13
Stroke
Stroke is the fourth leading cause of death in the United States (after heart disease, cancer, and chronic lung disease) and the most common disabling neurologic disorder. Approximately 750,000 new strokes occur and approximately 150,000 people die from stroke in the United States each year.
The incidence of stroke increases with age, with approximately two-thirds of all strokes occurring in those older than 65 years, and is somewhat higher in men than in women and in African Americans than in whites. Modifiable risk factors for stroke include systolic or diastolic hypertension, atrial fibrillation, diabetes, dyslipidemia, and physical inactivity (Table 13-1). The incidence of stroke has decreased in recent decades, largely because of improved treatment of hypertension.
Table 13-1. Well-documented risk factors for stroke.1
Genetic factors also appear to be important in stroke pathogenesis, although the cause of most strokes is likely to be multifactorial and involve both polygenic and environmental influences. Several, mostly rare Mendelian disorders have stroke as a major manifestation; some of these, in which the affected gene has been identified, are listed in Table 13-2.
Table 13-2. Some monogenic disorders associated with stroke.
APPROACH TO DIAGNOSIS
Stroke is a syndrome characterized by four key features:
1. Sudden onset—The sudden onset of symptoms is documented by the history.
2. Focal involvement of the central nervous system—The site of central nervous system involvement is suggested by the nature of the symptoms and signs, delineated more precisely by the neurologic examination, and confirmed by imaging studies (computed tomography [CT] scans or magnetic resonance imaging [MRI]).
3. Lack of rapid resolution—The duration of neurologic deficits is documented by the history. In the past, the standard definition of stroke required that deficits persist for at least 24 hours to distinguish stroke from transient ischemic attack (discussed later). However, any such time point is arbitrary, and transient ischemic attacks usually resolve within 1 hour.
4. Vascular cause—A vascular cause may be inferred from the acute onset of symptoms and often from the patient’s age, the presence of risk factors for stroke, and the occurrence of symptoms and signs referable to the territory of a particular cerebral blood vessel. When this is confirmed by imaging studies, further investigations can be undertaken to identify a more specific etiology, such as arterial thrombosis, cardiogenic embolus, or clotting disorder.
ACUTE ONSET
Strokes begin abruptly. Neurologic deficits may be maximal at onset, as is common in embolic stroke, or may progress over seconds to hours (or occasionally days), which may occur with progressive arterial thrombosis or recurrent emboli.
A stroke that is actively progressing as a direct consequence of the underlying vascular disorder (but not because of associated cerebral edema) or has done so in recent minutes is termed stroke in evolution or progressing stroke (Figure 13-1).
Figure 13-1. Time course of cerebral ischemic events. A transient ischemic attack (TIA) produces neurologic deficits that resolve completely within a short period, usually within 1 hour. Stroke-in-evolution, or progressing stroke, causes deficits that continue to worsen even as the patient is seen. Completed stroke is defined by the presence of persistent deficits, which may be stable or improving when the patient is seen; it does not necessarily imply that the entire territory of the involved vessel is affected or that no improvement has occurred since the onset.
Focal cerebral deficits that develop slowly (over weeks to months) are unlikely to be due to stroke and are more suggestive of another process, such as tumor or inflammatory or degenerative disease.
FOCAL INVOLVEMENT
Stroke produces focal symptoms and signs that correlate with the area of the brain supplied by the affected blood vessel.
In ischemic stroke (discussed in more detail later), occlusion of a blood vessel interrupts the flow of blood to a specific region of the brain, interfering with neurologic functions dependent on that region and producing a more or less stereotyped pattern of deficits.
Hemorrhage produces a less predictable pattern of focal involvement because complications such as increased intracranial pressure, cerebral edema, compression of brain tissue and blood vessels, or dispersion of blood through the subarachnoid space or cerebral ventricles can impair brain function at sites remote from the hemorrhage.
Cerebrovascular disorders can also affect the brain in more diffuse fashion and produce global cerebral dysfunction, but the term stroke should not be applied in these cases. These disorders include global cerebral ischemia (usually from cardiac arrest) and subarachnoid hemorrhage (discussed in Chapter 6).
In most cases of stroke, the history and neurologic examination provide enough information to localize the lesion to one side of the brain (eg, to the side opposite a hemiparesis or hemisensory deficit or to the left side if aphasia is present) and to the anterior or posterior cerebral circulation.
ANTERIOR (CAROTID) CIRCULATION
The anterior cerebral circulation supplies most of the cerebral cortex and subcortical white matter, basal ganglia, and internal capsule. It consists of the internal carotid artery and its branches: the anterior choroidal, anterior cerebral and middle cerebral arteries. The middle cerebral artery in turn gives rise to deep, penetrating lenticulostriate branches (Figure 13-2). The specific territory of each of these vessels is listed in Table 13-3.
Figure 13-2. Major cerebral arteries. The anterior and posterior cerebral circulations comprise arteries that arise anterior and posterior to the posterior communicating arteries, respectively. The circle of Willis is formed by the anterior communicating artery and the anterior cerebral, internal carotid, posterior communicating, and posterior cerebral arteries. (Reproduced, with permission, from Waxman S. Clinical Neuroanatomy. 26th ed. New York, NY: McGraw-Hill; 2010.)
Table 13-3. Territories of the principal cerebral arteries.
