16 Summary and Synopsis of the Brain Trauma Foundation Head Injury Guidelines Vin Mathur and Jack Jallo Trauma, and in particular neurotrauma, takes a large toll on society. Of the 1 million Americans evaluated in emergency rooms for traumatic brain injury (TBI) annually, nearly one quarter require inpatient care. Of the 150,000 deaths due to trauma each year, one third are secondary to head injuries. Another 10,000 people each year suffer from spinal cord injuries, with 200,000 Americans suffering from disabilities as a direct result. Although difficult to calculate, the annual net cost of trauma to society is estimated at just under $40 billion. To this end, guidelines have been developed to assist neurosurgeons, trauma surgeons, emergency room physicians, hospitals, and various other health care workers and entities involved in TBI care. The following guidelines are adapted from the recommendations developed jointly by the Brain Trauma Foundation and the American Association of Neurological Surgeons for the management of severe traumatic head injury. This review of the guidelines is divided into three sections: general adult trauma management, surgical management of traumatic lesions, and general pediatric trauma management. Utilizing an exhaustive review of the literature for various topics, recommendations in the form of standards, guidelines, and options were developed. Briefly, standards reflect the most certainty and are more likely to be based on class I data, whereas options have the least certainty and are most likely to have class II and III data. Class I data consist of prospective randomized studies. Class II is made up of studies in which either data were collected prospectively or reliable data were analyzed retrospectively. Examples include cohort and case control studies. Class III data are based on studies utilizing a retrospective analysis. With limited prospective data available, the guidelines leave much discretion to the clinician and individual patient circumstance. This chapter summarizes the pediatric and adult guidelines for the management of severe TBI as well as the surgical guidelines for the management of traumatic lesions. Table 16-1 summarizes the guidelines for adult trauma management. The guidelines recommend that all regions have an organized trauma care system. Although the exact form that this system should take is not defined, several important considerations are provided for neurosurgeons: Multiple studies noted significant drops in mortality (50% and 20%, respectively) when organized trauma systems were put into place.1,2 To date, no published data have provided evidence of better outcome with an unorganized trauma system as compared with an organized one. A neurotrauma care facility requires an Advanced Trauma Life Support (ATLS)-certified physician, most commonly a trauma surgeon, to provide the overall management of the patient, particularly for those with multiorgan injuries. The critical intervention required for all trauma patients, including those with head injuries, is adequate cardiopulmonary resuscitation. Interventions, such as prophylactic mannitol and hyperventilation, can adversely affect systemic resuscitation and outcome. A variety of class II evidence has shown increased morbidity and mortality with hypotension (systolic blood pressure less than 90 mm Hg) and hypoxia (cyanosis, apnea, or a PaO2 <60 mm Hg).3,4 Their use should therefore be limited to discrete indications (i.e., clinical evidence of elevated intracranial pressures).
General Adult Trauma Management Guidelines
Trauma Systems
Initial Management
Treatment Modality | Guidelines |
Trauma systems | Standards—Insufficient data Guidelines—Presence of organized trauma system in all geographical areas recommended Options—Neurosurgeon involvement in planning neurotrauma management at all points of care; neurosurgery, in-house trauma surgeon, ICU, and CT scanner at trauma facilities; trauma surgeon trained to perform evacuations of extra-axial hematomas in rural facilities with no neurosurgeon |
Initial systems | Standards—Insufficient data Guidelines—Insufficient data Options—Systemic resuscitation; treatment of elevated intracranial hypertension when appropriate |
Resuscitation of blood pressure and oxygenation | Standards—Insufficient data Guidelines—Avoid hypotension (SBp < 90 mm Hg) and hypoxia (oxygen saturation < 90% or PaO2 < 60 mm Hg) Options—Mean arterial pressure above 90 mm Hg; the airway should be secured, utilizing entro tracheal intubation if possible, in patients with declining neurological status (i.e., a GCS score < 9) or hypoxemia |
Indications for intracranial pressure monitoring | Standards—Insufficient data Guidelines—ICP monitoring for TBI patients with a GCS score of 3 to 8 and presence of an intracranial contusion, hematoma, edema, or effacement of basal cisterns; in those with normal CT scans, ICP may be monitored if two or more of the following are present: motor posturing on exam, age > 40 years, SBp < 90 Options-None |
Intracranial pressure treatment threshold | Standards—Insufficient data Guidelines—Treatment should begin when ICPs reach 20–25 mm Hg Options—Management of ICPs requires evaluation of cerebral perfusion pressures and clinical examinations |
Recommendations for intracranial pressure monitoring technology | The ventricular catheter is considered the most cost-efficient and accurate means for monitoring ICP |
Recommendations for cerebral perfusion pressure | Standards—Insufficient data Guidelines—Insufficient data Options—Cerebral perfusion pressures should be maintained above 60 mm Hg |
Hyperventilation | Standards—In a patient with normal intracranial pressures, prolonged hyperventilation (PaCO2 < 25 mm Hg) is not recommended Guidelines—In the first 24 hours after TBI, the use of prophylactic hyperventilation (PaCO2 < 35 mm Hg), secondary to its possible deleterious effects on CPP, is not recommended Options—Hyperventilation may be utilized when intracranial hypertension is refractory to other modalities of treatment; monitoring for cerebral ischemia may be necessary with PaCO2 below 30 mm Hg |
The use of mannitol/ hyperosmolar therapy | Standards—Insufficient data Guidelines—Intracranial hypertension may be effectively managed with mannitol, utilizing doses of 0.25–1.0 g/kg of body weight Options—Mannitol may be used in the presence of neurological deterioration without ICP monitoring; serum osmolarity should remain below 320 mOsm, and the patient should be kept euvolemic; boluses, as compared with a continuous infusion, may be more effective in managing intracranial hypertension |
The use of barbiturates in the control of intracranial hypertension | Standards—Insufficient data Guidelines—Reserved for patients with refractory intracranial hypertension Options—None |
The role of steroids | Standards—No role in the management of patients with TBI Guidelines—None Options—None |
Nutrition | Standards—Insufficient data Guidelines—For paralyzed patients, 100% of resting metabolism use should be replaced, whereas 140% should be replaced in nonparalyzed patients; 15% of calories should be in the form of protein (nitrogen replacement) Options—To avoid gastric complications, feeds via gastrojejunostomy are recommended |
The role of anti-seizure prophylaxis following head injury | Standards—Anticonvulsants are not recommended for preventing late posttraumatic seizures Guidelines—None Options—Phenytoin and carbamazepine may be used to help prevent early posttraumatic seizures |
CPP, cerebral perfusion pressure; CT, computed tomography; GCS, Glasgow Coma Scale; ICP, intracranial pressure; ICU, intensive care unit; SBp, systolic blood pressure; TBI, traumatic brain injury.
Sedation
There are no published studies evaluating the effect of sedation on outcome. The use of sedation for patients with head injury is left to the discretion of the physician.
Neuromuscular Blockade
Only one study (by Hsiang et al) evaluated the outcome for head injury patients with prophylactic neuromuscular blockade use.5 The study found that patients receiving paralytics had a higher incidence of pneumonia and sepsis as well as a longer stay in the ICU. Therefore the use of neuromuscular blockade should be limited to patients with intracranial hypertension and for assisting with transportation.
Hyperventilation
Hyperventilation is a commonly used method for reducing elevated intracranial pressures (ICPs). This is accomplished with vasoconstriction, which results in reduced cerebral blood flow (CBF) and ultimately reduced ICPs. Unfortunately, for patients with head injuries, studies have shown that CBF is less than one half that of an uninjured individual’s 1 day after the injury. This poses potential complications for the clinician considering hyperventilation for the management of elevated ICPs. One study clearly showed no benefit with the use of prophylactic hyperventilation.6
Hyperventilation can reduce ICPs, but when significantly utilized may result in loss of autoregulation and cerebral ischemia, again stressing the importance of using this modality for discrete indications only.
When there is clinical evidence of intracranial hypertension, aggressive treatment is recommended. Hyperventilation may be instituted relatively rapidly, and unlike mannitol, independently of the patient’s volume status.
Resuscitation of Blood Pressure and Oxygenation
Although there are insufficient data to establish standards, the guidelines strongly recommend avoiding hypotension, defined as a single episode of systolic blood pressure less than 90 mm Hg, and hypoxia defined as a PaO2 less than 60 mm Hg, or the presence of cyanosis or apnea. It should be noted that the systolic blood pressure value of 90 mm Hg does not necessarily reflect an ideal physiological end point, and in fact a higher value may be ideal; unfortunately, no studies are available to evaluate an elevated parameter.
It is recommended that the clinician, utilizing intravenous (IV) fluids if necessary, maintain an appropriate blood pressure so that an adequate cerebral perfusion pressure (CPP), defined as greater than 60 mm Hg, can be achieved.
For patients who remain hypoxemic, particularly in those with a Glasgow Coma Scale (GCS) score of less than 9, endotracheal intubation is strongly recommended.
Hypoxemia and hypotension have been documented to cause worse outcomes in head-injury patients.7 Unfortunately, studies have revealed that hypoxemia affects TBI patients commonly, up to 44% in one study,8 occurring most commonly in the field or during transportation. Another study documented the devastating results of hypoxemia where 50% of patients with oxygen saturations less than 60 mm Hg died and the remainder were severely disabled as compared with a rate of 14.3% mortality and a severe disability rate of less than 5% in those who were not hypoxemic.9 The same study revealed that 55% of patients were hypoxemic prior to intubation, raising again the importance of prehospital airway management.
