Abstract
Most people experience trivial movements of their facial muscles during daily life. These are often barely recognizable, but they sometimes imply pathological conditions that require appropriate treatments. Hemifacial spasm (HFS) is a representative movement disorder affecting the face. It is a common condition that can cause discomfort and cosmetic problems, as well as psychological impairments. Fortunately, remarkable advancements in treatment have been achieved by studying HFS. In this chapter, the authors review the current knowledge of HFS and its optimal treatment strategies, focusing on surgical options. In addition, Meige syndrome is summarized as an important differential diagnosis with a completely different etiology and management strategy, including deep brain stimulation.
Keywords
Deep brain stimulation, Hemifacial spasm, Meige syndrome, Microvascular decompression
Outline
Hemifacial Spasm 953
Introduction 953
Etiology and Pathophysiology 954
Clinical Manifestations 954
Diagnosis 955
Management 957
Operative Technique 957
Patient Positioning 957
Scalp Incision to Craniectomy 957
Dural Incision and Exposure 958
Decompression of the Offending Vessel 958
Closure 958
Intraoperative Monitoring 958
Surgical Outcome 959
Complications 959
Conclusions 960
Meige Syndrome 960
Introduction 960
Clinical Manifestations and Pathophysiology 960
Management 960
Operative Techniques of Globus Pallidus Interna Deep Brain Stimulation 962
Outcome and Complications 962
Conclusions 962
References 962
Hemifacial Spasm
Introduction
Hemifacial spasm (HFS) is a unilateral, involuntary, paroxysmal movement of facial muscles innervated by the ipsilateral facial nerve. Its characteristic features include tonic–clonic contractions and synkinesis of the muscles. These symptoms usually start from the orbicularis oculi muscle and gradually spread inferiorly to the muscles around the cheek, mouth, and neck. Eventually the facial spasm worsens in frequency and severity, resulting in sustained muscle contractions and a disfiguring grimace ( ).
In Schultze first reported the concept of vascular compression in a 56-year-old man with left HFS, revealing at autopsy a giant aneurysm from the left vertebral artery that was compressing the facial nerve root. Gowers described the classical clinical features of HFS in , and the terminology of “Hémispasme facial périphérique” was initially used by Babinski in . Gardner reported his first case of HFS treated by posterior fossa vascular decompression surgery in . In the 1970s Jannetta expanded Gardner’s neurovascular compression theory and established the microvascular decompression (MVD) procedure as a standard treatment for HFS ( ). Since then detailed surgical techniques for MVD have advanced, with the development of neuroimaging and intraoperative monitoring in the pursuit of better outcomes and fewer complications ( ).
Etiology and Pathophysiology
HFS can be divided into two types according to the cause of the disease: primary or secondary. Primary HFS is approximately four times more frequently presented than secondary HFS ( ). Primary HFS is mainly precipitated by aberrant vascular compression at the root exit zone of the seventh cranial nerve ( ). Compression of the nerve by any artery or vein can elicit clinical symptoms in the affected patients. The most common offending vessels are the posterior inferior cerebellar or anterior inferior cerebellar arteries, followed by the vertebral artery or superior cerebellar artery ( ). In contrast, secondary HFS is caused by facial nerve damage from any other cause, such as cerebellopontine angle tumor, vascular malformation, or a demyelinating condition (e.g., multiple sclerosis) ( ) ( Table 78.1 ).
Cerebellopontine angle tumors: acoustic neuroma, meningioma |
Epidermoid, arachnoid cyst, lipoma |
Arteriovenous malformation, cerebral aneurysm |
Brainstem lesions: stroke, trauma, demyelinating disorders |
Infections |
Structural abnormalities of the posterior cranial fossa: chiari malformation |
Two different hypotheses have been accepted regarding the underlying pathophysiology of HFS. The ephaptic transmission hypothesis (the peripheral hypothesis) states that close vascular contact with a portion of the “transitional zone,” 1.9–2.5 mm between the central (oligodendrocytes) and peripheral (Schwann cell) types of myelin, can cause myelin breakdown, thereby allowing abnormal neural firing in injured axons to communicate aberrantly to the adjacent nerve fibers ( ). In particular, the cranial nerves in the transitional zone are only protected by the arachnoid membrane because of a lack of epineurium, and thus are vulnerable to mechanical injury ( ). The second hypothesis, the hyperactivity hypothesis (the central hypothesis), states that abnormal hyperexcitability of the facial motor nucleus results from vascular irritation to the peripheral facial nerve as well as to the nucleus itself ( ). Recently a sympathetic hypothesis came to the fore, suggesting that neurotransmitters released from sympathetic nerve endings in the adventitia of the offending arteries induced ectopic action potentials in nerve fibers and consequent involuntary muscle contractions ( ). One new study suggests that HFS is related to the reorganization of the gray and white matter, based on viewing reduced or increased gray-matter volume in a specific cortex on voxel-based morphometry and diffusion tensor imaging ( ).
Clinical Manifestations
The prevalence of HFS is 7.4–14.5 per 100,000 persons per year ( ). Most patients are middle aged, with a mean age of onset of 44 years. HFS rarely occurs in young patients (1%–6%), although when it does it assumes a similar course and progress as in adults ( ). Females are about twice as susceptible to HFS as males ( ). The majority of HFS appears on one side of the face, and less than 5% of cases occur on both sides ( ). Generally, HFS is triggered or aggravated by emotional stress, fatigue, psychiatric tension, or anxiety ( ). Although it is still controversial, some studies suggest that arterial hypertension is associated with HFS. Hypertension contributes to the intravascular hemodynamic changes resulting in vessel ectasia, and a strong correlation between hypertension and ventrolateral medulla compression has been found ( ).