Anterior circulation strokes are commonly associated with symptoms and signs that indicate hemispheric dysfunction (Table 13-4), such as aphasia, apraxia, or agnosia (described in Chapter 1). They often produce hemiparesis, hemisensory disturbances, and visual field defects, which can also occur with posterior circulation strokes.
Table 13-4. Symptoms and signs of anterior and posterior circulation ischemia.
POSTERIOR (VERTEBROBASILAR) CIRCULATION
The posterior cerebral circulation supplies the brainstem, cerebellum, thalamus, and portions of the occipital and temporal lobes. It consists of the paired vertebral arteries, the basilar artery, and their branches: the posterior inferior cerebellar, anterior inferior cerebellar, superior cerebellar, and posterior cerebral arteries (Figure 13-2). The posterior cerebral artery also gives off thalamoperforate and thalamogeniculate branches. Areas supplied by these arteries are listed in Table 13-3.
Posterior circulation strokes produce symptoms and signs of brainstem dysfunction (Table 13-4), including coma, drop attacks (sudden collapse without loss of consciousness), vertigo, nausea and vomiting, cranial nerve palsies, ataxia, and crossed sensorimotor deficits that affect the face on one side of the body and the limbs on the other. Hemiparesis, hemisensory disturbances, and visual field deficits also occur, but are not specific to posterior circulation strokes.
DURATION OF DEFICITS
Stroke produces neurologic deficits that persist. When symptoms and signs of cerebral ischemia resolve completely after briefer periods (usually within 1 hour), the term transient ischemic attack (TIA) is used (Figure 13-1). Recurrent TIAs with identical clinical features (stereotypic TIAs) are usually caused by thrombosis or embolism arising within the cerebral circulation. TIAs that differ in character from event to event suggest recurrent emboli from a cardiac source. Although TIAs do not themselves produce lasting neurologic dysfunction, they are important to recognize because approximately one-third of patients with TIAs will go on to have a stroke within 5 years—and because this risk may be reduced with treatment.
VASCULAR ORIGIN
Although hypoglycemia, other metabolic disturbances, trauma, and seizures can produce focal central neurologic deficits that begin abruptly and last for at least 24 hours, the term stroke is used only when such events are caused by vascular disease.
The underlying pathologic process in stroke can be either ischemia or hemorrhage, usually arising from an arterial lesion. Ischemia accounts for approximately 90% and hemorrhage for approximately 10% of strokes in typical American and European series. It may not be possible to distinguish between ischemia and hemorrhage from the history and neurologic examination, but CT scan or MRI permits a definitive diagnosis.
ISCHEMIA
Interruption of blood flow to the brain deprives neurons, glia, and vascular cells of substrate glucose and oxygen. Unless blood flow is promptly restored, this leads to ischemic death of brain tissue (infarction) within the ischemic core, where flow is typically less than 20% of normal. The pattern of cell death depends on the severity of ischemia. With mild ischemia, as may occur in cardiac arrest with reperfusion, selective vulnerability of certain neuronal populations result in their preferential loss. More severe ischemia produces selective neuronal necrosis, in which all neurons die but glia and vascular cells are preserved. Complete, permanent ischemia, such as occurs in stroke, causes pannecrosis, affecting all cell types. If the patient survives, this ultimately produces chronic cavitary brain lesions.
Where ischemia is incomplete (20%-40% of normal)—as in the border zone or penumbra surrounding the core of an ischemic brain region—cell damage is potentially reversible and cell survival may be prolonged. However, unless blood flow is restored, by recanalization of the occluded vessel or collateral circulation from other vessels, reversibly damaged cells begin to die as well, and the infarct expands. Death of penumbral tissue is associated with a worse clinical outcome.
Two pathogenetic mechanisms can produce ischemic stroke—thrombosis and embolism. However, the distinction is often difficult or impossible to make on clinical grounds.
Thrombosis
Thrombosis produces stroke by occluding large cerebral arteries (especially the internal carotid, middle cerebral, or basilar), small penetrating arteries (as in lacunar stroke), cerebral veins, or venous sinuses. Symptoms typically evolve over minutes to hours. Thrombotic strokes are often preceded by TIAs, which tend to produce similar symptoms because they affect the same territory recurrently.
Embolism
Embolism produces stroke when cerebral arteries are occluded by the distal passage of thrombus from the heart, aortic arch, or large cerebral arteries. Emboli in the anterior cerebral circulation most often occlude the middle cerebral artery or its branches, because approximately 85% of the hemispheric blood flow is carried by this vessel. Emboli in the posterior cerebral circulation usually lodge at the apex of the basilar artery or in the posterior cerebral arteries. Embolic strokes characteristically produce neurologic deficits that are maximal at onset. When TIAs precede embolic strokes, especially those arising from a cardiac source, symptoms typically vary between attacks because different vascular territories are affected.
HEMORRHAGE
Hemorrhage may interfere with cerebral function through a variety of mechanisms, including destruction or compression of brain tissue and compression of vascular structures, leading to secondary ischemia and edema. Intracranial hemorrhage is classified by its location as intracerebral, subarachnoid, subdural, or epidural, all of which—except subdural hemorrhage—are usually caused by arterial bleeding.
Intracerebral Hemorrhage
Intracerebral hemorrhage causes symptoms by compressing adjacent tissue (which can then produce local ischemia) and, to a lesser extent, by destroying tissue. Unlike ischemic stroke, intracerebral hemorrhage tends to cause more severe headache and depression of consciousness as well as neurologic deficits that do not correspond to the distribution of any single blood vessel.
