Fig. 7.1
SpineAssist setup. Preoperative CT-based planning of the transpedicular screws (a), intraoperative oblique fluoroscopy for referencing the SpineAssist robot (b), SpineAssist positioned on the bed mount angulating the screw trajectory (c), end of procedure demonstrating tiny skin incision for four pedicular screws and lateral TLIF (d)
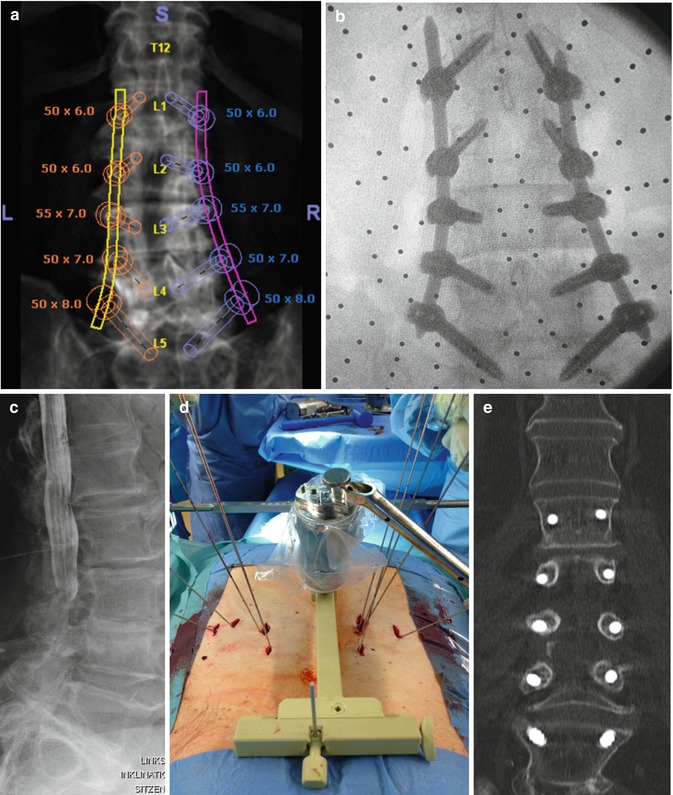
Fig. 7.2
Percutaneous dorsal five-level instrumentation with SpineAssist robot. Preoperative coronal CT-based planning (a) compared to intraoperative AP view (b); preoperative myelography demonstrating severe stenosis (c). Intraoperative setup with the robotic device positioned on the bed mount angulating the screw trajectory and all 10 K-wires in place (e). Postoperative coronal CT with 100 % accuracy (d)
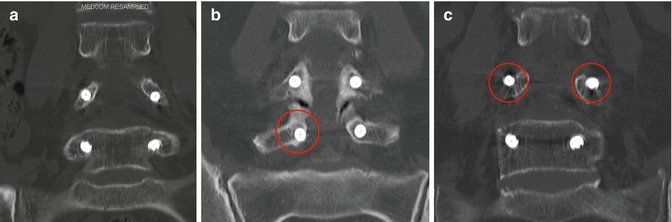
Fig. 7.3
Gertzbein -Robbins classification (coronal reformatted postoperative CT scans). Screws exactly within the pedicle were evaluated as group A (a) and screws deviating < 2 mm were evaluated as group B (b), ≥2 to < 4 mm as group C (c), ≥4 mm to <6 mm as group D (not shown), and more than 6 mm as group E (not shown)
Recent reports have considered the contribution of robotic-assisted techniques in minimizing radiation doses and exposure times during spinal interventions. A median radiation exposure 39.9 % lower than that recorded for conventional pedicle screw-insertion techniques was reported for SpineAssist-guided percutaneous spinal fusion surgery [28]. These findings resonate with those demonstrating an average radiation exposure time of 34 s/screw for robotic-guided insertions versus a mean 77 s/screw in freehand procedures using conventional techniques [22]. It is worthwhile noting that 30 of the 55 surgeries performed with robotic guidance were using an MIS approach, while the parallel group of screws were all inserted using an open approach. Similarly, Barzilay et al. [29] reported an up to 60 % reduction in radiation exposure under SpineAssist support, when compared to best reports in the literature. Moreover, when comparing spinal instrumentation executed using a freehand technique (n = 10, all using an open approach) versus robotic (n = 18, all using MIS) support, Roser et al. found that the radiation times and doses for the robotic groups were 1.97-fold and 1.71-fold shorter, respectively [25].