Typical HFS begins at the lower eyelid, gradually progressing to the entire oculi muscles and those in the lower face, including the orbicularis oris and platysma. Atypical HFS, a less common type, is associated with a facial contraction that spreads in the opposite direction, from the lower portion up to the frontalis muscles. Although these two entities usually show different compression sites of the facial nerve at the brainstem (typical HFS is associated with compression at the antero-caudal aspect), all types of HFS are regarded as a type of neurovascular compression syndrome and managed in the same way ( ). In severe cases paroxysmal and persisting contraction of facial muscles causes functional blindness in the affected eye, insomnia, an annoying clicking sound from involving the stapedius muscle, and eventually cranial nerve deficits such as hearing loss or facial palsy ( ). HFS usually has a progressive course, and spontaneous resolution is generally not expected ( ).
Diagnosis
Clinical Diagnosis
The diagnosis of HFS is made based on the clinical symptoms. Synchronized upper facial muscle contractions are the main feature of HFS diagnosis and are induced by a simple physical maneuver, like lifting the ipsilateral eyebrow with eye closure (the other Babinski sign) ( ). Furthermore, HFS mimics other craniofacial dyskinesias, including blepharospasm, tics, synkinesis, myokymia, craniocervical dystonia, and partial seizures ( ). Psychogenic HFS is rare, occurring in 2.4% of patients, who do not require surgical treatment ( ). It is very important to differentiate HFS from other similar diseases ( ).
- •
Blepharospasm is characterized by bilateral synchronous contractions of the muscles around the eye. It differs from HFS in being bilateral, and involves only the musculature around the eye rather than presenting with steady progression down the face, as in HFS.
- •
Tics are brief, repetitive, stereotyped, and involuntary movements. Tics are similar to the movements of habitual spasms like blinking, and are associated with more tonic components than HFS.
- •
Facial myokymia is characterized by unilateral, undulating, worm-like, continuous muscle contractions associated with intrinsic brainstem pathology, which has a distinct and diagnostic electromyographic pattern. The symptoms disappear spontaneously in most cases within a few weeks.
- •
Postparalytic synkinesis may occur after aberrant regeneration of the facial nerve following Bell’s palsy. A history of antecedent Bell’s palsy, with these movements developing on regeneration of the nerve, is helpful in differentiating this condition.
- •
Tardive dyskinesia is associated with a history of exposure to dopaminergic antagonists or other neuroleptic drugs. It usually presents as stereotypical movements of the face, especially around the lips and neck.
Neuroradiologic Evaluations
Neuroradiologic examinations are usually required to confirm the diagnosis and detect the cause of HFS. In most patients the pathogenesis of HFS is attributed to neurovascular compression of the facial nerve at the root exit zone; however, a minority of patients with HFS may have secondary causes like a space-occupying lesion or demyelination, which must be ruled out ( ). Of the various modalities, high-resolution three-dimensional (3D) T2-weighted sequences of magnetic resonance imaging (MRI), including 3D balanced steady-state free precession (SSFP) imaging such as constructive interference in the steady state (CISS) or fast imaging employing steady-state acquisition, are useful to assess the location of the neurovascular compression ( ). These are imaging methods combined with fast-gradient echo that have various advantages, such as a high signal-to-noise ratio, high spatial resolution, and less cerebrospinal fluid (CSF) flow artifact ( ). Three-dimensional time-of-flight (3D TOF) magnetic resonance angiography (MRA) can assist in evaluation of the anatomical characteristics of the vascular structures at the root exit zone ( ) ( Fig. 78.1 ), and 3D SSFP and TOF MRA have a high accuracy in detecting the offending vessels compressing the facial nerve ( ). Fusion imaging of 3D magnetic resonance cisternograms and angiograms is also useful for the preoperative assessment for MVD in patients with HFS to show the relationship between the vessel and nonvascular soft tissues clearly ( ). Three-dimensional virtual endoscopy not only provides excellent views of the neurovascular structure but also enables preoperative simulations of the surgery ( ). Recently, with the development of imaging techniques and software, 3D tubular models make it possible to understand preoperative 3D neurovascular structures at a level that nearly matches the intraoperative findings ( ).

In addition, 3D TOF MRA can describe the postoperative state of the decompression site between the vessels and the root exit zone. These findings may be helpful to guide further management in patients with persistent or recurrent HFS after MVD ( ). In our institution we routinely use 3D TOF MRA and CISS imaging for the preoperative evaluation of patients with HFS, and if needed these images are acquired to ascertain whether adequate decompression was performed in failure cases ( Fig. 78.2 ).

Additional Methods
Electromyography (EMG) can differentiate HFS from other abnormal movement disorders with a muscle or denervation origin. Electrophysiological findings such as detecting lateral spread are an important hallmark providing additional information to make a diagnosis and determine whether adequate decompression has been achieved during surgery. Preoperative audiologic examinations including pure tone audiometry and tympanometry are informative to make a decision on surgery. If a patient has a hearing impairment on the contralateral side of the HFS, risking hearing loss, the surgeon has to contemplate performing the MVD or make a vigilant preoperative plan. Detailed information is given below in the subsection on intraoperative monitoring.
Management
Medical Treatment
A number of drugs have been found to be helpful for HFS, including anticonvulsants, anticholinergics, muscle relaxants like baclofen, and haloperidol ( ). The drugs commonly have limitations in terms of their tolerance and subsequent side-effects. Botulinum neurotoxin has been indicated since the early 1980s as the treatment of choice for patients who refuse MVD and those with high surgical risks. The therapeutic effects start to show in 3–6 days after injection. A successful outcome has been reported in up to 75%–100% of cases ( ). However, the injections must be repeated every 3–6 months, resulting in a high cost for the therapy ( ). Long-term use of the botulinum neurotoxin also has the risk of muscle weakness leading to facial palsy, diplopia, or ptosis ( ).