Subarachnoid Hemorrhage
Subarachnoid hemorrhage leads to cerebral dysfunction by elevating intracranial pressure as well as by exerting still poorly understood toxic effects of subarachnoid blood on brain tissue. In addition, subarachnoid hemorrhage may be complicated by vasospasm (leading to ischemia), rebleeding, extension of blood into brain tissue (producing an intracerebral hematoma), or hydrocephalus. Subarachnoid hemorrhage typically presents with headache rather than focal neurologic deficits; it is discussed in Chapter 6.
Subdural or Epidural Hemorrhage
Subdural or epidural hemorrhage produces a mass lesion that can compress the underlying brain. These hemorrhages are often traumatic in origin and usually present with headache or altered consciousness. Because their recognition is most critical in the setting of coma, subdural and epidural hemorrhages are discussed in Chapter 3.
FOCAL CEREBRAL ISCHEMIA
PATHOPHYSIOLOGY
The pathophysiology of focal cerebral ischemia is complex, as it involves a process that evolves over time, affects the brain nonuniformly, and targets multiple cell types. Nevertheless, several potentially important underlying mechanisms have been identified. These are not mutually exclusive and are in many cases mutually reinforcing. Some mechanisms are likely to have their major impact early and others later in the course of stroke. Moreover, some contribute to ischemic injury, whereas others promote tissue survival or repair.
INJURY MECHANISMS
Energy Failure
Neurons rely on oxidative metabolism to generate the large amounts of adenosine triphosphate (ATP) required to support their high energy demands. Reduction of blood flow interferes with the delivery of two key substrates for this process—oxygen and glucose—causing ATP levels to fall. Cells can compensate to a limited extent by generating ATP via an alternative, oxygen-independent pathway, namely glycolysis (conversion of glucose to pyruvate and lactate). However, without prompt reperfusion, this strategy is inadequate; cells cease to function normally and eventually die. Like other injury mechanisms, energy failure is most pronounced in the ischemic core and less so in the surrounding penumbra.
Ion Gradients
One of the major uses of cell-generated energy is the maintenance of transmembrane ion gradients. With energy failure, these gradients are dissipated. Na+/K+-ATPase, which accounts for approximately two-thirds of neuronal energy expenditure and is responsible for maintaining high intra-cellular K+ concentrations, fails to do so. As a consequence, K+ leaks from cells and depolarizes adjacent cells, activating voltage-gated ion channels, Ca2+ influx, and neurotransmitter release. High extracellular levels of K+, together with glutamate (see later), are also thought to cause cortical spreading depression, an electrical phenomenon associated with neuron and astrocyte depolarization, which consumes energy and may extend the infarct.
Calcium Dysregulation
Ca2+ is maintained at low cytoplasmic levels by a variety of sequestration and extrusion mechanisms. Ischemia-induced elevation of extracellular K+ causes membrane depolarization and triggers Ca2+ entry through voltage-gated Ca2+ channels, voltage-sensitive glutamate receptor-gated channels, and transient receptor potential channels. Ca2+ also enters through acid-sensitive ion channels activated by H+ produced by glycolysis. The rise in intracellular Na+ that results from loss of Na+/K+-ATPase activity causes the Na+/Ca2+ exchanger, which normally exports Ca2+ from cells against its concentration gradient, to operate in reverse and import Ca2+. In addition, Ca2+ induces further Ca2+ release from endoplasmic reticulum.
As intracellular Ca2+ levels rise, the Ca2+-buffering capacity of organelles (mitochondria and endoplasmic reticulum) and Ca2+-binding proteins is overwhelmed. Catabolic proteases, lipases, and nucleases are activated, mitochondrial function is compromised, and cell death pathways are mobilized.
Excitotoxicity
The term excitotoxicity refers to the pathogenic effects of excitatory neurotransmitters, especially glutamate, on central neurons. Ischemia promotes excitotoxicity by stimulating depolarization-induced synaptic release of neuronal glutamate, releasing glutamate through reversal of astrocytic glutamate uptake, and activating voltage-sensitive glutamate receptor-gated channels. The receptors primarily involved in excitotoxicity are N-methyl-D-aspartate (NMDA)-preferring, ionotropic (ion channel-coupled) receptors, especially those that are extrasynaptic and contain an NR2B subunit. In contrast, synaptic NMDA receptors are thought to be involved in normal glutamate-mediated neurotransmission.
Influx of Ca2+ through NMDA receptor-coupled channels contributes to the Ca2+ dysregulation described previously. In addition, this route of Ca2+ entry is closely linked to the activation of neuronal nitric oxide synthase (NOS1), which generates the gaseous transmitter (gasotransmitter), nitric oxide (NO). Neuronally derived NO contributes to nitrosative injury, as described later. In contrast, NO generated by endothelial nitric oxide synthase (NOS3) confers protection in ischemia, at least partly through its vasodilator properties.
Oxidative and Nitrosative Injury
Some of the toxic effects of ischemia are mediated through the production of highly reactive oxygen-containing species (ROS) and nitrogen-containing species (RNS). These include superoxide anion (O2–) and NO, which can combine to generate peroxynitrite (ONOO–). Because these compounds contain oxygen, they are thought to act primarily during the reperfusion phase that follows ischemia. Their effects are protean, but include inhibiting mitochondrial enzymes and function, damaging DNA, activating ion channels, causing covalent modification of proteins, and triggering cell death pathways.