Early intubation (i.e., prior to arrival in the emergency room), has been found to reduce mortality, particularly in the sickest patients.10,11 In terms of determining when a patient should be intubated, one study noted a need for earlier intubation for those with a lower GCS score; for those with a GCS of 3 to 5, 27% were intubated in the field, and 72% in the emergency room whereas GCS scores of 8 to 9 had 8% of patients intubated in the field and 53% in the emergency room.12
The Traumatic Coma Data Bank (TCDB), which provided prospectively collected data (class II), revealed that the presence of either or both hypotension and hypoxemia was among the most important predictors of poor outcome.3,13 Hypotension was found to double mortality and increase morbidity when compared with normotensive patients. Hypotension most likely causes worse outcomes in TBI patients secondary to inadequate CPPs.
Although the American College of Surgeons (ACS) recommends that crystalloid be rapidly administered to trauma patients, the ATLS cautions that fluids may inadvertently exacerbate cerebral edema and elevate ICPs. Of note, one study did reveal no correlation between ICPs and the amount of fluid or blood administered.14 Various studies have begun to reveal hypertonic saline as a viable alternative for resuscitation in TBI patients. ICPs were found to be reduced in TBI patients,15 whereas a meta-analysis16 revealed a doubling of survival in those receiving hypertonic saline. Other studies cited revealed no change in outcome or improved outcome primarily for those with a GCS score of less than 9.
Indications for Intracranial Pressure Monitoring
As a guideline, ICP monitoring is recommend for patients with a GCS score of 3 to 8 and a CT scan with an acute intracranial finding such as a hematoma. Monitoring should also be considered in those with a normal head CT who have any two of the following: age greater than 40, systolic blood pressure less than 90 mm Hg, or unilateral or bilateral posturing. It is left to the discretion of the physician to consider monitoring in those patients who do not meet these criteria.
Normal ICP is considered to be 0 to 10 mm Hg, with most citing 20 mm Hg as the upper limit of normal. As already mentioned, systemic hypotension may have a deleterious effect on TBI patients because of reduced CPPs, and because CPP is defined as the difference between the mean arterial pressure and ICP, monitoring ultimately allows the clinician to evaluate and maintain adequate cerebral perfusion.
Although multiple studies have documented poor outcomes for severe head injury patients (GCS score <8) with elevated ICP, routine ICP monitor placement has not been supported. For example, less than 3% of patients with a GCS of 14 or 15 later deteriorated into a coma, limiting the efficacy of ICP monitoring.
In TBI patients with a GCS score of less than 8, intracranial hypertension was found to develop in 53% to 63% of patients with an abnormal CT as compared with 13% in those with a normal CT. The rate increased to the level of the abnormal CT patients when patients with a normal CT were found to have at least two of three aforementioned criteria for ICP monitor placement. An analysis of the TCDB revealed that elevated ICP pressures (>20 mm Hg) were the most important predictor of poor outcome followed by hypotension (systolic blood pressure <80 mm Hg).
An ICP monitor’s most important role may be for evaluating and guiding intervention for management of elevated ICPs. Indications for the use and efficacy of treatment modalities, including hyperventilation, mannitol, sedation, and paralysis, are often guided by the values provided by the ICP monitor. In addition, multiple studies have ascertained that ICP values can determine outcome, with lower ICPs often equating with better outcomes.
Multiple studies appear to show a reduction in mortality and morbidity when an ICP monitor is used, both for ICP monitoring and for cerebrospinal fluid (CSF) drainage. Some of the earlier studies17,18 showed a marked decrease in mortality (30% vs 50%) when various intensive care management interventions, including ICP monitoring, were implemented and compared with a prior study.19 Another study seemed to support more aggressive intervention; in a study completed in 1982, patients who were treated for ICPs greater than 15 mm Hg, as compared with those with ICP greater than 20 to 25 mm Hg, were found to have lower mortality (28% vs 46%) and reduced incidence of ICPs greater than 25 mm Hg (25% vs 34%).20 A nonrandomized study by Ghajar et al found a mortality rate of 12% in the group of patients who were monitored as compared with 53% for the group that was not.21 Another nonrandomized analysis, completed in the United States, also appears to show reduced mortality for patients monitored and drained.22 Mortality was found to be 21% when CSF drainage was used routinely and 35% and 43% when used occasionally and never, respectively.
Intracranial Pressure Treatment Threshold
It is recommended as a guideline that intervention for elevated ICPs should begin after a value of 20 to 25 mm Hg is reached. Because patients can herniate at a level less than 20 mm Hg and some patients may be able to tolerate ICPs greater than 25, this value must be evaluated in the context of frequent clinical examinations and CT scans.
Most of the studies, none of which are class I, agree with beginning treatment after a value of 20 mm Hg. One study, as already mentioned, appears to indicate a reduction in mortality (28% vs 46%) when treatment is initiated at a value 15 mm Hg versus 25 mm Hg.21 This study appears to be confounded by inconsistent management in the two groups.
Recommendations for Intracranial Pressure Monitoring Technology
The ventricular catheter, with its ability to transduce ICPs and drain CSF, is considered the most effective means for monitoring a patient’s ICPs. Transducing ICPs with a parenchymal monitor initially may be as accurate as a ventricular catheter but is prone to measurement drift. Others such as subarachnoid, subdural, and epidural monitors are considered much less effective at this time.
There are data that appear to show a discrepancy between values read on a subdural or parenchymal monitor and true ventricular pressures.23 Considering that most studies regarding ICP monitoring are based on ventricular pressures these monitors have the potential to inadvertently cause mismanagement of a patient’s ICPs.
Complications related to ICP monitors are uncommon and rarely cause long-term sequelae. Bacterial infection was found on average in 5% of ventricular, subarachnoid, and subdural monitors, and 14% in parenchymally placed monitors. Infections of significance were exceedingly rare. The overall incidence of hematomas secondary to monitor placement was 1.4%,24,25 with 0.5% requiring surgical intervention.24,26
Rates of malfunction or obstruction vary greatly from 6.3% for ventricular catheters to as high as 40% in some studies for parenchymal monitors.27,28
Recommendations for Cerebral Perfusion Pressure
As a therapeutic option, maintaining CPP greater than 60 mm Hg has the potential to significantly reduce the morbidity and mortality in TBI patients. CPP is the difference between the mean arterial pressure and ICP. Analogous to voltage, it provides the gradient to drive the current, or in this case the CBF. Intravascular depletion with hypotension, loss of autoregulation, and posttraumatic vasospasm, as well as elevated ICPs can significantly reduce CPP and ultimately lead to cerebral ischemia, by some estimates the most deleterious complication of TBI.
An unfortunate fact for TBI patients is that CBF appears to be significantly below normal following the injury, predisposing the patient to cerebral ischemia. This reduced CBF may be due to vasospasm, reduced metabolism, or as a direct result of a mass lesion. Unlike CPP or ICP, no consensus regarding a numerical value above which CBF should be maintained has been established.
Brain tissue oxygen pressures (tipO2) have been found to increase by 62% when CPP was increased from 32 to 67, which correlated with better neurological outcomes.29 As mentioned before, studies have documented a worse outcome for patients who have even one episode of hypotension (systolic blood pressure <90).30,31 This is most likely due to a reduced CPP. In addition, hypotension has been found to cause elevations in ICP, possibly due to cerebral vasodilation exacerbating the issue of a suboptimal CPP.
ICP appears to remain stable or, at worst, has a minimal change when blood pressure is increased by as much as 30 mm Hg, independent of whether cerebral autoregulation is intact. Loss of autoregulation, however, makes it more likely that ICP will be more labile with increases in the systolic blood pressure.
In one of the largest studies of its kind, Rosner et al found that, of the 71% of patients who survived, 80% had a positive outcome; of note in this study, CPP was maintained at an average value of 83 and ICP at 27.32 McGraw found an increase in mortality of 20% for each 10 mm Hg that the CPP was lowered; the difference is stark with a mortality of 35% to 40% for a CPP above 80 mm Hg and 95% when below 60 mm Hg.33
For studies maintaining a CPP of 70 mm Hg, the average mortality was found to be 21% and compares favorably with the mortality rate of 40% seen in the TCDB; favorable outcomes were noted in 54% as compared with 37% in TCDB. Unfortunately, artificially maintaining the CPP above 70 is associated with the development of acute respiratory distress syndrome.34 As such, the correct value that CPP should be kept above is 60 mm Hg.
Hyperventilation
Two important findings speak to the difficulty in determining when hyperventilation for TBI patients should be implemented: research indicating 40% of TBI patients develop brain swelling and consequently elevated ICPs35 and studies showing that CBF is one half of normal 1 day after injury.36 Although it may stem the devastating effects of uncontrolled ICP, by inducing cerebral vasoconstriction, hyperventilation may inadvertently exacerbate cerebral ischemia. In fact, improved outcome was noted for patients who did not receive prophylactic hyperventilation as compared with those who did, whereas autopsy results have shown most victims of TBI with cerebral ischemia.
With sufficient data available, both standards and guidelines have been developed. As a standard, it is recommended that for those without elevated ICPs, chronic prolonged hyperventilation (PaCO2 <25 mm Hg) should not be implemented. Recommended as a guideline, during the first 24 hours after injury, prophylactic hyperventilation (PaCO2 <35 mm Hg) should not be instituted. As an option, the clinician may use hyperventilation when the patient has elevated ICPs or neurological decline refractory to other modalities of treatment. If used, monitoring for cerebral ischemia, utilizing brain tissue oxygen monitoring for example, should be considered.