Microvascular Decompression
MVD of the facial nerve is one of the standard treatments to alleviate neurovascular contact. It can offer a complete cure and has excellent and prolonged symptom relief, with success rates of 83%–99% ( ). Since there are a variety of operative techniques involving MVD, depending on the surgeon’s preference and the patient’s condition, we describe our own MVD techniques here. The main aspects of our techniques are sharp arachnoid dissection and minimal cerebellar retraction to achieve MVD with optimal safety and effectiveness. Neuroendoscopy can be used to provide a more accurate view of neurovascular conflicts and confirm the completeness of the decompression in cases with limited microscopic view ( ). In rare cases HFS appears with the cisternal portion of the facial nerve being indented by the offending vessels ( ). The outcomes of MVD in HFS cases with distal compression are statistically similar when compared with proximal compression ( ), so it is recommended that neurosurgeons keep in mind the possibility of the compressing vessel existing at a distal part of the facial nerve when a likely candidate is not detected at the conventional root exit zone on preoperative evaluation.
Operative Technique
Patient Positioning
After general anesthesia the patient is placed in either supine or lateral position (the latter is our preference). Two large, soft pillows are inserted beneath the patient’s body and leg, and one pillow is placed between the legs to prevent compression of the fibular peroneal nerve. An axillary roll is placed under the dependent axilla, and the forearm, opposite to the incision site, is wrapped with air-bead wrapping to secure the space between the head and shoulder. The head is fixed using a three-point head clamp (Mayfield). One pin should be on the front side over the hairline and two near the inion and the vertex, so that the clamp holds the head up against gravity. The neck should be bent at the end of positioning to allow for the drilling procedure. The head is rotated slightly away from the affected side, and flexed with lateral bending of about 10–15 degrees so that the digastric notch is positioned at the top. In this head position, to preserve venous return and minimize postoperative neck pain due to extensive bending, about two fingers breadth of distance must be left between the chin and sternum, as well as between the mandibular angle and clavicle. The upper shoulder is taped down and away from the operator’s field.
Scalp Incision to Craniectomy
The mastoid eminence is defined with a marker, and a line is drawn from the inion to the external auditory canal along which the transverse sinus is located. The digastric groove line will show the junction of the transverse and sigmoid sinuses. In MVD for HFS exposure of the transverse sinus is not needed, so the incision line is rather inferior to that made for trigeminal neuralgia. A linear incision line is created medial and parallel to the hairline, measuring about 60–70 mm in length. If the patient has a short, thick neck the incision line must be rather longer and medially (posteriorly) positioned, approximately slightly greater than 10 mm in distance from the hairline, to allow free movement of the operator’s hands and instruments during the microsurgery. An S-shaped incision at the end is more helpful to render a wider exposure for short- and thick-necked patients. After the scalp incision, the subcutaneous tissue is undermined and a small self-retaining retractor is applied. The fascia is incised, and the muscle layers are split using monopolar cauterization. There are two muscle layers: the sternocleidomastoid muscle and the suboccipital muscle. In most cases the anastomosis of the superficial temporal artery and occipital artery passes through the second layer of fascia and must be safely sacrificed with bipolar coagulation and then cut. After the muscles are split, a burr hole is created with a cranial perforator at the flat occipital squama containing the mastoid emissary vein, located at the superior lateral corner. The craniectomy is extended to about 20–25 mm in size using a rongeur until the sigmoid sinus is slightly exposed at its lateral (anterior) margin. During craniectomy all bleeding from bone and the opened mastoid air cell must be fully sealed with bone-wax.
Dural Incision and Exposure
A small dural incision of less than 5 mm in length is started at the caudal lateral corner under a microscope. The CSF is aspirated slowly using a small cottonoid until the cerebellum becomes more relaxed. If relaxation is not sufficient, the small dura incision is extended to expose and tear the cisterna magna or lateral cerebellomedullary cistern to drain more CSF. The dura is incised in a T-shaped fashion. The vertical line of this T-shaped incision (stem) is created curvilinearly along the sigmoid sinus and the horizontal side (arm) of the incision at the caudal medial corner. The resulting flaps are secured with tenting sutures to widen the exposure. After the dural incision, the cerebellum is elevated superiorly (cephaladly) using a tapered retractor to expose the XIth cranial nerve. With gentle retraction, all the arachnoid membranes around the lower cranial nerve are incised sharply with microscissors. A wide dissection is applied toward the IXth and Xth cranial nerves to provide sufficient surgical space and minimal tension to the VIIth and VIIIth nerves. When the choroid plexus of the lateral recess is exposed, the retractor is moved in such a way as to retract the cerebellum slightly from the lateral to the medial side, taking care not to cause mechanical traction injury to the cochlear nerve. Some small bridging veins are occasionally met and, as a policy, we do not coagulate and divide them unless these tiny veins cannot be mobilized during retraction after full dissection of the arachnoid layer.
While exposing the root exit zone of the facial nerve and the VIIth and VIIIth nerve complex, one must avoid placing the retractor deeper than the choroid plexus since the ventral cochlear nucleus and the proximal portion of the cochlear nerve are located just beneath it. Retracting this area could lead to severe hearing loss. Therefore it is necessary to pull the choroid plexus up with a retractor and then retract the flocculus and the choroid plexus.
Decompression of the Offending Vessel
Once the offending vessel is visualized, it must be carefully dissected until it is free enough to be mobilized. However, if the vertebral artery or its branches are involved, transposing them may not be easy because of their large caliber and frequently associated atherosclerotic change. Vascular compression is frequently caused by two or more vessels and completely exposing and dissecting them may be difficult, especially for those offenders with tiny perforators into the brainstem. If an offender is not found, we try to find it utilizing minimal retraction with a suction tip or micromirror behind the VII–VIIIth nerve complex.
Materials used for MVD are various, including muscle–fascial grafts, Gelfoam, Ivalon sponges, Teflon felt, artificial dura, and others. In our institution we use only Teflon, because shredded Teflon is easy to manipulate in the small field of operation and is conveniently shaped into balls of variable sizes or sheet forms. Teflon felt can also be used when performing a sling technique to transpose the offender away from the root exit zone. At the end of the decompression, a few drops of fibrin glue are placed around the Teflon felt for fixation.