Cell Death Cascades
Ischemic cell death probably proceeds by several mechanisms, depending partly on location and time course. Death is thought to occur most rapidly in the infarct core and more slowly in the penumbra and during reperfusion. Rapid cell death in the ischemic core is epitomized by necrosis, in which cells and organelles swell, membranes rupture, and cellular contents spill into the extracellular space. However, slower and more regulated modes of cell death (programmed cell death), which require energy and protein synthesis, may predominate in the penumbra and during reperfusion injury.
The best characterized form of programmed cell death is apoptosis, which is associated with cell shrinkage, membrane blebbing without rupture, and DNA fragmentation. Apoptosis may be triggered by extracellular (extrinsic pathway) or intracellular (intrinsic pathway) signals; the latter predominate in stroke. Cell death via the intrinsic pathway can be subdivided further based on its dependence on cysteine-aspartic proteases (caspases). Both caspase-dependent and caspase-independent cell death prominently involve mitochondria. In both cases, Ca2+ overload and movement of proapoptotic proteins from cytoplasm to mitochondria alter the permeability of the outer mitochondrial membrane, permitting the release of additional proapoptotic factors. In caspase-dependent cell death, the major factor involved is cytochrome c, which translocates from mitochondria to the cytoplasm and activates caspase-9 and then the executioner caspase, caspase-3. In caspase-independent cell death, apoptosis-inducing factor (AIF) translocates from mitochondria to the nucleus, where it promotes DNA degradation.
Inflammation
Cerebral ischemia triggers an inflammatory response that involves both resident and blood-borne cells of the innate immune system. The former include astrocytes and microglia, and the latter neutrophils, lymphocytes, and monocytes. Inflammation begins within blood vessels as a response to occlusion and is amplified by cell death within brain parenchyma. Adaptive immune responses may emerge later in the course. Molecular mediators of ischemia-induced inflammation include adhesion molecules, cytokines, chemokines, and proteases. Although the early inflammatory response to ischemia is thought to provoke secondary injury, subsequent inflammatory events may be neuroprotective or contribute to tissue repair.
SURVIVAL & REPAIR MECHANISMS
Collateral Circulation
The first line of defense against ischemia is collateral circulation which, if adequate, can bypass an arterial occlusion. The cerebral circulation contains numerous collateral pathways, and the observation that patients with total occlusion of a major vessel may sometimes be asymptomatic indicates that these pathways can be clinically functional. However, the fact that stroke occurs at all indicates that this is not always the case. Examples of collateral routes for cerebral blood flow include the following:
1. Bilateral vertebral artery occlusion—anterior spinal artery
2. Common carotid artery occlusion—contralateral common carotid artery via ipsilateral external carotid artery or vertebral artery via ipsilateral occipital artery
3. Internal carotid artery occlusion—ipsilateral external carotid artery via ophthalmic artery or circle of Willis
4. Middle cerebral artery occlusion—ipsilateral anterior or posterior cerebral artery via leptomeningeal anastomoses
Inhibitory Neurotransmitters
As noted previously, ischemic brain tissue is characterized by excessive excitation, caused by a combination of gluta-mate, extracellular K+, and other factors. This appears to be mitigated early in the course of stroke by enhanced tonic inhibition mediated through extrasynaptic GABAA receptors. Later, however, this inhibitory effect may be detrimental to recovery.
Transcriptional Hypoxia Response
The cellular response to hypoxia is mediated partly by hypoxia-inducible protein-1 (HIF-1) and its transcriptional activation of proteins that promote cell survival and tissue recovery. These include glycolytic enzymes, erythropoietin (EPO), and vascular endothelial growth factor (VEGF). In addition to their respective effects on erythropoiesis and angiogenesis (discussed later), EPO and VEGF also exert direct protective effects on ischemic cells. Other cytoprotective proteins induced after ischemia include antiapoptotic proteins, growth factors, and heat-shock proteins.
Neurogenesis
New neurons and astrocytes continue to be produced in the adult brain in at least two regions: the hippocampal dentate gyrus and the subventricular zone surrounding the lateral ventricles. Cerebral ischemia stimulates neurogenesis, and some of the cells generated migrate from the subventricular zone to ischemic striatum and cerebral cortex. The extent to which they can replace the large numbers of cells lost after stroke is likely limited, but these cells may promote the survival and repair of ischemic brain tissue by releasing growth factors, suppressing inflammation, or other effects.
Angiogenesis
Ischemia also stimulates the sprouting of existing blood vessels to enhance local blood supply, which is mediated largely through the hypoxia response discussed previously and specifically by VEGF. Because this process takes at least several days, it probably has little impact in the acute phase of stroke, but may help to protect against subsequent ischemic episodes.
Ischemic Tolerance
Another mechanism through which ischemia may protect against a subsequent bout of ischemia is ischemic tolerance. In this process, mild ischemia preconditions brain tissue so it is better able to survive more severe ischemia for a few days thereafter. Ischemic tolerance appears to involve extensive changes in gene expression and numerous molecular mediators.