As mentioned, CBF drops precipitously following injury, falling as low as 20 mL/100 g/min in the immediate hours afterward. For those who survive, CBF gradually increases over several days. The parameters below which irreversible cerebral ischemia will set in have yet to be determined. A study by Heiss et al noted a mean CBF of 16.7 mL/100 g/min at the center of an infarct and 31 mL/100 g/min in the surrounding tissue.37 Studies have shown which intracranial injuries are most likely to precipitate a lower CBF as compared with those that only cause a small change or no change. Those with epidural hematomas (EDHs) and unremarkable CT scans have the smallest change from baseline CBF, whereas diffuse injuries, subdural hematomas, as well as those with hypotension have the largest.
Oxygen desaturations, defined as below 50% when measured with jugular venous saturations, occur with low CBF and are associated with poor outcome.
A study evaluating hyperventilation in uninjured patients showed a reduction in PaCO2 of 15 to 20 mm Hg resulted in a CBF reduction of 40%.38 Evidence has shown a disproportionate reduction in CBF as compared with cerebral blood volume when PaCO2 is reduced. When PaCO2 was reduced to 26 mm Hg, cerebral blood volume was found to reduce -7%, whereas CBF dropped by almost one third.39
The Use of Mannitol/Hyperosmolar Therapy
Recommendations for the use of mannitol in TBI include a guideline for its use in doses ranging from 0.25 g/kg to 1 g/kg for the treatment of elevated ICPs. Treatment options provided for mannitol’s use in the presence of neurological decline, optimally given in bolus form, while maintaining euvolemia and serum osmolarity below 320 mOsm.
Mannitol is commonly used in the treatment of TBI, with multiple studies documenting its positive influence on a variety of parameters, including ICP and CPP.
It most likely acts by both an immediate and a delayed mechanism. Within several minutes of being given as a bolus, mannitol can reduce ICP. This occurs because the intravascular volume is increased, diluting the hematocrit and subsequently decreasing blood viscosity. The result is an increase in CPP and CBF.
After ∼20 minutes, during which time gradients between plasma and cells have developed, the osmotic effect of mannitol begins. Mannitol’s effect may last up to 6 hours. Its risks include precipitating acute renal failure and inadvertently increasing ICP. Risk factors for renal failure include a serum osmolarity above 320 mOsm and the presence of renal disease.
By making the blood-brain barrier more porous, mannitol, particularly when given in large doses or over a long period of time, may accumulate in the brain causing an osmotic shift intracranially and resulting in an increase in brain swelling. The recommendation for administering mannitol in bolus form stems from this concern.
Its ability to increase intravascular volume has some advocating its use for resuscitation, primarily in patients with shock and TBI. Studies to date have not found a deleterious effect on cardiac function. Although there are limited data, the neuroprotective effect provided by mannitol in cases of mild ischemia is most likely due to its effect on ICP and CPP.
The Use of Barbiturates in the Control of Intracranial Hypertension
As a guideline, barbiturates are recommended for patients for whom all other interventions for managing ICP have failed. The outcomes for intractable elevations of ICP are grim. For the 10% to 15% of TBI patients who are diagnosed with this, mortality is estimated at 84% to 100%. By lowering cerebral metabolic demands, barbiturates are able to reduce CBF. Barbiturates also act by reducing free radical-dependent lipid peroxidation and varying vascular tone.
Since 1979, several studies have documented increases in survival because barbiturate-induced coma was able to lower otherwise intractable elevations in ICP. In one study, 19 of the 25 patients treated with barbiturates had ICPs reduced, with 50% having a good recovery, whereas 83% of those who did not respond died.40 A separate study reported a 33% mortality in those when ICP was controlled and 75% when it was not.41
No improvement in outcome was found when barbiturates were compared with mannitol as initial therapy for managing ICPs.42 The same study found that barbiturate-treated patients had a worse outcome when diffuse injury was present (77% mortality vs 41%).
A randomized controlled trial found no difference in outcome between one group treated prophylactically and the other without and noted that the barbiturate group was nearly eight times more likely to develop hypotension.43
Eisenberg et al’s randomized trial found that the barbiturate-treated group had at least twice the likelihood of their ICP being lowered. Ninety-two percent of those who responded were alive at 1 month compared with 17% for those who did not. Ninety percent of those whose ICP did not respond were dead or vegetative at 6 months compared with 36% for those who did. Hypotension was found to be the most common systemic complication.44
Although pentobarbital is most commonly used for inducing coma, multiple agents are considered appropriate. A study comparing pentobarbital and etomidate noted a similar effect on ICP and a statistically insignificant difference regarding changes to the cardiovascular system.45 Monitoring an EEG for burst suppression is considered the most reliable means of ensuring significant reductions in cerebral metabolism and CBF.
The Role of Steroids
Significant data are present to support as a standard, the recommendation against the use of steroids as a modality of treatment for TBI patients. Steroids were first indicated in the treatment of brain tumor patients. Their ability to attenuate cerebral edema was supported by evidence that steroids reduced CSF production and vessel permeability; in addition, steroids were found to limit free radicals. Several studies correlated clinical improvement with the administration of steroids in brain tumor patients.46,47
Soon after their use became established in tumor patients, steroids were applied to a variety of neurosurgical patients, including TBI patients. Early studies appeared to support the efficacy of steroids in TBI patients, including one in which improved outcome was found in patients receiving high-dose steroids, compared with those receiving low doses.48 Following these initial favorable results, studies were published that did not support a beneficial outcome, as measured by ICPs and outcome.49,50
Evidence has mounted revealing no improvement in clinical outcome or ICPs as well as significant risks of gastrointestinal bleeding and hyperglycemia. A study by Saul et al revealed, overall, no improvement in outcome for those receiving steroids, but is of note because it showed, for those patients who improved within 72 hours of injury, the steroid group had a better outcome compared with the control group.51
Another study also showed no improvement in outcome, independent of whether the patient received high- or low-dose steroid.52 This same study, however, did reveal an improvement in outcome in those patients who were under 40 and received high-dose steroids.
Two recent prospective studies, utilizing a synthetic steroid, also found no improvement in outcome for patients receiving steroids. These were large studies taking place on three different continents, one in North America52 with nearly 1200 patients and the other53 with over 1100 patients in Europe and Australia. The latter study did find improvement with the synthetic drug in male patients who presented with a subarachnoid hemorrhage (SAH).
The CRASH trial completed in 2004 actually found a higher mortality in head injury patients treated with methylprednisolone as compared with the group treated with placebo.54
A meta-analysis completed in 1997, including many of the aforementioned prospective trials as well as several others, came to the expected conclusion of no improvement in outcome with steroid use in TBI patients.55
The Role of Antiseizure Prophylaxis following Head Injury
Posttraumatic seizures (PTSs) have been found to occur in up to 42% of patients up to 36 months after incurring a head injury. The rates vary for early PTSs, defined as occurring within 7 days of injury (4% to 25%), and late seizures, occurring after 7 days (9% to 42%).
Standards and options have been developed that provide for distinctive intervention depending on whether early or late seizures are being treated. As a treatment standard, anticonvulsants are not recommended for the prevention of late seizures. As an option available to clinicians, anticonvulsants, primarily phenytoin and carbamazepine, may be used for early seizures. With this said, evidence has yet to support improved outcomes with anticonvulsants.
Seizures in and of themselves can be dangerous, particularly in a compromised brain, and may precipitate elevations in ICPs, hemodynamic instability, and reductions in cerebral oxygenation. Studies support the use of anticonvulsants to prevent early PTSs to reduce the chances of epilepsy.56
Considering, as with any medication, the potential side effects of anticonvulsants, ranging from benign rashes to dysequilibrium, the use of medications should be limited to the patients most at risk for developing PTSs. Studies have found certain intracranial lesions, such as intracerebral as well as extra-axial hematomas, depressed skull fractures, and low GCS score (<10) predispose patients to developing seizures.56,57
Phenytoin was one of the first medications found to be beneficial in reducing the development of PTSs. Its use became quite common in the 1970s when nearly two thirds of neurosurgeons prescribed phenytoin prophylactically. Later studies utilizing phenytoin, phenobarbital, or a combination of the two failed to provide evidence of reduced PTSs.56,57
In the largest prospective trial 404 patients were randomized.58 The results, once again, showed that phenytoin reduced early seizures but was of no benefit in reducing late seizures. Of importance, however, no difference in survival between the two treatment groups was noted. In this trial, the majority of patients were ensured therapeutic levels of the medication. In addition, the placebo and phenytoin groups showed similar rates of developing side effects.
A recent prospective study compared the efficacy of phenytoin and valproate in preventing seizures.59 The medications appeared to be equally effective in reducing early seizures. Valproate was associated with a higher mortality rate.
Nutrition
The guidelines recommend that full nutritional replacement should begin by the seventh day after injury. Using either enteral or parenteral means, 140% of patients’ metabolic needs should be replaced if they are not paralyzed, and 100% if they are. Metabolic needs have been found to increase an average of 60% in head-injury patients without paralytics, and 20% to 30% in those with, indicating that increased muscle tone accounts for much of the increase in caloric needs. The caloric needs for a 25-year-old male, at 70 kg, is considered 1700 kcal for 24 hours, which would make needs after injury ∼2400 kcal. Without nutrition, head-injured patients have been found to lose ∼ 15% of body weight per week, primarily in the form of nitrogen (protein). Mortality has been found to increase once there has been 30% or greater weight loss. Recommendations include that 15% of the calories replaced should be provided as protein.
As an option for the clinician, to avoid gastric complications, jejunal feeds utilizing a gastrojejunostomy may be implemented.