There are two methods for MVD: interposition and transposition. The interposition technique is useful for almost all cases, but the transposition technique may be better for a compressing vertebral artery when decompression is not sufficient with interposition because of strong compression by or immobility of the vertebral artery.
Closure
The dura is closed in a watertight fashion via subcutaneous fascial grafting or with a small amount of muscle grafting. Recently artificial materials such as a fibrin sealant patch are frequently used to improve hemostasis and prevent CSF leakage. The craniectomy site is covered with autologous bone chips that were collected during perforating and rongeuring at opening. Muscles and fascia are sutured layer by layer.
Intraoperative Monitoring
Intraoperative facial EMG is highly useful to predict the adequacy of decompression of the affected nerve. The lateral spread response (LSR) is recorded at the other branches of the facial nerve when one branch of the facial nerve is electrically stimulated based on the ephaptic transmission theory. The sensitivity and specificity of this technique were each reported to be 0.97 ( ). Disappearance of the LSR seems to be a good index associated with early spasm relief. However, its consistency with the overall clinical outcome is still a matter of debate ( ).
The use of intraoperative brainstem auditory evoked potential monitoring has substantially reduced the risk of postoperative hearing loss ( ). Among several waves, latency and amplitude changes in waves I and V are important factors to predict postoperative auditory disturbances ( ). In particular, a decrease in amplitude by over half or a latency delay of more than 1 ms of wave V are important points that might correlate with ischemic change or stretching of the VIIIth cranial nerve, resulting in postoperative hearing deficits ( ). During the operation, if such warning signs are detected surgeons should pause the procedure and release the cerebellar retraction to allow time for recovery. The operation can resume in 10 min, in which time the waves usually recover to the original state. Loss of wave V during MVD significantly increases the odds of hearing loss, so careful manipulation is necessary to prevent changes in the waves and subsequent hearing problems ( ).
Hemifacial Spasm
Introduction
Hemifacial spasm (HFS) is a unilateral, involuntary, paroxysmal movement of facial muscles innervated by the ipsilateral facial nerve. Its characteristic features include tonic–clonic contractions and synkinesis of the muscles. These symptoms usually start from the orbicularis oculi muscle and gradually spread inferiorly to the muscles around the cheek, mouth, and neck. Eventually the facial spasm worsens in frequency and severity, resulting in sustained muscle contractions and a disfiguring grimace ( ).
In Schultze first reported the concept of vascular compression in a 56-year-old man with left HFS, revealing at autopsy a giant aneurysm from the left vertebral artery that was compressing the facial nerve root. Gowers described the classical clinical features of HFS in , and the terminology of “Hémispasme facial périphérique” was initially used by Babinski in . Gardner reported his first case of HFS treated by posterior fossa vascular decompression surgery in . In the 1970s Jannetta expanded Gardner’s neurovascular compression theory and established the microvascular decompression (MVD) procedure as a standard treatment for HFS ( ). Since then detailed surgical techniques for MVD have advanced, with the development of neuroimaging and intraoperative monitoring in the pursuit of better outcomes and fewer complications ( ).
Etiology and Pathophysiology
HFS can be divided into two types according to the cause of the disease: primary or secondary. Primary HFS is approximately four times more frequently presented than secondary HFS ( ). Primary HFS is mainly precipitated by aberrant vascular compression at the root exit zone of the seventh cranial nerve ( ). Compression of the nerve by any artery or vein can elicit clinical symptoms in the affected patients. The most common offending vessels are the posterior inferior cerebellar or anterior inferior cerebellar arteries, followed by the vertebral artery or superior cerebellar artery ( ). In contrast, secondary HFS is caused by facial nerve damage from any other cause, such as cerebellopontine angle tumor, vascular malformation, or a demyelinating condition (e.g., multiple sclerosis) ( ) ( Table 78.1 ).
Cerebellopontine angle tumors: acoustic neuroma, meningioma |
Epidermoid, arachnoid cyst, lipoma |
Arteriovenous malformation, cerebral aneurysm |
Brainstem lesions: stroke, trauma, demyelinating disorders |
Infections |
Structural abnormalities of the posterior cranial fossa: chiari malformation |
Two different hypotheses have been accepted regarding the underlying pathophysiology of HFS. The ephaptic transmission hypothesis (the peripheral hypothesis) states that close vascular contact with a portion of the “transitional zone,” 1.9–2.5 mm between the central (oligodendrocytes) and peripheral (Schwann cell) types of myelin, can cause myelin breakdown, thereby allowing abnormal neural firing in injured axons to communicate aberrantly to the adjacent nerve fibers ( ). In particular, the cranial nerves in the transitional zone are only protected by the arachnoid membrane because of a lack of epineurium, and thus are vulnerable to mechanical injury ( ). The second hypothesis, the hyperactivity hypothesis (the central hypothesis), states that abnormal hyperexcitability of the facial motor nucleus results from vascular irritation to the peripheral facial nerve as well as to the nucleus itself ( ). Recently a sympathetic hypothesis came to the fore, suggesting that neurotransmitters released from sympathetic nerve endings in the adventitia of the offending arteries induced ectopic action potentials in nerve fibers and consequent involuntary muscle contractions ( ). One new study suggests that HFS is related to the reorganization of the gray and white matter, based on viewing reduced or increased gray-matter volume in a specific cortex on voxel-based morphometry and diffusion tensor imaging ( ).
Clinical Manifestations
The prevalence of HFS is 7.4–14.5 per 100,000 persons per year ( ). Most patients are middle aged, with a mean age of onset of 44 years. HFS rarely occurs in young patients (1%–6%), although when it does it assumes a similar course and progress as in adults ( ). Females are about twice as susceptible to HFS as males ( ). The majority of HFS appears on one side of the face, and less than 5% of cases occur on both sides ( ). Generally, HFS is triggered or aggravated by emotional stress, fatigue, psychiatric tension, or anxiety ( ). Although it is still controversial, some studies suggest that arterial hypertension is associated with HFS. Hypertension contributes to the intravascular hemodynamic changes resulting in vessel ectasia, and a strong correlation between hypertension and ventrolateral medulla compression has been found ( ).
