frequency at the end. The atypical absence seizure has a frequency between 1.5 to 2.5 Hz (usually slower), may be asymmetric, and usually has a slow background (see above).
EEG Manifestations
The ictal EEG of the absence seizure is characterized by the generalized synchronous 3 Hz spike-wave discharge. No typical absence seizure can occur without this classical pattern, but the 3 Hz spike-wave pattern may occur, usually in bursts of less than 5-second duration, without an accompanying clinical absence seizure. This should serve as a stern warning not to equate generalized 3 Hz spike waves with absence seizures; the latter cannot occur without the former, but the former frequently occur without clinical absences (52, 53 and 54) (Fig. 26.20).
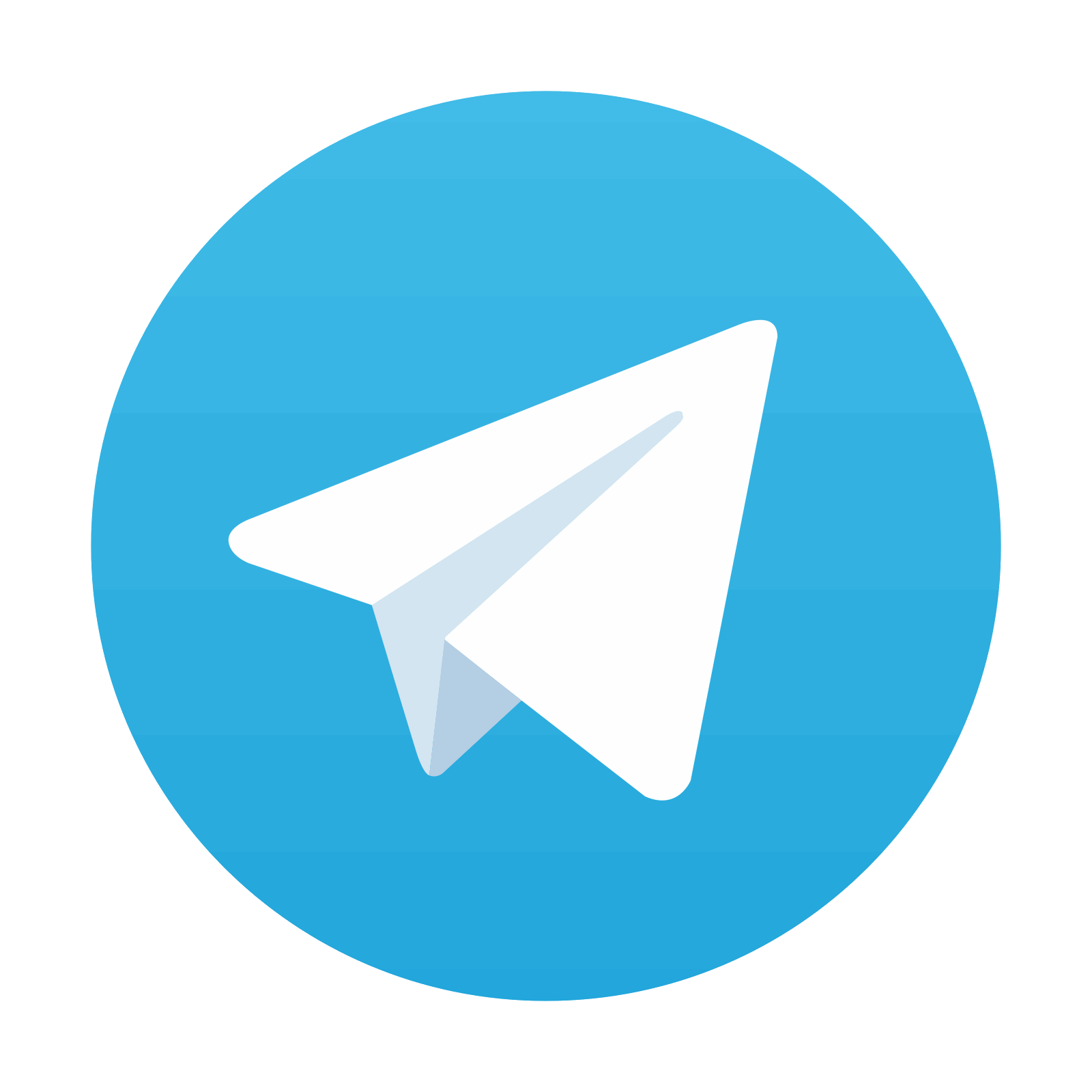
Stay updated, free articles. Join our Telegram channel

Full access? Get Clinical Tree
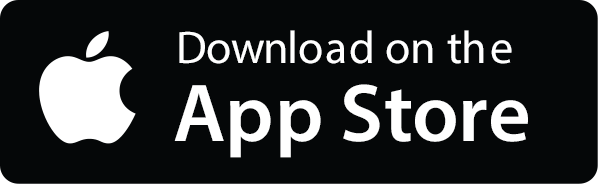
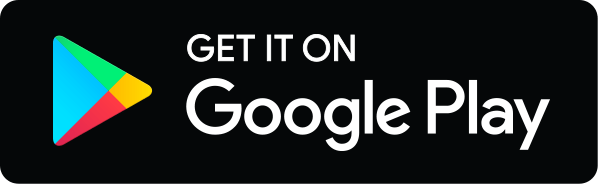