Another study found an increase in mortality for patients with undernourishment for 2 weeks after injury.60 In line with achieving full nutrition by the seventh day after injury, studies have supported beginning feeds by the third day after injury. After injury, nitrogen excretion increases and the consumption of nitrogen increases from 10% in those uninjured to 30% in those with head injuries.61 For this reason, it is important not only to achieve appropriate total caloric replacement but also to provide the appropriate amount of nitrogen replacement. Nitrogen excretion returns to normal levels approximately 2 weeks after injury.
Certain studies have found that jejunal and parenteral feeds replace lost nitrogen more effectively than gastric feeds. Gastric feeds have also been found to predispose to dysfunction of the esophageal sphincter and gastric emptying.
Enteral feeds are preferred over the parenteral route for several reasons, which include a reduced likelihood of hyperglycemia and infection, as well as being more cost-effective. Many studies have supported the exacerbation of hypoxic ischemic brain with hyperglycemia. Studies have supported worse outcome when hyperglycemia is present. Another study revealed that more insulin is required for maintaining a normal blood glucose level with parenteral feedings as compared with enteral.62 Although there are no data supporting an increased rate of infection with parenteral feedings in TBI patients, studies with trauma to other organ systems support a reduced rate of infection with enteral feeds. Although animal studies have found parenteral nutrition may exacerbate cerebral edema, these findings, fortunately, have not been replicated clinically.63,64 Despite the increased day-to-day cost of parenteral nutrition, comparing the entire cost of a hospitalization shows more comparable costs this is most likely due to the cost of placing and maintaining functioning feeding tubes. Despite concerns with parenteral feeds, their most important advantage is that they are well tolerated by patients.
Surgical Management of Traumatic Lesions
Table 16-2 summarizes the guidelines for surgical management of traumatic lesions
Surgical Management of Acute Epidural Hematomas
Surgical evacuation of an acute EDH is dependent on the patient’s neurological status and size of the hematoma. As a treatment option, it is recommended that an EDH greater than 30 mL in volume be evacuated regardless of neurological status.
For smaller hematomas, neurological status dictates surgical and medical management. For a hematoma less than 30 mL in volume, 15 mL in thickness, with less than 5 mm of midline shift in a patient with no focal deficits and a GCS score greater than 8, nonoperative management with neurological exams and CT scans is appropriate. Regardless of size, in a patient with anisocoria and a GCS score less than 9, the hematoma should be evacuated as soon as possible.
EDHs are found in up to 4% of TBI patients65,66 and 9% of patients who present in a coma.67,68 The vast majority occur in patients aged 20 to 30 and is much rarer in those older and younger. Over 90% of all epidurals occur secondary to traffic accidents, falls, or assaults, with accidents accounting for over half (53%). Falls are the leading cause (49%) followed by traffic accidents (34%) in the pediatric population where the mean age is 6 to 10 years. Classically, the source of bleeding is considered to be the middle meningeal artery, but in a recent study this was identified as the source in only 36% of adults and 18% of children.69 Other sources include the venous sinuses and diploic veins. EDH occurs most commonly in the temporal and temporoparietal regions.
Patients with an EDH may present with a spectrum of findings, ranging from no deficits to comatose. A little under half (47%) present with the classic “lucid interval” whereas up to 27% present neurologically intact.70,71 Up to 42%70,71 of patients remain awake until the time of surgical evacuation, whereas up to 44% present with pupillary findings.
Multiple factors, including the patient’s age and GCS score, affect the outcome for patients with an EDH. Mortality ranges from 7.0% to 12.5% in adults and is ∼ 5% in the pediatric population.
Multiple studies support the GCS score at the time of presentation as the single most important factor in determining a patient’s outcome. Studies have shown the pronounced effect of GCS scores on mortality, including one by Gennarelli et al where EDH with a presenting GCS of 6 to 8 had a mortality of 9% compared with 36% when the GCS score was 3 to 5.67
Poor outcome can occur regardless of pupillary findings as Van den Brink et al showed.72 In their study, less than favorable outcome was noted in half of patients with both pupils fixed, 35% of those with a single pupil fixed, and 30% with no pupillary findings.
Other intracranial injuries, including subdural hematomas, contusions, and cerebral swelling, have been documented in many patients with an EDH. As expected, these studies have shown an increase in morbidity and mortality when a second intracranial lesion is present. Although the data are inconsistent regarding their impact on outcome, skull fractures have been reported in up to 95% of patients with EDHs. The mechanism of injury associated with EDHs predisposes patients to extracranial injuries and has been noted in several studies.
Absolute recommendations based on CT findings are difficult to make; however, some generalizations about prognosis can be made. Poor outcome was associated with a hematoma volume >50 mL, the presence of acute blood, effacement of the basal cisterns, and a midline shift greater than 10 mm in Lee et al’s series,73 whereas Van den Brink et al72 found that only the presence of traumatic SAH predisposed a patient to a less than favorable outcome.
As mentioned, the decision to take a patient to the operating room is dependent on clinical and radiographic findings. Narrowing down the particular radiographic findings that most likely predispose a patient to operative intervention may be difficult, but possible. One such prospective study by Servadei et al compared operative and nonoperative management of EDH and found that midline shift (on average, >5 mm) and hematoma thickness (on average, >15 mm) were the most likely findings in patients requiring surgery, with over 90% of the operative cases meeting the aforementioned criterion.74 In addition, it was noted that all patients had favorable outcome.
Studies regarding delayed surgery evaluate the criterion predisposing patients for conversion from nonoperative management to operative. The prospective study by Bezircioglu et al determined that patients initially treated nonoperatively (those with a GCS score >8 among other criteria) were most likely to need surgery if the hematoma was located along the temporal lobe.75 Interestingly, a separate study by Bullock et al documented good outcome for the 10% of patients with EDH who were treated nonoperatively.76 None of these patients had temporal hematomas.
Surgical Lesion | Guidelines |
Acute epidural hematoma | Standards—Insufficient data Guidelines—Insufficient data Options—A hematoma >30 cc in size should be evacuated; conservative management may be considered for patients with hematomas <30 cc in volume, <15 mm in thickness, <5 mm in midline shift, with no focal deficit and a GCS score >8; for those requiring surgery (GCS score <9 with a dilated pupil) surgery should be completed in a timely manner |
Acute subdural hematoma | Standards—Insufficient data Guidelines—Insufficient data Options—A hematoma >10 mm in thickness or midline shift >5 mm should be evacuated; surgery should also be considered in patients with hematomas <10 mm in thickness with <5 mm in midline shift if the GCS score declines by 2, the ICP is >20 mm Hg, or there are asymmetric or dilated pupils; ICP monitoring should be provided for all patients with a GCS score <9; surgery should be completed in a timely fashion to obtain the best outcome; for patients with a GCS score <9 undergoing surgery, the preferred surgery is a craniotomy, with bone flap removal and duraplasty left at the discretion of the physician |
Traumatic parenchymal lesions | Standards—Insufficient data Guidelines—Insufficient data Options—Patients with mass effect, declining neurological status, or refractory intracranial hypertension should have the lesion surgically removed; surgery should also be performed for any lesion >50 cc in size or any frontal or temporal contusion >20 cc in size, effacement of the cisterns, and/or a midline shift >5 mm with a GCS score of 6–8; conservative management may be considered for neurologically stable patients with no mass effect or evidence of elevated ICPs; focal lesions may be treated with a craniotomy; bifrontal decompressive craniectomy, ideally completed within 48 hours of injury, should be considered for patients who develop refractory cerebral edema and intracranial hypertension; decompressive procedures are the treatment of choice for diffuse injuries and transtentorial herniation |
Posterior fossa mass lesion | Standards—Insufficient data Guidelines—Insufficient data Options—Lesions should be evacuated in the presence of mass effect or neurological decline; conservative management is reserved for neurologically stable patients with minimal to no mass effect; suboccipital craniectomy completed in a timely fashion is recommended |
Depressed skull fractures | Standards—Insufficient data Guidelines—Insufficient data Options—Open fractures displaced greater than the thickness of the skull should undergo surgery; conservative management of open fractures may be considered if the skull is depressed <1 cm, there is not a large intracranial hematoma, dural violation, involvement of the frontal sinus, wound infection, cosmetic deformity, or evidence of pneumocephalus; conservative management may be considered for closed fractures; to avoid infection, early surgery is recommended; elevation and debridement is the preferred option for operative intervention; primary bone fragment replacement may be considered; an antibiotic regimen should started for all open fractures |
GCS, Glasgow Coma Scale; ICP, intracranial pressure.
For those patients who do require operative management, expedited surgery is imperative. Cohen et al found good outcome when the EDH was evacuated within 70 minutes of the onset of pupillary dilation; all the patients taken to the operating room after 70 minutes died.77
Surgical Management of Acute Subdural Hematomas
Acute subdural hematomas (SDH) occur in up to 29% of TBI patients, with the majority male and aged 31 to 47. The majority of SDH are due to motor vehicle accidents (primary cause in those aged 18 to 40) and falls (primary cause for those older than 65). Motor vehicle accident accounts for the mechanism of injury in up to 75% of SDH patients in a coma, which may also be a reflection of concomitant diffuse axonal injury. Up to 50% of acute SDH patients may present with pupillary abnormalities, whereas up to 80% may present with a GCS score of less than 8.
The mortality from SDH requiring surgery, regardless of GCS score at presentation, is between 40% and 60%, whereas the mortality for patients requiring surgery who present in a coma is 57% to 68%.
For those patients who do require surgery 60% or more have associated intracranial and extracranial lesions. Associated lesions are seen in up to 82% of patients presenting with a GCS score <10 and up to 57% of patients regardless of GCS score. Up to one quarter may also have SAH and up to 14% may have EDH, and 18% to 51% patients have extracranial injuries, including abdominal trauma and facial fractures.