The increasing incidence of spinal surgeries has promoted design of supportive aides aimed at improving procedural performance and overcoming limitations of less invasive approaches. Automation and robotics have been applied to a wealth of procedures performed in spine surgery. A meta-analysis published by Kosmopoulos et al. determined that out of 16,717 pedicle screws placed in the lumbar spine in vivo, 86.7 % were accurate [30]. Moreover, the meta-analysis, covering 37,337 pedicle screw implants in total, showed that navigation of pedicle screw implantations improved placement accuracy by a mean of 5 % when compared to unassisted procedures.
Introduced in the 1990s, spinal surgical robotic systems have significantly increased the surgeon’s capacity to accurately perform minimally invasive surgical procedures in a variety of clinical applications. The reproducibility, precision, and accuracy of their movements have enabled robots to perform some surgical tasks more precisely than their human counterparts. Renaissance has recently been adapted to guide needle aspiration brain biopsies, deep brain stimulation electrode placement procedures, brain mapping, and shunt placements. These intricate and delicate procedures require maximal precision, due to minimal tolerance of the eloquent regions of the brain to surgical deviations. The dedicated software enables the user to design a preoperative plan, using an MRI or CT scan, which is then fused with the CT scan performed on the day of the procedure with a fiducial array used to fuse and synchronize the imaging modalities and the planning with the robot’s mounting platform. Renaissance application in intracranial procedures is expected to provide precision equivalent to the gold standard frame-based stereotactic surgeries while significantly reducing patient discomfort, by abrogating the need for affixation of a head-encaging stereotactic frame to the patient’s skull.
Advanced imaging capacities and platform compatibility with both MRI and CT will further enhance accuracy and precision, boosting their applicability and relevance in spinal procedures, with notable support of MIS approaches. Whenever implementing new techniques, a significant learning curve has to be taken into consideration; the registration process could lead to systemic errors before execution of the procedure. Again, safety is a big issue, as computer-controlled surgical robotics may be associated with inadvertent motion.
Even though the utilization of robotics in surgery is in its infancy, we believe that the few surgical robots performing currently in operating rooms have already shown great potential to improve surgical outcomes, especially where accuracy and minimal invasiveness are needed. A robotic device should be ideally universal in its use, not only designed specifically for the spine but capable of various procedures to create a joint venture among surgical disciplines.
References
1.
Beasley RA (2012) Medical robots: current systems and research directions. J Robotics. Article ID 401613, 14 pages; doi: 10.1153/2012/401613
2.
3.