Repair Mechanisms
Most patients recover to some extent after stroke, reflecting a capacity for spontaneous postischemic repair and the brain’s innate plasticity. Plastic changes occur in the peri-infarct region and at remote sites, such as the contralateral cerebral hemisphere, and include changes in gene expression, increased neuronal excitability, axonal sprouting, synaptogenesis, somatotopic reorganization, and the formation of new neuronal circuits. Not all of these changes are necessarily beneficial, however. For example, increased activation of the contralesional hemisphere may in some cases simply reflect more severe injury.
PATHOLOGY
LARGE VESSEL TERRITORY INFARCTION
On gross inspection, a recent infarct is a swollen, softened area of brain that usually affects both gray and white matter. Microscopy shows acute ischemic changes in neurons (shrinkage, microvacuolization, dark staining), destruction of glial cells, necrosis of small blood vessels, disruption of nerve axons and myelin, and accumulation of interstitial fluid from vasogenic edema. In some cases, perivascular hemorrhages are observed in the infarcted area.
LACUNAR INFARCTION
In contrast to infarcts associated with major cerebral blood vessels, smaller lacunar infarcts result from lipohyalinosis of small resistance vessels, usually in patients with chronic hypertension. Lacunar infarcts—often multiple—are found in approximately 10% of brains at autopsy. The pathologic appearance is of small cavities ranging in size from 0.5 to 15 mm in diameter.
CLINICAL-ANATOMIC CORRELATION
Infarction in the distribution of different cerebral arteries often produces distinctive clinical syndromes. Recognizing these syndromes can facilitate anatomic and sometimes etiologic diagnosis and thereby help guide treatment.
ANTERIOR CEREBRAL ARTERY
Anatomy
The anterior cerebral artery supplies the parasagittal cerebral cortex (Figures 13-3 and 13-4), which includes portions of motor and sensory cortex related to the contralateral leg and the so-called bladder inhibitory or micturition center.
Figure 13-3. Arterial supply of the primary motor and sensory cortex (lateral view). The middle cerebral artery supplies parts of the primary motor and sensory cortex related to face and arm function, whereas the anterior cerebral artery supplies parts of the primary motor and sensory cortex related to leg function. This explains why middle cerebral artery strokes affect the face and arm most severely, whereas anterior cerebral artery strokes affect the leg. (Reproduced, with permission, from Waxman S. Clinical Neuroanatomy. 26th ed. New York, NY: McGraw-Hill; 2010.)
Figure 13-4. Arterial supply of the primary motor and sensory cortex (coronal view). (Reproduced, with permission, from Waxman S. Clinical Neuroanatomy. 26th ed. New York, NY: McGraw-Hill; 2010.)
Clinical Syndrome
Anterior cerebral artery strokes are uncommon, perhaps because emboli from the extracranial vessels or the heart are more apt to enter the larger-caliber middle cerebral artery, which receives the bulk of cerebral blood flow. There is a contralateral paralysis and sensory loss affecting the leg. Voluntary control of micturition may be impaired because of failure to inhibit reflex bladder contractions, resulting in precipitate micturition.
MIDDLE CEREBRAL ARTERY
Anatomy
The middle cerebral artery supplies most of the remainder of the cerebral hemisphere and deep subcortical structures (Figures 13-3 and 13-4). The cortical branches of the middle cerebral artery include the superior division, which supplies the entire motor and sensory cortical representation of the face, hand, and arm, and the expressive language (Broca) area of the dominant hemisphere (Figure 13-5). The inferior division supplies the visual radiations, the region of visual cortex related to macular vision, and the receptive language (Wernicke) area of the dominant hemisphere. Lenticulostriate branches of the most proximal portion (stem) of the middle cerebral artery supply the basal ganglia as well as motor fibers related to the face, hand, arm, and leg as they descend in the genu and the posterior limb of the internal capsule.
Figure 13-5. Anatomic basis of middle cerebral artery syndromes. Stroke in the distribution of the middle cerebral artery causes hemiparesis affecting especially the face and arm (due to involvement of the primary motor area), hemisensory deficit affecting especially the face and arm (due to involvement of the primary sensory area), gaze preference toward the affected hemisphere (due to involvement of the frontal eye field), aphasia (due to involvement of Broca area, Wernicke area, or both, if in the dominant hemisphere), and hemianopia (due to involvement of the optic radiations leading to the primary visual area. (Reproduced, with permission, from Waxman S. Clinical Neuroanatomy. 26th ed. New York, NY: McGraw-Hill; 2010.)
Clinical Syndrome
The middle cerebral artery is the vessel most commonly involved in large vessel ischemic stroke. Depending on the site of involvement, several clinical syndromes can occur.
1. Superior division stroke results in contralateral hemiparesis that affects the face, hand, and arm but spares the leg, contralateral hemisensory deficit in the same distribution, but no homonymous hemianopia. If the dominant hemisphere is involved, these features are combined with Broca (expressive) aphasia, which is characterized by impairment of language expression with intact comprehension.
2. Inferior division stroke is less common in isolation and results in contralateral homonymous hemianopia that may be denser inferiorly, impaired cortical sensory functions (eg, graphesthesia and stereognosis on the contralateral side of the body), and disorders of spatial thought (eg, lack of awareness that a deficit exists [anosognosia], neglect of and failure to recognize the contralateral limbs, neglect of the contralateral side of external space, dressing apraxia, and constructional apraxia). If the dominant hemisphere is involved, Wernicke (receptive) aphasia occurs and is manifested by impaired comprehension and fluent but often nonsensical speech. With involvement of the nondominant hemisphere, an acute confusional state may occur.