As with other TBIs, radiographic and clinical considerations, among others, must be taken into account when considering surgical intervention. Many studies have attempted to determine surgical guidelines based on CT findings. Zumkeller et al found a mortality of 90% in patients with SDH greater than 30 mm in thickness compared with only 10% in those less than 10 mm.78 A significant increase in mortality was noted for midline shift greater than 20 mm. All patients in this study underwent surgery and present with GCS scores of 3 to 15. Other studies had similar findings regarding the volume of the hematoma and midline shift. Initial nonoperative management of patients with midline shifts greater than 5 mm was found to be ineffective in a study by Wong.79 Mathew et al found that of the patients who were initially treated nonoperatively, the patients with hematomas >10 mm in thickness all eventually required surgery.80 Servadei et al found good outcome in two thirds of comatose patients treated nonoperatively if midline shift was <5 mm, thickness <10 mm, and ICP remained under 20 mm Hg.81
Several studies have documented a worsening prognosis with increasing age. One study noted a marked decline in outcome after the of age 60, whereas Wilberger et al found similar declines after the age of 65.82 Kotwica and Brzezin-ski documented a stark difference based on age in outcomes for patients requiring surgery for an SDH who initially presented with a GCS score <10.83 Patients aged 18 to 30 had a 25% mortality at 3 months compared with 75% for patients older than 50. As might be expected, increasing age with lower GCS scores correlated with worsening outcomes as well. Hatashita et al documented a 75% mortality rate in patients older than 65 who initially presented with a GCS score of 4 to 6 and underwent surgery; the rate was 34% in patients aged 19 to 40.84 A separate study documented a poor outcome in nearly 100% of patients older than 65 who underwent surgery for the evacuation of an SDH.
Most studies support an improved outcome for patients who undergo earlier versus later surgery. A large study by Haselsberger et al noted a mortality of 80% in comatose patients who underwent surgery 2 hours or more after the onset of clinical decline compared with a mortality rate of 47% for those operated on within 2 hours.71 Only 4% of the former group had a good outcome compared with 32% who had surgery within 2 hours. Likewise, Seelig et al documented 90% mortality for those undergoing surgery 4 hours or more after an injury compared with 30% for those operated on within 4 hours.85 In a study evaluating outcomes for epidural and intracerebral hematomas, as well as SDH, Sakas et al found a mortality of 63% at 6 months for those operated on 3 hours or more after the onset of herniation compared with 30% for patients operated on earlier.86
Few studies evaluated the impact of differing surgical techniques such as burr hole trephination and craniotomy on outcome. For patients with GCS scores of 4 to 6, burr hole trephination was associated with a much higher rate of mortality as compared with those undergoing craniotomy in a study by Hatashita et al.84
As an option, it is recommended that acute SDH with a midline shift >5 mm or thickness >10 mm should be operated on, ideally with a craniotomy, as soon as possible. At the very least, patients with a GCS score <9 should undergo ICP monitoring. In patients with a GCS score <9, midline shift <5, and thickness <10, the SDH should be evacuated for a decline in the GCS score of 2 or more, the presence of pupillary findings consistent with elevated ICPs, and/or ICPs >20.
Surgical Management of Posterior Fossa Mass Lesions
Although traumatic injuries of the posterior fossa (PF) make up a limited number of all head injuries (<3%), the small volume of the PF predisposes the patient to the rapid development of hydrocephalus, mass effect, and ultimately neurological decline. Therefore, as an option, it is recommended that a patient undergo surgery, preferably a suboccipital craniectomy, for neurological decline or the development of mass effect or hydrocephalus in an expedited fashion.
This does not mean, however, that patients may not be treated conservatively. Studies have supported conservative management in nonfocal patients without hydrocephalus or mass effect. There are quantifiable parameters that appear to limit the use of conservative management. One retrospective study noted that PF hemorrhages with midline shifts >5 mm, 10 cc in volume, and 15 mm in thickness had a significantly higher mortality compared with those treated with surgery.87 Interestingly, the same study showed similar outcomes for conservative and surgical management in patients whose PF hemorrhage’s dimensions were less than the aforementioned parameters.
As would be expected, additional injuries, including a second hemorrhage and supratentorial extension, are associated with worse outcome, as were hydrocephalus and acute presentation.
Surgical Management of Depressed Skull Fractures
The presence of depressed skull fractures, found in -6% of TBI cases, exposes the patient to multiple complications. Open skull fractures account for the vast majority of these (up to 90%). The danger of open skull fractures is not only infection (found in up to 10.6% of patients), but also the development of late-onset epilepsy (in up to 15%), and increased neurological morbidity (11% of patients) and mortality (19%).
Skull fractures in general have been found to be associated with increased morbidity and worse outcome. Several studies have found a significantly increased risk of developing an intracranial lesion when a skull fracture is present. Macpherson et al found that patients with skull fractures had a 25% higher risk of developing an intracranial lesion as compared with those without one.88
Conventional treatment of open skull fractures entails elevation and debridement, primarily to avoid infection. Simple fractures are commonly treated if displaced a distance greater than the skull thickness to avoid late-onset epilepsy and morbidity as well as to ensure adequate cosmesis. Several studies support this approach. Jennett and Miller found over a 50% reduction in the infection rate for patients undergoing surgical management of depressed skull fractures as compared with those who did not and over a tripling of the infection rate (to 36.5%) for those whose surgery was delayed greater than 48 hours.89
Other studies, however, appear to go against conventional wisdom. Heary et al treated 26 open skull fracture patients nonoperatively where no cases were found to develop an infection.90 The criterion for nonoperative management included no evidence of wound infection, extension into the frontal sinus, pneumocephalus, dural violation, large hematoma, large deformity, or depression greater than 1 cm.
The practice of delayed cranioplasty was also challenged. A study by Wylen et al found no infectious complications in patients who had a depressed skull fracture treated with primary replacement of bone fragments within 3 days of presentation.91 Several other studies found similar results. In addition, patients benefit by avoiding a second surgery.
Ultimately, the guidelines provide the clinician the option of nonoperative management of open depressed skull fractures if the aforementioned criteria cited in the Heary study are met. Closed depressed skull fractures may be treated similarly. Expedited surgery utilizing elevation and debridement is recommended with the concomitant use of antibiotics. The clinician may also avoid delayed cranioplasty with primary bone replacement.
Surgical Management of Traumatic Parenchymal Lesions
Traumatic parenchymal lesions, broadly defined, occur in a little over one third of all TBI patients and represent approximately one fifth of head injury patients requiring surgical intervention. The presence of a parenchymal lesion certainly predisposes a patient to surgical intervention and increased morbidity and mortality. However, quantifying the parameters, both clinical and radiographic, for when a patient should undergo surgical intervention has remained difficult. Most analyses of patient outcomes have come to the conclusion that a parenchymal lesion cannot be exclusively evaluated when determining whether a patient should proceed to the operating room. Radiographic findings, clinical status and course, associated injuries, in addition to the evaluation of the parenchymal lesion, should be taken into account when deciding between surgical and medical management.
Parenchymal lesions include hematomas, contusions, and infarcts as well as nonfocal entities such as intracerebral edema, swelling, and diffuse injury. Regardless of type, parenchymal injuries can result in mass effect, midline shift, elevated ICPs, and herniation. Despite the diverse forms a parenchymal lesion may take, patient outcomes have been found to be accurately predicted by prognostic factors evaluated in all TBI patients, including presence of cisternal effacement or skull fracture, status of pupillary exam, and brainstem reflexes, respiratory status, and the patient’s age and GCS score. Other studies have noted a relation between outcome and amount of edema, hematoma size, or clinical decline prior to surgery. Andrews et al found a positive correlation between an intraparenchymal bleed greater than 30 mL in size located in the temporal or temporoparietal region and the development of herniation or brainstem compression.92 Again, this study concluded that this correlation, in and of itself, should not be used in determining surgical versus nonsurgical management.
Determining when a patient should go to surgery is one of the most important decisions a neurosurgeon can make. Studies have noted a negative correlation between nonoperative management and multiple findings. These include hypoxia, effacement of the cisterns, presence of SAH, and intracranial hypertension. Bullock et al, in an attempt to determine the need for surgery, evaluated patients with ICP monitoring.93 Although unable to predict clinical decline over a longer time frame, the study did find a positive correlation between peak ICP and the need for surgical evacuation. This study also concluded that the decision to proceed with surgery should be based on clinical and radiographic findings, in addition to ICP values. The timing of surgery can be as important as the decision to go to surgery. Mathiesen et al showed improved outcome in patients who underwent surgery prior to neurological decline.94 The outcome was even better in those patients with radiographic evidence of mass effect or midline shift.
One reason it is difficult to determine appropriate patient care based solely on the radiographic findings of a parenchymal lesion is due to the fact that CT scans provide only a “snapshot” of a lesion. As Yamaki et al showed, only a little over 80% of intraparenchymal bleeds enlarged to their maximal volume within 12 hours of the initial injury; furthermore, for hemorrhages greater than 3 cm in size, only a little under 60% presented within 6 hours.95 The development of these lesions follows a fluid course, making decisions based on a head CT uncertain.
Conventionally, delayed traumatic intracerebral hematoma (DTICH) occurs in portions of initially unremarkable brain in patients with abnormal CT scans; some stated that these hematomas develop at sites of prior contusion. DTICH is found in up to 7.4% of all TBI patients, with a mortality ranging up to 72%.