Typical HFS begins at the lower eyelid, gradually progressing to the entire oculi muscles and those in the lower face, including the orbicularis oris and platysma. Atypical HFS, a less common type, is associated with a facial contraction that spreads in the opposite direction, from the lower portion up to the frontalis muscles. Although these two entities usually show different compression sites of the facial nerve at the brainstem (typical HFS is associated with compression at the antero-caudal aspect), all types of HFS are regarded as a type of neurovascular compression syndrome and managed in the same way ( ). In severe cases paroxysmal and persisting contraction of facial muscles causes functional blindness in the affected eye, insomnia, an annoying clicking sound from involving the stapedius muscle, and eventually cranial nerve deficits such as hearing loss or facial palsy ( ). HFS usually has a progressive course, and spontaneous resolution is generally not expected ( ).
Diagnosis
Clinical Diagnosis
The diagnosis of HFS is made based on the clinical symptoms. Synchronized upper facial muscle contractions are the main feature of HFS diagnosis and are induced by a simple physical maneuver, like lifting the ipsilateral eyebrow with eye closure (the other Babinski sign) ( ). Furthermore, HFS mimics other craniofacial dyskinesias, including blepharospasm, tics, synkinesis, myokymia, craniocervical dystonia, and partial seizures ( ). Psychogenic HFS is rare, occurring in 2.4% of patients, who do not require surgical treatment ( ). It is very important to differentiate HFS from other similar diseases ( ).
- •
Blepharospasm is characterized by bilateral synchronous contractions of the muscles around the eye. It differs from HFS in being bilateral, and involves only the musculature around the eye rather than presenting with steady progression down the face, as in HFS.
- •
Tics are brief, repetitive, stereotyped, and involuntary movements. Tics are similar to the movements of habitual spasms like blinking, and are associated with more tonic components than HFS.
- •
Facial myokymia is characterized by unilateral, undulating, worm-like, continuous muscle contractions associated with intrinsic brainstem pathology, which has a distinct and diagnostic electromyographic pattern. The symptoms disappear spontaneously in most cases within a few weeks.
- •
Postparalytic synkinesis may occur after aberrant regeneration of the facial nerve following Bell’s palsy. A history of antecedent Bell’s palsy, with these movements developing on regeneration of the nerve, is helpful in differentiating this condition.
- •
Tardive dyskinesia is associated with a history of exposure to dopaminergic antagonists or other neuroleptic drugs. It usually presents as stereotypical movements of the face, especially around the lips and neck.
Neuroradiologic Evaluations
Neuroradiologic examinations are usually required to confirm the diagnosis and detect the cause of HFS. In most patients the pathogenesis of HFS is attributed to neurovascular compression of the facial nerve at the root exit zone; however, a minority of patients with HFS may have secondary causes like a space-occupying lesion or demyelination, which must be ruled out ( ). Of the various modalities, high-resolution three-dimensional (3D) T2-weighted sequences of magnetic resonance imaging (MRI), including 3D balanced steady-state free precession (SSFP) imaging such as constructive interference in the steady state (CISS) or fast imaging employing steady-state acquisition, are useful to assess the location of the neurovascular compression ( ). These are imaging methods combined with fast-gradient echo that have various advantages, such as a high signal-to-noise ratio, high spatial resolution, and less cerebrospinal fluid (CSF) flow artifact ( ). Three-dimensional time-of-flight (3D TOF) magnetic resonance angiography (MRA) can assist in evaluation of the anatomical characteristics of the vascular structures at the root exit zone ( ) ( Fig. 78.1 ), and 3D SSFP and TOF MRA have a high accuracy in detecting the offending vessels compressing the facial nerve ( ). Fusion imaging of 3D magnetic resonance cisternograms and angiograms is also useful for the preoperative assessment for MVD in patients with HFS to show the relationship between the vessel and nonvascular soft tissues clearly ( ). Three-dimensional virtual endoscopy not only provides excellent views of the neurovascular structure but also enables preoperative simulations of the surgery ( ). Recently, with the development of imaging techniques and software, 3D tubular models make it possible to understand preoperative 3D neurovascular structures at a level that nearly matches the intraoperative findings ( ).

In addition, 3D TOF MRA can describe the postoperative state of the decompression site between the vessels and the root exit zone. These findings may be helpful to guide further management in patients with persistent or recurrent HFS after MVD ( ). In our institution we routinely use 3D TOF MRA and CISS imaging for the preoperative evaluation of patients with HFS, and if needed these images are acquired to ascertain whether adequate decompression was performed in failure cases ( Fig. 78.2 ).

Additional Methods
Electromyography (EMG) can differentiate HFS from other abnormal movement disorders with a muscle or denervation origin. Electrophysiological findings such as detecting lateral spread are an important hallmark providing additional information to make a diagnosis and determine whether adequate decompression has been achieved during surgery. Preoperative audiologic examinations including pure tone audiometry and tympanometry are informative to make a decision on surgery. If a patient has a hearing impairment on the contralateral side of the HFS, risking hearing loss, the surgeon has to contemplate performing the MVD or make a vigilant preoperative plan. Detailed information is given below in the subsection on intraoperative monitoring.
Management
Medical Treatment
A number of drugs have been found to be helpful for HFS, including anticonvulsants, anticholinergics, muscle relaxants like baclofen, and haloperidol ( ). The drugs commonly have limitations in terms of their tolerance and subsequent side-effects. Botulinum neurotoxin has been indicated since the early 1980s as the treatment of choice for patients who refuse MVD and those with high surgical risks. The therapeutic effects start to show in 3–6 days after injection. A successful outcome has been reported in up to 75%–100% of cases ( ). However, the injections must be repeated every 3–6 months, resulting in a high cost for the therapy ( ). Long-term use of the botulinum neurotoxin also has the risk of muscle weakness leading to facial palsy, diplopia, or ptosis ( ).