3. Occlusion at the bifurcation or trifurcation of the middle cerebral artery involves a lesion situated at the point where the artery splits into two (superior and inferior) or three (superior, middle, and inferior) major divisions. This severe stroke syndrome combines the features of superior and inferior division stroke. Its clinical features include contralateral hemiparesis and hemisensory deficit involving the face and arm far more than the leg, homonymous hemianopia, and—if the dominant hemisphere is affected—global (combined expressive and receptive) aphasia.
INTERNAL CAROTID ARTERY
Anatomy
The internal carotid artery arises where the common carotid artery divides into internal and external carotid branches in the neck. In addition to its anterior cerebral and middle cerebral branches discussed previously, the internal carotid artery also gives rise to the ophthalmic artery, which supplies the retina. The severity of internal carotid artery strokes is highly variable, depending on the adequacy of collateral circulation, which tends to develop in compensation for a slowly evolving occlusion.
Clinical Syndrome
Intra- or extracranial internal carotid artery occlusion is responsible for approximately one-fifth of ischemic strokes. In approximately 15% of cases, progressive atherosclerotic occlusion of the internal carotid artery is preceded by premonitory TIAs or by transient monocular blindness caused by ipsilateral retinal artery ischemia.
Carotid artery occlusion may be asymptomatic. Symptomatic occlusion results in a syndrome similar to that of middle cerebral artery stroke (contralateral hemiplegia, hemisensory deficit, and homonymous hemianopia, together with aphasia if the dominant hemisphere is involved).
POSTERIOR CEREBRAL ARTERY
Anatomy
The paired posterior cerebral arteries arise from the tip of the basilar artery (Figure 13-6) and supply the occipital cerebral cortex, medial temporal lobes, thalamus, and rostral midbrain. Emboli carried up the basilar artery tend to lodge at its apex, where they can occlude one or both posterior cerebral arteries. These emboli can subsequently fragment and produce signs of asymmetric or patchy posterior cerebral artery infarction.
Figure 13-6. Sites of thrombotic and embolic occlusions in the vertebrobasilar circulation. A: Thrombotic occlusion of the basilar artery. B: Thrombotic occlusion of both vertebral arteries. C: Embolic occlusion at the apex of the basilar artery. D: Embolic occlusion of both posterior cerebral arteries.
Clinical Syndrome
Occlusion of a posterior cerebral artery produces homonymous hemianopia affecting the contralateral visual field. Macular vision may be spared, however, because of the dual (middle and posterior cerebral artery) blood supply to the portion of the visual cortex representing the macula (Chapter 7). In contrast to visual field defects from infarction in the middle cerebral artery territory, those caused by posterior cerebral artery occlusion may be denser superiorly. With occlusions near the origin of the posterior cerebral artery at the level of the midbrain, ocular abnormalities can include vertical gaze palsy, oculomotor (III) nerve palsy, internuclear ophthalmoplegia, and vertical skew deviation of the eyes. When posterior cerebral artery occlusion affects the occipital lobe of the dominant (usually left) hemisphere, patients may exhibit anomic aphasia (difficulty in naming objects), alexia without agraphia (inability to read, with no impairment of writing), or visual agnosia. The last is a failure to identify objects presented in the left side of the visual field, caused by a lesion of the corpus callosum that disconnects the right visual cortex from language areas of the left hemisphere. Bilateral posterior cerebral artery infarction may result in cortical blindness, memory impairment (from temporal lobe involvement), or the inability to recognize familiar faces (prosopagnosia), as well as a variety of exotic visual and behavioral syndromes.
BASILAR ARTERY
Anatomy
The basilar artery usually arises from the junction of the paired vertebral arteries (Figure 13-6), although in some cases only a single vertebral artery is present. The basilar artery courses over the ventral surface of the brainstem to terminate at the level of the midbrain, where it bifurcates to form the posterior cerebral arteries (see earlier discussion). Branches of the basilar artery supply the occipital and medial temporal lobes, the medial thalamus, the posterior limb of the internal capsule, and the entire brainstem and cerebellum.
Clinical Syndromes
1. Thrombosis—Thrombotic occlusion of the basilar artery (Figure 13-6)—a serious event that is often incompatible with survival—causes bilateral neurologic signs referable to involvement of multiple branch arteries (Figure 13-7). Occlusion of both vertebral arteries (Figure 13-6) or of a lone unpaired vertebral artery produces a similar syndrome. Temporary occlusion of one or both vertebral arteries can also occur in relation to rotating the head in patients with cervical spondylosis, leading to transient symptoms and signs of brainstem dysfunction.