Two studies showed adverse effect on outcome when DTICH developed within 48 hours of the original injury.95,96 It is associated with a variety of other findings, including coagulopathy; interestingly, there is a higher incidence of DTICH in those patients who have undergone surgery for separate lesions.
Several surgical procedures, including temporal lobectomy, subtemporal decompression, hemispheric decompressive craniectomy, and bifrontal decompressive craniectomy, can be utilized. These procedures are used primarily for the treatment of medically refractory intracranial hypertension. Several studies, utilizing human and animal trials, found significant reductions in ICP following decompressive frontoparietal craniectomy. Gower et al noted, on average, a 34% decrease in ICP for patients who underwent subtemporal decompression.97
Fifty-seven percent of patients who underwent a decompressive craniectomy for refractory intracranial hypertension were found to have a favorable outcome at 1 year in one study98; these findings may be limited secondary to the exclusion of many patients with significant associated disease. Two fifths of patients were found to be functionally independent following temporal lobectomy; surgery was performed on patients with transtentorial herniation, within 2 hours of the onset of symptoms. Guerra et al noted that up to 65% of patients who underwent a decompressive craniectomy for diffuse brain swelling refractory to medical management had a good outcome at 1 year.99
Evaluating the multiple types of parenchymal injuries and different means of treating them, several options for treatment are available to the clinician. These include craniotomy for the evacuation of mass lesions in patients with neurological decline, mass effect on CT, midline shift greater than 5 mm, cisternal effacement, lesion volume greater than 50 mL, and a frontal or temporal contusion greater than 20 mL in size in a patient with a GCS score of 6 to 8. With the presence of cerebral edema and intracranial hypertension refractory to medical management, a bifrontal decompressive craniectomy within 48 hours of the injury may be performed. Subtemporal decompression, temporal lobectomy, and hemispheric decompressive craniectomy may be used for the management of transtentorial herniation as well as for intracranial hypertension refractory to medical management.
Nonoperative management may be appropriate in patients with stable neurological exams, normal ICPs, and no radiographic evidence of mass effect.
General Pediatric Trauma Management Guidelines
Table 16-3 summarizes the guidelines for pediatric trauma management.
Trauma Systems, Pediatric Trauma Centers, and the Neurosurgeon
A greater proportion of pediatric than adult trauma involves TBI. However, surprisingly little substantial research has been conducted to specify standard treatment protocols for acute care and inpatient and outpatient rehabilitation of our youngest patients. This is partly due to the difficulty in evaluating treatments across age groups and developmental phases for children with TBI. However, because it is now widely recognized that children are not just “little adults,” it is not proper to generalize from the adult literature to the pediatric population. The following guidelines are intended to provide some guidance from the peer reviewed literature to pediatric physicians.
There are studies that have demonstrated decreased mortality with implementation of trauma systems and the use of pediatric trauma centers.100 However, recent research indicates that certain subgroups are not helped. There was, for example, a significant increase in deaths from TBI from pre- to postimplementation of Oregon’s trauma system for patients injured in rural areas and transferred to a higher level of care.101 This may be because trauma system protocols for quick transfer may have unintentionally resulted in subjecting unstable patients to premature transfer.
Data appear to support the conclusion that pediatric patients with severe TBI are more likely to survive if treated in pediatric trauma centers, or adult trauma centers with added qualifications in pediatrics rather than in a level I or level II adult trauma center, and that pediatric patients with severe TBI who require neurosurgical procedures have a low chance of survival in a level II adult trauma center as compared with the other centers.9 Additionally, in metropolitan areas, direct transport to a pediatric trauma center appears to increase survival rate overall.102
Treatment Modality | Guidelines |
Trauma systems | Standards—Insufficient data Guidelines—Pediatric TBI patients are best served at a pediatric trauma center Options—Pediatric TBI patients are best served at a pediatric trauma center or an adult trauma center with qualifications for managing children |
Prehospital airway management | Standards—Insufficient data Guidelines—Hypoxia should be treated as efficiently and effectively as possible Options—End-tidal PaCO2 monitoring should be utilized for those patients requiring endotracheal intubation |
Resuscitation of blood pressure and oxygenation | Standards—Insufficient data Guidelines—Hypotension (value is age dependent) must be avoided. Options—In patients with poor neurological exams (GCS ≤to 8) the airway should be secured |
Indications for intracranial pressure monitoring | Standards—Insufficient data Guidelines—Insufficient data Options—ICPs may be monitored in children with a GCS score ≤ to 8 |
Intracranial pressure treatment threshold | Standard—Insufficient data Guidelines—Insufficient data Options—Treatment should begin with ICPs ≤ 20 mm Hg |
Recommendations for intracranial pressure monitoring technology | Standard—Insufficient data Guidelines—Insufficient data Options—The ventricular catheter will provide the most accurate values |
Recommendations for cerebral perfusion pressure | Standards—Insufficient data Guidelines—Cerebral perfusion pressure should be maintained > 40 mm Hg Options—Outcomes may improve when cerebral perfusion pressures are maintained at 40 to 65 mm Hg |
Hyperventilation | Standards—Insufficient data Guidelines—Insufficient data Options—Although prophylactic hyperventilation (PaCO2 < 35 mm Hg) is not recommended, mild (PaCO2 < 30–35) or aggressive (PaCO2 < 30 mm Hg) hyperventilation may be used for refractory intracranial hypertension or acute neurological decline |
The use of mannitol/hyperosmolar therapy | Standards—Insufficient data Guidelines—Insufficient data Options—Hypertonic saline (3% at 0.1–1.0 mL/kg of body wt/hr) and mannitol (0.25–1.0 g/kg of body weight) can be effective in managing elevated ICPs Euvolemia should be maintained; for those on mannitol, serum osmolarity should be below 320 mOsm, whereas those on hypertonic saline, or hypertonic saline and mannitol, should be below 360 mOsm |
The use of barbiturates in the control of intracranial hypertension | Standards—Insufficient data Guidelines—Insufficient data Options—Reserved for patients with refractory intracranial hypertension |
The role of steroids | Standards—Insufficient data Guidelines—Insufficient data The use of steroids can predispose patients to infection secondary to the reduction of cortisol production Options—Steroids not recommended for use in TBI patients |
Nutrition | Standards—Insufficient data Guidelines—Insufficient data Options—Nutrition administration should begin within 3 days of injury; a goal of 130–160% of resting metabolic use should be provided by the seventh day postinjury |
The role of antiseizure prophylaxis following head injury | Standards—Insufficient data Guidelines—Anticonvulsants for the treatment of late seizures is not recommended Options—Anticonvulsants may be considered for the prevention of early seizures |
Use of sedation and neuromuscular blockade in the treatment of severe TBI | Standards—Insufficient data Guidelines—Insufficient data Options—With limited data available, the use of sedation and neuromuscular blockade is left to the discretion of the physician |
The role of cerebrospinal fluid drainage in the treatment of severe pediatric TBI | Standards—Insufficient data Guidelines—Insufficient data Options—Drainage via ventriculostomy may be considered for the treatment of elevated intracranial pressures (no numerical parameters provided); a lumbar drain may be added for refractory intracranial hypertension in patients without effacement of the basal cisterns or the presence of a mass lesion or midline shift |
The role of temperature control following severe pediatric TBI | Standards—Insufficient data Guidelines—Insufficient data Options—Although hyperthermia should be avoided, hypothermia may be considered for refractory intracranial hypertension |
Surgical treatment of pediatric intracranial hypertension | Standards—Insufficient data Guidelines—Insufficient data Options—Decompressive craniectomy may be considered in those with refractory intracranial hypertension; outcome may be improved if the surgery is completed within 48 hours of the injury |
GCS, Glasgow Coma Scale; ICP, intracranial pressure; TBI, traumatic brain injury.
Prehospital Airway Management
It is known that hypoxemia in the prehospital setting is associated with worse outcomes in TBI patients.3 Additionally, several studies have suggested that hypoxia during the prehospital care of pediatric patients with TBI is common.8 As many as nearly a third of pediatric patients with severe TBI are hypoxic on arrival to the emergency department.103 Therefore, it may be tempting to advocate immediate endotracheal intubation for all pediatric patients with severe TBI and signs of hypoxia in the field. However, two large, randomized, prospective studies, including one using the National Pediatric Trauma Registry, demonstrated no significant difference in outcome among those managed with endotracheal intubation and those treated with bag mask ventilation in the field.104 A smaller study of 16 pediatric patients intubated in the field demonstrated four deaths related to “major airway mishaps.”105 Therefore, although there is clear evidence that hypoxemia leads to poorer neurological outcome in both pediatric and adult TBI patients, and that hypoxia frequently occurs in the prehospital setting in this patient population, there is also evidence that successful prehospital intubation of infants and children requires specialized training, and that success rates are in general lower than in adults.
Resuscitation of Blood Pressure and Oxygenation and Prehospital Brain-Specific Therapies
Multiple studies have demonstrated the negative outcomes related to hypoxia and hypotension in both the adult and the pediatric population with TBI. One study by Pigula et al analyzed the influence of hypoxia and hypotension on mortality from severe TBI.106 They reported an 18% incidence of hypotension on arrival to the emergency department. A mortality rate of 61% was associated with hypotension on admission versus 22% among patients without hypotension. When hypotension was combined with hypoxia, the mortality rate was 85%.