Devito DP, Kaplan L, Dietl R, Pfeiffer M, Horne D, Silberstein B et al (2010) Clinical acceptance and accuracy assessment of spinal implants guided with SpineAssist surgical robot: retrospective study. Spine 35(24):2109–2115PubMedCrossRef
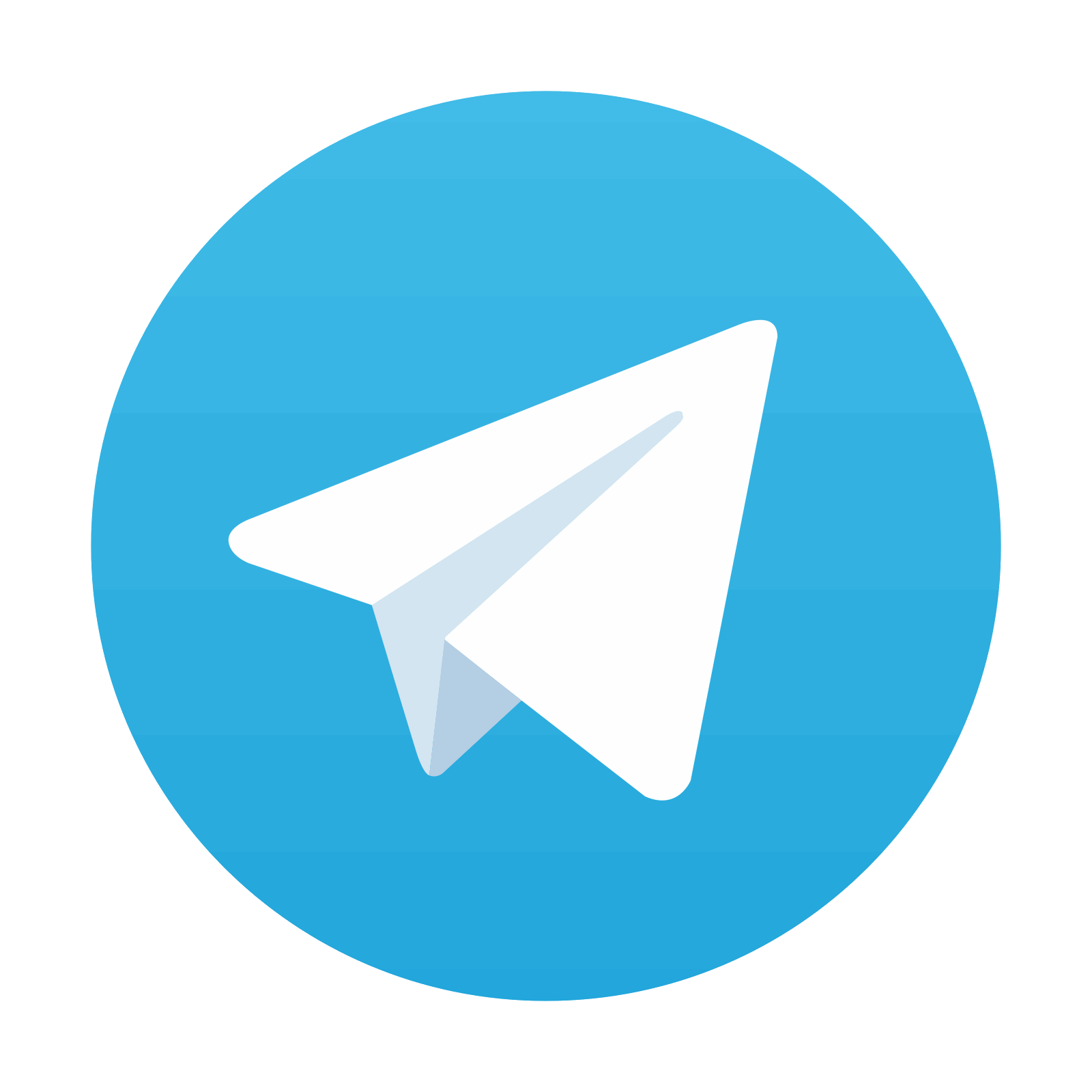
Stay updated, free articles. Join our Telegram channel

Full access? Get Clinical Tree
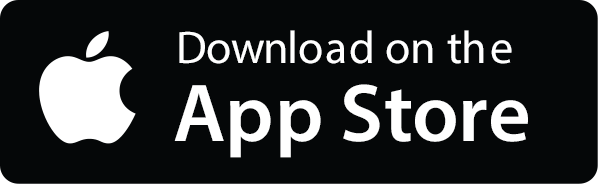
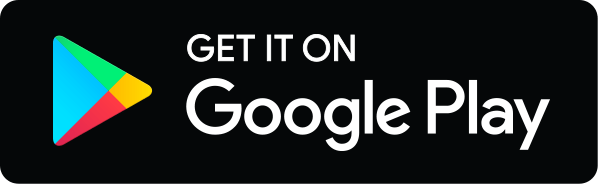