Figure 13-7. Arterial supply of the brainstem. A: Midbrain. The basilar artery gives off paramedian branches that supply the oculomotor (III) nerve nucleus and the red nucleus (RN). A larger branch, the posterior cerebral artery, courses laterally around the midbrain, giving off a basal branch that supplies the cerebral peduncle (CP) and a dorsolateral branch supplying the spinothalamic tract (ST) and medial lemniscus (ML). The posterior cerebral artery continues (upper arrows) to supply the thalamus, occipital lobe, and medial temporal lobe. B: Pons. Paramedian branches of the basilar artery supply the abducens (VI) nucleus and the medial lemniscus (ML). The anterior inferior cerebellar artery gives off a basal branch to the descending motor pathways in the basis pontis (BP) and a dorsolateral branch to the trigeminal (V) nucleus, the vestibular (VIII) nucleus, and the spinothalamic tract (ST), before passing to the cerebellum (upper arrows). C: Medulla. Paramedian branches of the vertebral arteries supply descending motor pathways in the pyramid (P), the medial lemniscus (ML), and the hypoglossal (XII) nucleus. Another vertebral branch, the posterior inferior cerebellar artery, gives off a basal branch to the olivary nuclei (ON) and a dorsolateral branch that supplies the trigeminal (V) nucleus, the vestibular (VIII) nucleus, and the spinothalamic tract (ST), on its way to the cerebellum (upper arrows). (Reproduced, with permission, from Waxman S. Clinical Neuroanatomy. 26th ed. New York, NY: McGraw-Hill; 2010.)
Major stenosis or occlusion of the subclavian artery before it has given rise to the vertebral artery can lead to the subclavian steal syndrome, in which blood passes from the vertebral artery into the distal subclavian artery with physical activity of the ipsilateral arm. The syndrome is a manifestation of generalized atherosclerosis and is not predictive of stroke in the vertebrobasilar system. Patients are usually asymptomatic, and stroke, when it occurs, is typically due to coexisting carotid lesions.
Basilar thrombosis usually affects the proximal portion of the basilar artery (Figure 13-6), which supplies the pons. Involvement of the dorsal portion (tegmentum) of the pons produces unilateral or bilateral abducens (VI) nerve palsy; horizontal eye movements are impaired, but vertical nystagmus and ocular bobbing may be present. The pupils are constricted as a result of the involvement of descending sympathetic pupillodilator fibers in the pons, but they may remain reactive. Hemiplegia or quadriplegia is usually present, and coma is common. Although the syndrome of basilar occlusion in unconscious patients may be confused with pontine hemorrhage, a CT or MRI brain scan will differentiate the two.
In some patients with basilar occlusion, the ventral portion of the pons (basis pontis) is infarcted and the tegmentum is spared. Such patients remain conscious but quadriplegic. The term locked-in syndrome is applied to this state. Locked-in patients may be able to signify that they are conscious by opening their eyes or moving their eyes vertically on command. In other cases, a conventional electroencephalogram (EEG) with stimulation may be needed to distinguish the locked-in state (in which the EEG is normal) from coma (Chapter 3).
2. Embolism—Emboli small enough to pass through the vertebral arteries into the larger basilar artery are usually arrested at the top of the basilar artery, where it bifurcates into the posterior cerebral arteries (Figure 13-6). The resulting reduction in blood flow to the ascending reticular formation of the midbrain and thalamus produces immediate loss or impairment of consciousness. Unilateral or bilateral oculomotor (III) nerve palsies are characteristic. Hemiplegia or quadriplegia with decerebrate or decorticate posturing occurs because of the involvement of the cerebral peduncles in the midbrain. Thus the top of the basilar syndrome may be confused with midbrain damage caused by transtentorial uncal herniation. Less commonly, an embolus may lodge more proximally in an atheromatous narrowed portion of the basilar artery, producing a syndrome indistinguishable from basilar thrombosis.
Smaller emboli may occlude the rostral basilar artery transiently before fragmenting and passing into one or both posterior cerebral arteries (Figure 13-6). In such cases, portions of the midbrain, thalamus, and temporal and occipital lobes can be infarcted. If conscious, these patients display a variety of visual (homonymous hemianopia, cortical blindness), visuomotor (impaired convergence, paralysis of upward or downward gaze, diplopia), and behavioral (especially confusion) abnormalities without prominent motor dysfunction. Sluggish pupillary responses are a helpful sign of midbrain involvement.
LONG CIRCUMFERENTIAL VERTEBROBASILAR BRANCHES
Anatomy
The long circumferential branches arising from the vertebral and basilar arteries are the posterior inferior cerebellar, anterior inferior cerebellar, and superior cerebellar arteries (Figure 13-2). These vessels supply the dorsolateral brainstem, including dorsolaterally situated cranial nerve nuclei (V, VII, and VIII) and pathways entering and leaving the cerebellum in the cerebellar peduncles.
Clinical Syndromes
Occlusion of one of the circumferential branches produces infarction in the dorsolateral medulla or pons.
1. Posterior inferior cerebellar artery occlusion results in the lateral medullary (Wallenberg) syndrome (Chapter 8). This syndrome varies in its presentation with the extent of infarction, but it can include ipsilateral cerebellar ataxia, Horner syndrome, and facial sensory deficit; contralateral impaired pain and temperature sensation; and nystagmus, vertigo, nausea, vomiting, dysphagia, dysarthria, and hiccup. The motor system is characteristically spared because of its ventral location in the brainstem.
2. Anterior inferior cerebellar artery occlusion leads to infarction of the lateral portion of the caudal pons and produces a syndrome with many of the same features. Horner syndrome, dysphagia, dysarthria, and hiccup do not occur, however, but ipsilateral facial weakness, gaze palsy, deafness, and tinnitus are common findings.
3. The syndrome of lateral rostral pontine infarction from superior cerebellar artery occlusion resembles that associated with anterior inferior cerebellar artery lesions, but impaired optokinetic nystagmus or skew deviation of the eyes may occur. Auditory function is unaffected, and the contralateral sensory disturbance may involve touch, vibration, and position sense as well as pain and temperature sense.