The adult neurosurgical literature traditionally defines hypotension as systolic blood pressure <90 mm Hg. In pediatric patients, hypotension is defined as less than the fifth percentile of normal systolic blood pressure for age. In children, however, hypotension is a late sign of shock. Pediatric patients may maintain their blood pressure despite significant hypovolemia and clinical signs of shock. Signs of diminished perfusion include tachycardia, loss of central pulses, diminished urine output to less than 1 mL/kg/hr, or increased capillary filling time >2 seconds. Fluid resuscitation is indicated in children for clinical signs of decreased perfusion even when an adequate blood pressure is observed. Fluid restriction to avoid cerebral edema or exacerbating cerebral edema is contraindicated in the management of the TBI pediatric patient in shock.107 Shock is rarely due to TBI alone; therefore, evaluation for internal or spinal cord injury is indicated.108
Apnea and hypoventilation are common in both adult and pediatric patients with severe TBI. Hypoxia may be defined as a PaO2 <60 to 65 torr or O2 saturation <90%. Hypoxia develops more rapidly in children than in adults during apnea or hypoventilation. Respiratory rate and effort should be monitored and corrected to age-appropriate levels. When available, oxygen saturation should be monitored on all patients with severe TBI continuously or as frequently as possible.
Sedation, analgesia, and neuromuscular blockade may be useful to optimize transport of the patient with TBI; however, because no outcome studies provide guidance on the use of these adjuncts, the timing and choice of agents are best left to the local emergency medical services (EMS) protocols.109 The prehospital use of mannitol to lower ICP is an area that requires further study. However, it appears that mannitol is an effective but potentially hazardous method of lowering ICP and that its use during the prehospital period should be limited to the euvolemic patient with evidence of cerebral herniation.
There are no studies on the effect of outcome on the use of hyperventilation during the prehospital period. Based on adult studies, recommendations include hyperventilation as the first line of intervention in the patient with suspected cerebral herniation.109 Prophylactic hyperventilation is not indicated.
Indications for Intracranial Pressure Monitoring
ICP monitoring is appropriate in infants and children with severe TBI. Data obtained from a study of predominantly adult patients demonstrate that detection and treatment of intracranial hypertension may protect cerebral perfusion, avoid cerebral herniation, and improve neurological outcome.14,110
ICP monitoring is not routinely indicated in infants and children with mild or moderate head injury. However, a physician may choose to monitor ICP in certain conscious patients with traumatic mass lesions or in those in whom the administration of sedation or neuromuscular blockage precludes serial neurological exams. The relative importance in avoiding increased ICP (generally >20 mm Hg) and reduced CPP remains uncertain. However, strong evidence indicates that significant changes in either value are likely to be deleterious, related to changes in the other, and with poor outcome.
Continuous monitoring combined with aggressive ICP-directed therapy has resulted in the best historical outcomes in the treatment of severe pediatric TBI. Various studies using three different interventional strategies for ICP control have produced similar and convincing reductions in the expected mortality and neurological morbidity rate. These strategies include decompressive craniectomy,111,112 hyperosmolar therapy,113 and hyperventilation therapy.114 Additional investigations have demonstrated the efficacy of decompressive craniectomy in improving neurological outcome in patients with medically refractory intracranial hypertension from trauma in infants and toddlers.111,112 Given the low risk of ICP monitoring, the data indicate a clear benefit for ICP monitoring in severe pediatric TBI.
Predicting those children with TBI at risk for increased ICP is more difficult than it is for adults. Although GCS scores and the neurological exam remain the standards for clinical evaluation of patients with TBI, these are less sensitive in infants and young children. Imaging correlates of intracranial hypertension, such as effacement of the basal cisterns of CT, can be misleading in children. The clinical evaluation of infants with TBI can be difficult, and a negative initial head CT does not exclude the possibility of increased ICP. The presence of open fontanels and sutures in an infant with severe TBI does not preclude the development of intracranial hypertension or negate the utility of ICP monitoring. Therefore, it is appropriate to monitor ICP in all infants suffering severe TBI as well as all pediatric patients with TBI undergoing sedation, neuromuscular blockade, or anesthesia for management of extracranial injuries.
Continuous ICP data allow the management of severe head injury by objective criteria. This is important because the medical and surgical treatments for intracranial hypertension have significant potential adverse consequences. Therefore, ICP monitoring allows the judicious use of interventions such as hyperosmolar therapy, sedatives, paralytics, barbiturates, and ventilator management with a defined end point that is correlated with clinical outcome.
Threshold for Treatment of Intracranial Hypertension
Specific thresholds of ICP for institution of therapy in children with severe TBI have not been established. It is known that prolonged periods of intracranial hypertension or large increases in ICP are associated with poor outcome. Pfenninger et al retrospectively reviewed the monitoring of ICP in 24 patients with severe TBI. They used a definition of ICP elevation as >20 to 25 mm Hg. The goal of treatment was to maintain ICP <20 mm Hg and abolish ICP elevations >25 to 30 mm Hg. They found sustained ICP >40 mm Hg associated with death, ICP between 20 and 40 mm Hg associated with moderate outcome, and ICP <20 mm Hg associated with good outcome.115 Although pediatric patients with ICP >30 mm Hg may be treated successfully with medical treatment only, there is clearly a role for decompressive craniotomy in patients with ICP >30 mm Hg.112
The adult guidelines recommend initiation of ICP treatment at an upper threshold of 20 to 25 mm Hg.116 One multicenter, double blind, controlled study demonstrated improved outcome when ICP could be controlled by using a threshold of 20 mm Hg.44 Additionally, patients may herniate at ICPs <20 to 25 mm Hg. Therefore the choice of any threshold must be closely and repeatedly corroborated with the clinical exam and radiological findings in an individual patient.
Recommendations for Intracranial Pressure Monitoring Technology
In those pediatric patients for whom ICP monitoring is indicated, the clinician must decide what type of monitoring device to use. The optimal ICP monitoring device is safe, accurate, reliable, and cost effective. Current ICP monitors allow pressure transduction by external strain gauge, catheter tip strain gauge, and catheter tip fiberoptic technology.
External strain gauge transducers are coupled to the patient’s intracranial space via fluid-filled lines, whereas catheter tip transducers are placed intracranially. External strain gauge transducers are accurate and can be recalibrated, but obstruction of the fluid can result in inaccurate readings. Additionally, the external transducer must be maintained at a fixed reference point relative to the patient’s head to prevent a measurement error.
Catheter tip strain gauge or fiberoptic devices are calibrated before intracranial insertion and cannot be recalibrated once inserted without an associated ventricular catheter. Therefore, they are vulnerable to device measurement drifts, especially if the device is used over a long period of time. Adult studies have, however, demonstrated negligible drift over 5 days of use.116
In pediatric patients who require ICP monitoring, a ventricular catheter and/or external strain gauge transducer or catheter tip pressure transducer device is an accurate and reliable method of monitoring ICP. A ventriculostomy catheter also enables therapeutic CSF drainage. Clinically significant infections associated with ICP devices causing significant patient morbidity are rare and should not deter the decision to monitor ICP.117
Recommendations for Cerebral Perfusion Pressure
Global or regional cerebral ischemia is an important secondary insult to the acutely injured brain. The CPP is defined as the mean arterial pressure minus the ICP. This defines the pressure gradient driving CBF, which is related to the metabolic delivery of essential substrates. The posttraumatic brain has a significant incidence of vasospasm, which may increase the cerebral vascular resistance, reducing the CPP and resulting in ischemia. With the use of continuous monitoring capabilities, including invasive blood pressure and ICP equipment, the CPP could be manipulated in an attempt to avoid regional and global ischemia.
It is well known that CBF declines following TBI and may frequently reach the ischemic threshold for brain tissue. Regional CBF may be even more reduced in the vicinity of intracranial hematomas and contusions. There is debate on how best to measure CBF and at what threshold there is actual tissue ischemia. CPP is relatively easy to measure and appears to correlate well with CBF when measured.
A low CPP is highly correlated with poor outcome, but there is less evidence that manipulating the CPP can change eventual neurological outcome in both adults and children. A CPP <40 mm Hg is consistently associated with increased mortality, independent of age. It is unclear whether this value represents a minimal threshold or whether the optimal CPP may be above this in children (e.g., 50 to 65 mm Hg). No study demonstrates that active maintenance of CPP above any target threshold in pediatric TBI is responsible for improved mortality or morbidity.
Use of Sedation and Neuromuscular Blockade in Treatment of Severe Pediatric Traumatic Brain Injury
Despite the common use of sedatives, analgesics, and neuromuscular blocking agents in pediatric patients with severe TBI both for emergency intubation and for management including control of ICP, there has been little formal clinical investigation performed regarding these practices. The lack of high-quality pediatric studies severely limits any conclusions that can be made.
Nevertheless, the use of sedatives, analgesics, and neuromuscular blocking agents can facilitate necessary general aspects of patient care such as the ability to maintain the airway, vascular catheters, and other monitors. Additionally, because pain and stress markedly increase cerebral metabolic demands and can pathologically increase cerebral blood volume and raise ICP, sedatives and analgesics may be useful in mitigating these aspects of secondary damage. In addition to treating noxious stimuli, they also facilitate mechanical ventilatory support, have anticonvulsant and antiemetic actions, prevent shivering, and help mitigate the long-term psychological trauma of pain and stress.
Neuromuscular blocking agents have been suggested to reduce ICP by a variety of mechanisms, including reduction in airway and intrathoracic pressure with facilitation of cerebral venous outflow and prevention of shivering, posturing, or bucking the ventilator.5 Risks of neuromuscular blockade include hypoxemia secondary to inadvertent extubation, risks of masking seizures, increased incidence of nosocomial pneumonia, cardiovascular side effects, immobilization stress, and increased ICU length of stay.118
Until experimental comparisons between specific regimens of these sedative, analgesic, and neuromuscular blocking agents are performed, the choice and dosing of sedatives and analgesic agents for the management of infants and children with severe TBI should be left to the treating physician.