Microvascular Decompression
MVD of the facial nerve is one of the standard treatments to alleviate neurovascular contact. It can offer a complete cure and has excellent and prolonged symptom relief, with success rates of 83%–99% ( ). Since there are a variety of operative techniques involving MVD, depending on the surgeon’s preference and the patient’s condition, we describe our own MVD techniques here. The main aspects of our techniques are sharp arachnoid dissection and minimal cerebellar retraction to achieve MVD with optimal safety and effectiveness. Neuroendoscopy can be used to provide a more accurate view of neurovascular conflicts and confirm the completeness of the decompression in cases with limited microscopic view ( ). In rare cases HFS appears with the cisternal portion of the facial nerve being indented by the offending vessels ( ). The outcomes of MVD in HFS cases with distal compression are statistically similar when compared with proximal compression ( ), so it is recommended that neurosurgeons keep in mind the possibility of the compressing vessel existing at a distal part of the facial nerve when a likely candidate is not detected at the conventional root exit zone on preoperative evaluation.
Operative Technique
Patient Positioning
After general anesthesia the patient is placed in either supine or lateral position (the latter is our preference). Two large, soft pillows are inserted beneath the patient’s body and leg, and one pillow is placed between the legs to prevent compression of the fibular peroneal nerve. An axillary roll is placed under the dependent axilla, and the forearm, opposite to the incision site, is wrapped with air-bead wrapping to secure the space between the head and shoulder. The head is fixed using a three-point head clamp (Mayfield). One pin should be on the front side over the hairline and two near the inion and the vertex, so that the clamp holds the head up against gravity. The neck should be bent at the end of positioning to allow for the drilling procedure. The head is rotated slightly away from the affected side, and flexed with lateral bending of about 10–15 degrees so that the digastric notch is positioned at the top. In this head position, to preserve venous return and minimize postoperative neck pain due to extensive bending, about two fingers breadth of distance must be left between the chin and sternum, as well as between the mandibular angle and clavicle. The upper shoulder is taped down and away from the operator’s field.
Scalp Incision to Craniectomy
The mastoid eminence is defined with a marker, and a line is drawn from the inion to the external auditory canal along which the transverse sinus is located. The digastric groove line will show the junction of the transverse and sigmoid sinuses. In MVD for HFS exposure of the transverse sinus is not needed, so the incision line is rather inferior to that made for trigeminal neuralgia. A linear incision line is created medial and parallel to the hairline, measuring about 60–70 mm in length. If the patient has a short, thick neck the incision line must be rather longer and medially (posteriorly) positioned, approximately slightly greater than 10 mm in distance from the hairline, to allow free movement of the operator’s hands and instruments during the microsurgery. An S-shaped incision at the end is more helpful to render a wider exposure for short- and thick-necked patients. After the scalp incision, the subcutaneous tissue is undermined and a small self-retaining retractor is applied. The fascia is incised, and the muscle layers are split using monopolar cauterization. There are two muscle layers: the sternocleidomastoid muscle and the suboccipital muscle. In most cases the anastomosis of the superficial temporal artery and occipital artery passes through the second layer of fascia and must be safely sacrificed with bipolar coagulation and then cut. After the muscles are split, a burr hole is created with a cranial perforator at the flat occipital squama containing the mastoid emissary vein, located at the superior lateral corner. The craniectomy is extended to about 20–25 mm in size using a rongeur until the sigmoid sinus is slightly exposed at its lateral (anterior) margin. During craniectomy all bleeding from bone and the opened mastoid air cell must be fully sealed with bone-wax.
Dural Incision and Exposure
A small dural incision of less than 5 mm in length is started at the caudal lateral corner under a microscope. The CSF is aspirated slowly using a small cottonoid until the cerebellum becomes more relaxed. If relaxation is not sufficient, the small dura incision is extended to expose and tear the cisterna magna or lateral cerebellomedullary cistern to drain more CSF. The dura is incised in a T-shaped fashion. The vertical line of this T-shaped incision (stem) is created curvilinearly along the sigmoid sinus and the horizontal side (arm) of the incision at the caudal medial corner. The resulting flaps are secured with tenting sutures to widen the exposure. After the dural incision, the cerebellum is elevated superiorly (cephaladly) using a tapered retractor to expose the XIth cranial nerve. With gentle retraction, all the arachnoid membranes around the lower cranial nerve are incised sharply with microscissors. A wide dissection is applied toward the IXth and Xth cranial nerves to provide sufficient surgical space and minimal tension to the VIIth and VIIIth nerves. When the choroid plexus of the lateral recess is exposed, the retractor is moved in such a way as to retract the cerebellum slightly from the lateral to the medial side, taking care not to cause mechanical traction injury to the cochlear nerve. Some small bridging veins are occasionally met and, as a policy, we do not coagulate and divide them unless these tiny veins cannot be mobilized during retraction after full dissection of the arachnoid layer.
While exposing the root exit zone of the facial nerve and the VIIth and VIIIth nerve complex, one must avoid placing the retractor deeper than the choroid plexus since the ventral cochlear nucleus and the proximal portion of the cochlear nerve are located just beneath it. Retracting this area could lead to severe hearing loss. Therefore it is necessary to pull the choroid plexus up with a retractor and then retract the flocculus and the choroid plexus.
Decompression of the Offending Vessel
Once the offending vessel is visualized, it must be carefully dissected until it is free enough to be mobilized. However, if the vertebral artery or its branches are involved, transposing them may not be easy because of their large caliber and frequently associated atherosclerotic change. Vascular compression is frequently caused by two or more vessels and completely exposing and dissecting them may be difficult, especially for those offenders with tiny perforators into the brainstem. If an offender is not found, we try to find it utilizing minimal retraction with a suction tip or micromirror behind the VII–VIIIth nerve complex.