LONG PENETRATING PARAMEDIAN VERTEBROBASILAR BRANCHES
Anatomy
Long penetrating paramedian arteries supply the medial brainstem from its ventral surface to the floor of the fourth ventricle. Structures located in this region include the medial portion of the cerebral peduncle, sensory pathways, the red nucleus, the reticular formation, and the midline cranial nerve nuclei (III, IV, VI, XII).
Clinical Syndrome
Occlusion of a long penetrating artery causes paramedian infarction of the brainstem and results in contralateral hemiparesis if the cerebral peduncle is affected. Associated cranial nerve involvement depends on the level of the brainstem at which occlusion occurs. Occlusion in the mid-brain
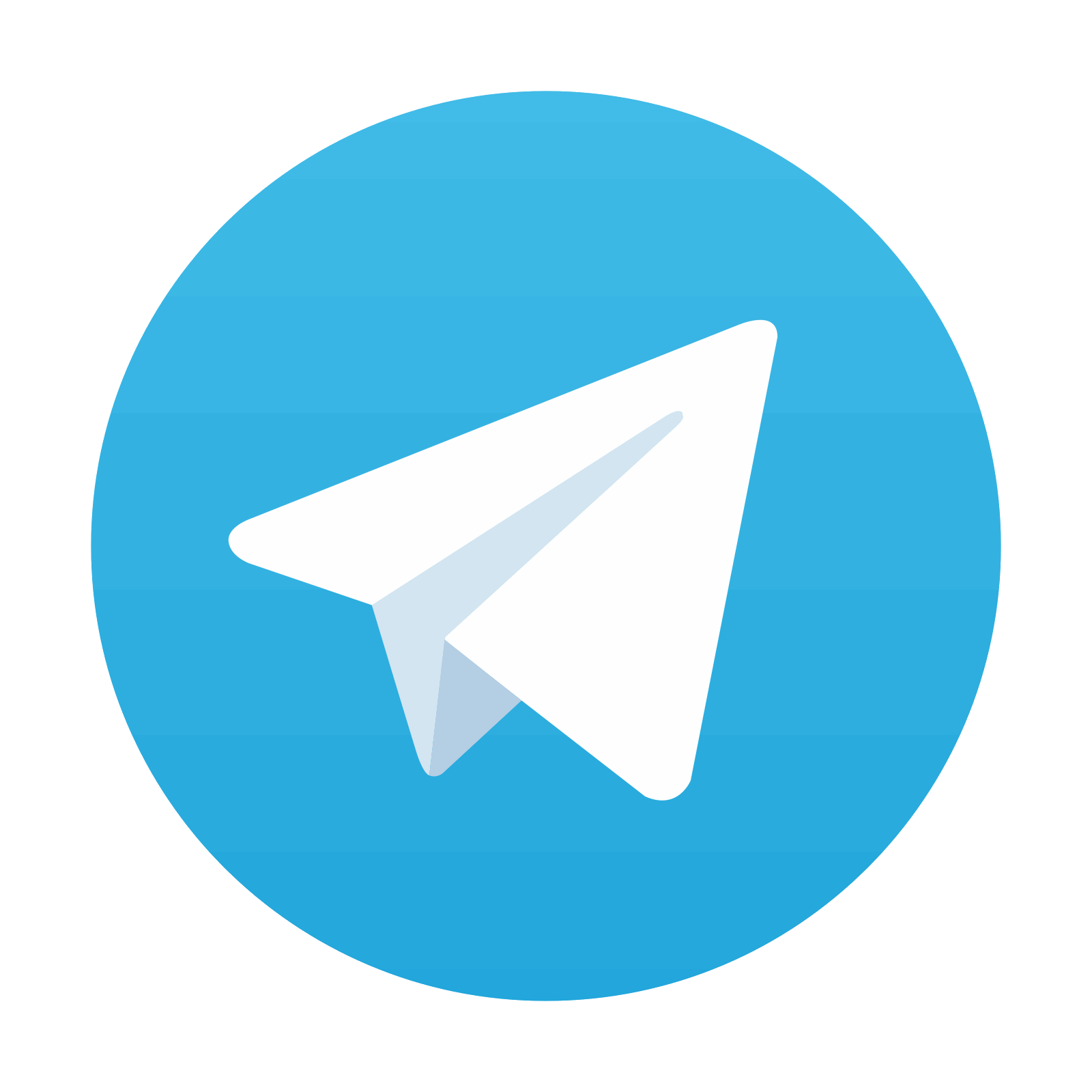
Stay updated, free articles. Join our Telegram channel

Full access? Get Clinical Tree
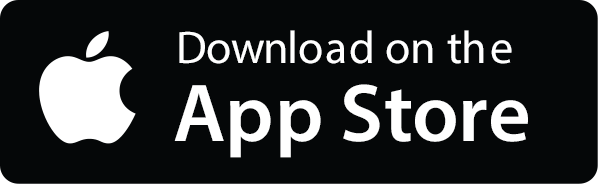
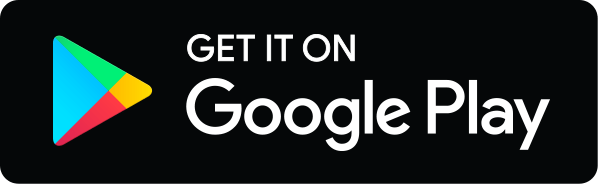