Role of Cerebrospinal Fluid Drainage in the Treatment of Severe Pediatric Traumatic Brain Injury
In children with severe TBI and intracranial hypertension, ventricular CSF drainage is a commonly employed therapeutic modality in conjunction with ICP monitoring. The role of CSF drainage is to reduce intracranial fluid volume and thereby lower ICP. With the use of a ventriculostomy as a common means of measuring the ICP of patients with TBI, the potential therapeutic benefits of CSF drainage became of interest. However, CSF drainage is not limited to the ventricular route. Controlled lumbar drainage has resulted in improved outcomes in the pediatric population with severe TBI and intracranial hypertension.119,120
In summary, ventricular CSF drainage in severe pediatric TBI is supported as a treatment option in the setting of refractory intracranial hypertension, and the addition of lumbar drainage in patients showing open cisterns on imaging and without major mass lesions or shift is also supported.
Use of Hyperosmolar Therapy in the Management of Severe Pediatric Traumatic Brain Injury
Intravenous administration of hyperosmolar agents has been shown to reduce ICP. Mannitol is a cornerstone in the management of increased ICP in pediatric and adult TBI. It can reduce ICP by two distinct mechanisms. Mannitol rapidly reduces ICP by reducing blood viscosity with a resultant decrease in blood vessel diameter. This occurs as a result of CBF autoregulation. The level of CBF is maintained, despite a reduction in blood viscosity, through reflex vasoconstriction. Thus, cerebral blood volume and ICP decrease. The effect of mannitol on blood viscosity is rapid albeit brief (75 min). Mannitol also reduces ICP by an osmotic effect, which develops more slowly (15 to 30 min) due to the gradual movement of water from the parenchyma into the circulation. This effect persists up to 6 hours and requires an intact blood-brain barrier. Mannitol may accumulate in injured brain regions where a reverse osmotic shift may occur, with fluid moving from the intravascular compartment into the brain parenchyma, possibly increasing the ICP. This phenomenon has been suggested to be most marked when mannitol is present in the circulation for extended periods of time, supporting the use of intermittent boluses. Mannitol has been deemed to be effective in controlling increased ICP after severe TBI, with effective doses ranging from 0.25 g/kg to 1 g/kg of body weight. Mannitol is excreted unchanged in the urine, and a risk of the development of acute tubular necrosis and renal failure has been suggested with mannitol administration with serum osmolarity levels >320 mOsm in adults.
Like mannitol, the penetration of sodium across the blood-brain barrier is low. Sodium thus shares both the favorable rheological and osmolar gradient effects involved in the reduction in ICP by mannitol. Hypertonic saline also exhibits several theoretical beneficial effects, including restoration of normal cellar resting membrane potential and cell volume,121 stimulation of atrial natriuretic peptide release,122 inhibition of inflammation, and enhancement of cardiac output.123 Possible side effects of hypertonic saline include a rebound in ICP, central pontine myelinolysis, and SAH. Administration of hypertonic saline is effective for control of increased ICP after severe head injury. Effective doses as a continuous infusion of 3% saline range between 0.1 and 1 mL/kg of body weight per hour, administered on a sliding scale. The minimum dose needed to maintain ICP <20 mm Hg should be used.
Until one or more direct comparisons between mannitol and hypertonic saline administration in pediatric patients with TBI and elevated ICP are performed, the choice of either agent is a matter of physician preference.
Use of Hyperventilation in the Acute Management of Severe Pediatric Traumatic Brain Injury
Aggressive hyperventilation therapy has been used in the management of severe pediatric TBI for the rapid reduction of ICP for the last 20 years. This approach has been based on the assumption that hyperemia was common after pediatric TBI. Hyperventilation therapy was also believed to be beneficial through other mechanisms, including reduction of brain acidosis, improvement of cerebral metabolism, restoration of autoregulation of CBF, and increasing perfusion to ischemic areas.
However, more recent pediatric studies demonstrate that hyperemia is uncommon and have also raised concerns about the safety of hyperventilation therapy.124 Hyperventilation reduces ICP by inducing hypocapnia leading to cerebral vasoconstriction and ultimately a reduction in CBF. This reduces cerebral blood volume and decreases the ICP. Hyperventilation may also induce ischemia. Chronic hyperventilation depletes the brain tissue of interstitial bicarbonate buffering and causes cerebral circulation to become hyperresponsive to subsequent increases in PaCO2. Additionally, the respiratory alkalosis that results from hyperventilation causes a left shift of the hemoglobin-oxygen dissociation curve, which may impair delivery of oxygen to tissue. The effect of hyperventilation therapy on outcome of infants and children with severe TBI has not been compared directly with other therapies such as hyperosmolar agents, barbiturates, hypothermia, or early decompressive craniectomy.
Mild or prophylactic hyperventilation (PaCO2 <35 mm Hg) in children should be avoided. Mild hyperventilation (PaCO2 30 to 35 mm Hg) may be considered for longer periods for intracranial hypertension refractory to sedation and analgesia, neuromuscular blockage, CSF drainage, and hyperosmolar therapy. Aggressive hyperventilation (PaCO2 <30 mm Hg) may be considered as a second-tier option in the setting of refractory hypertension. Close monitoring of CBF, jugular venous oxygen saturation, or brain tissue oxygen monitoring is suggested to help identify cerebral ischemia in this setting.
The Use of Barbiturates in the Control of Intracranial Hypertension in Severe Pediatric Traumatic Brain Injury
The use of barbiturates to treat elevated ICP in children with severe head injury has been reported since the 1970s.40 The ICP-reducing and direct neuroprotective properties of barbiturates have prompted the investigation of their use in the management of patients with TBI. Several therapeutic regimens have been reported. Eisenberg et al44 used the following protocol for pentobarbital:
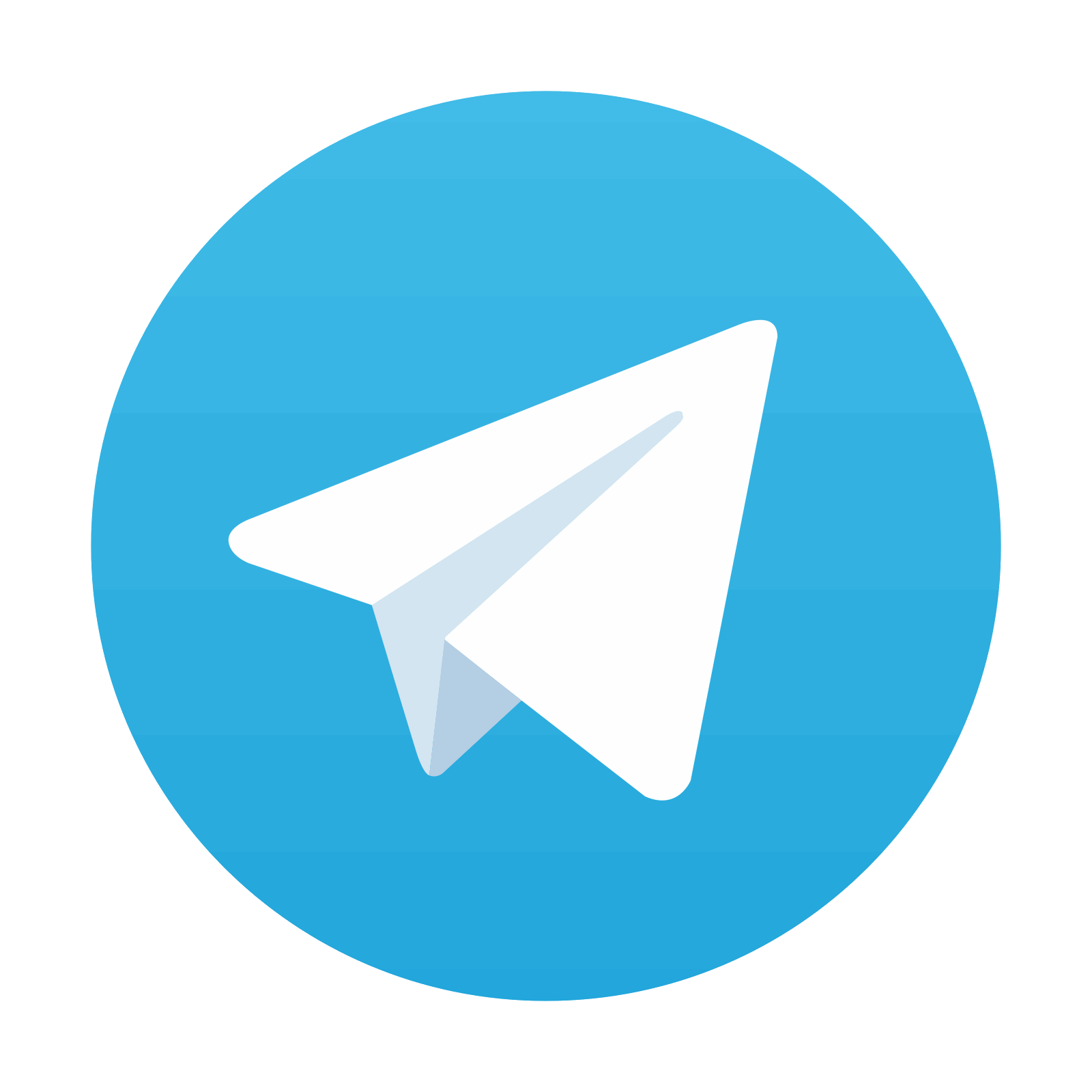