Materials used for MVD are various, including muscle–fascial grafts, Gelfoam, Ivalon sponges, Teflon felt, artificial dura, and others. In our institution we use only Teflon, because shredded Teflon is easy to manipulate in the small field of operation and is conveniently shaped into balls of variable sizes or sheet forms. Teflon felt can also be used when performing a sling technique to transpose the offender away from the root exit zone. At the end of the decompression, a few drops of fibrin glue are placed around the Teflon felt for fixation.
There are two methods for MVD: interposition and transposition. The interposition technique is useful for almost all cases, but the transposition technique may be better for a compressing vertebral artery when decompression is not sufficient with interposition because of strong compression by or immobility of the vertebral artery.
Closure
The dura is closed in a watertight fashion via subcutaneous fascial grafting or with a small amount of muscle grafting. Recently artificial materials such as a fibrin sealant patch are frequently used to improve hemostasis and prevent CSF leakage. The craniectomy site is covered with autologous bone chips that were collected during perforating and rongeuring at opening. Muscles and fascia are sutured layer by layer.
Intraoperative Monitoring
Intraoperative facial EMG is highly useful to predict the adequacy of decompression of the affected nerve. The lateral spread response (LSR) is recorded at the other branches of the facial nerve when one branch of the facial nerve is electrically stimulated based on the ephaptic transmission theory. The sensitivity and specificity of this technique were each reported to be 0.97 ( ). Disappearance of the LSR seems to be a good index associated with early spasm relief. However, its consistency with the overall clinical outcome is still a matter of debate ( ).
The use of intraoperative brainstem auditory evoked potential monitoring has substantially reduced the risk of postoperative hearing loss ( ). Among several waves, latency and amplitude changes in waves I and V are important factors to predict postoperative auditory disturbances ( ). In particular, a decrease in amplitude by over half or a latency delay of more than 1 ms of wave V are important points that might correlate with ischemic change or stretching of the VIIIth cranial nerve, resulting in postoperative hearing deficits ( ). During the operation, if such warning signs are detected surgeons should pause the procedure and release the cerebellar retraction to allow time for recovery. The operation can resume in 10 min, in which time the waves usually recover to the original state. Loss of wave V during MVD significantly increases the odds of hearing loss, so careful manipulation is necessary to prevent changes in the waves and subsequent hearing problems ( ).
Hemifacial Spasm
Introduction
Hemifacial spasm (HFS) is a unilateral, involuntary, paroxysmal movement of facial muscles innervated by the ipsilateral facial nerve. Its characteristic features include tonic–clonic contractions and synkinesis of the muscles. These symptoms usually start from the orbicularis oculi muscle and gradually spread inferiorly to the muscles around the cheek, mouth, and neck. Eventually the facial spasm worsens in frequency and severity, resulting in sustained muscle contractions and a disfiguring grimace ( ).
In Schultze first reported the concept of vascular compression in a 56-year-old man with left HFS, revealing at autopsy a giant aneurysm from the left vertebral artery that was compressing the facial nerve root. Gowers described the classical clinical features of HFS in , and the terminology of “Hémispasme facial périphérique” was initially used by Babinski in . Gardner reported his first case of HFS treated by posterior fossa vascular decompression surgery in . In the 1970s Jannetta expanded Gardner’s neurovascular compression theory and established the microvascular decompression (MVD) procedure as a standard treatment for HFS ( ). Since then detailed surgical techniques for MVD have advanced, with the development of neuroimaging and intraoperative monitoring in the pursuit of better outcomes and fewer complications ( ).
Etiology and Pathophysiology
HFS can be divided into two types according to the cause of the disease: primary or secondary. Primary HFS is approximately four times more frequently presented than secondary HFS ( ). Primary HFS is mainly precipitated by aberrant vascular compression at the root exit zone of the seventh cranial nerve ( ). Compression of the nerve by any artery or vein can elicit clinical symptoms in the affected patients. The most common offending vessels are the posterior inferior cerebellar or anterior inferior cerebellar arteries, followed by the vertebral artery or superior cerebellar artery ( ). In contrast, secondary HFS is caused by facial nerve damage from any other cause, such as cerebellopontine angle tumor, vascular malformation, or a demyelinating condition (e.g., multiple sclerosis) ( ) ( Table 78.1 ).
Cerebellopontine angle tumors: acoustic neuroma, meningioma |
Epidermoid, arachnoid cyst, lipoma |
Arteriovenous malformation, cerebral aneurysm |
Brainstem lesions: stroke, trauma, demyelinating disorders |
Infections |
Structural abnormalities of the posterior cranial fossa: chiari malformation |
Two different hypotheses have been accepted regarding the underlying pathophysiology of HFS. The ephaptic transmission hypothesis (the peripheral hypothesis) states that close vascular contact with a portion of the “transitional zone,” 1.9–2.5 mm between the central (oligodendrocytes) and peripheral (Schwann cell) types of myelin, can cause myelin breakdown, thereby allowing abnormal neural firing in injured axons to communicate aberrantly to the adjacent nerve fibers ( ). In particular, the cranial nerves in the transitional zone are only protected by the arachnoid membrane because of a lack of epineurium, and thus are vulnerable to mechanical injury ( ). The second hypothesis, the hyperactivity hypothesis (the central hypothesis), states that abnormal hyperexcitability of the facial motor nucleus results from vascular irritation to the peripheral facial nerve as well as to the nucleus itself ( ). Recently a sympathetic hypothesis came to the fore, suggesting that neurotransmitters released from sympathetic nerve endings in the adventitia of the offending arteries induced ectopic action potentials in nerve fibers and consequent involuntary muscle contractions ( ). One new study suggests that HFS is related to the reorganization of the gray and white matter, based on viewing reduced or increased gray-matter volume in a specific cortex on voxel-based morphometry and diffusion tensor imaging ( ).
Clinical Manifestations
The prevalence of HFS is 7.4–14.5 per 100,000 persons per year ( ). Most patients are middle aged, with a mean age of onset of 44 years. HFS rarely occurs in young patients (1%–6%), although when it does it assumes a similar course and progress as in adults ( ). Females are about twice as susceptible to HFS as males ( ). The majority of HFS appears on one side of the face, and less than 5% of cases occur on both sides ( ). Generally, HFS is triggered or aggravated by emotional stress, fatigue, psychiatric tension, or anxiety ( ). Although it is still controversial, some studies suggest that arterial hypertension is associated with HFS. Hypertension contributes to the intravascular hemodynamic changes resulting in vessel ectasia, and a strong correlation between hypertension and ventrolateral medulla compression has been found ( ).
Typical HFS begins at the lower eyelid, gradually progressing to the entire oculi muscles and those in the lower face, including the orbicularis oris and platysma. Atypical HFS, a less common type, is associated with a facial contraction that spreads in the opposite direction, from the lower portion up to the frontalis muscles. Although these two entities usually show different compression sites of the facial nerve at the brainstem (typical HFS is associated with compression at the antero-caudal aspect), all types of HFS are regarded as a type of neurovascular compression syndrome and managed in the same way ( ). In severe cases paroxysmal and persisting contraction of facial muscles causes functional blindness in the affected eye, insomnia, an annoying clicking sound from involving the stapedius muscle, and eventually cranial nerve deficits such as hearing loss or facial palsy ( ). HFS usually has a progressive course, and spontaneous resolution is generally not expected ( ).
Diagnosis
Clinical Diagnosis
The diagnosis of HFS is made based on the clinical symptoms. Synchronized upper facial muscle contractions are the main feature of HFS diagnosis and are induced by a simple physical maneuver, like lifting the ipsilateral eyebrow with eye closure (the other Babinski sign) ( ). Furthermore, HFS mimics other craniofacial dyskinesias, including blepharospasm, tics, synkinesis, myokymia, craniocervical dystonia, and partial seizures ( ). Psychogenic HFS is rare, occurring in 2.4% of patients, who do not require surgical treatment ( ). It is very important to differentiate HFS from other similar diseases ( ).
- •
Blepharospasm is characterized by bilateral synchronous contractions of the muscles around the eye. It differs from HFS in being bilateral, and involves only the musculature around the eye rather than presenting with steady progression down the face, as in HFS.
- •
Tics are brief, repetitive, stereotyped, and involuntary movements. Tics are similar to the movements of habitual spasms like blinking, and are associated with more tonic components than HFS.
- •
Facial myokymia is characterized by unilateral, undulating, worm-like, continuous muscle contractions associated with intrinsic brainstem pathology, which has a distinct and diagnostic electromyographic pattern. The symptoms disappear spontaneously in most cases within a few weeks.
- •
Postparalytic synkinesis may occur after aberrant regeneration of the facial nerve following Bell’s palsy. A history of antecedent Bell’s palsy, with these movements developing on regeneration of the nerve, is helpful in differentiating this condition.
- •
Tardive dyskinesia is associated with a history of exposure to dopaminergic antagonists or other neuroleptic drugs. It usually presents as stereotypical movements of the face, especially around the lips and neck.
Neuroradiologic Evaluations
Neuroradiologic examinations are usually required to confirm the diagnosis and detect the cause of HFS. In most patients the pathogenesis of HFS is attributed to neurovascular compression of the facial nerve at the root exit zone; however, a minority of patients with HFS may have secondary causes like a space-occupying lesion or demyelination, which must be ruled out ( ). Of the various modalities, high-resolution three-dimensional (3D) T2-weighted sequences of magnetic resonance imaging (MRI), including 3D balanced steady-state free precession (SSFP) imaging such as constructive interference in the steady state (CISS) or fast imaging employing steady-state acquisition, are useful to assess the location of the neurovascular compression ( ). These are imaging methods combined with fast-gradient echo that have various advantages, such as a high signal-to-noise ratio, high spatial resolution, and less cerebrospinal fluid (CSF) flow artifact ( ). Three-dimensional time-of-flight (3D TOF) magnetic resonance angiography (MRA) can assist in evaluation of the anatomical characteristics of the vascular structures at the root exit zone ( ) ( Fig. 78.1 ), and 3D SSFP and TOF MRA have a high accuracy in detecting the offending vessels compressing the facial nerve ( ). Fusion imaging of 3D magnetic resonance cisternograms and angiograms is also useful for the preoperative assessment for MVD in patients with HFS to show the relationship between the vessel and nonvascular soft tissues clearly ( ). Three-dimensional virtual endoscopy not only provides excellent views of the neurovascular structure but also enables preoperative simulations of the surgery ( ). Recently, with the development of imaging techniques and software, 3D tubular models make it possible to understand preoperative 3D neurovascular structures at a level that nearly matches the intraoperative findings ( ).

In addition, 3D TOF MRA can describe the postoperative state of the decompression site between the vessels and the root exit zone. These findings may be helpful to guide further management in patients with persistent or recurrent HFS after MVD ( ). In our institution we routinely use 3D TOF MRA and CISS imaging for the preoperative evaluation of patients with HFS, and if needed these images are acquired to ascertain whether adequate decompression was performed in failure cases ( Fig. 78.2 ).

Additional Methods
Electromyography (EMG) can differentiate HFS from other abnormal movement disorders with a muscle or denervation origin. Electrophysiological findings such as detecting lateral spread are an important hallmark providing additional information to make a diagnosis and determine whether adequate decompression has been achieved during surgery. Preoperative audiologic examinations including pure tone audiometry and tympanometry are informative to make a decision on surgery. If a patient has a hearing impairment on the contralateral side of the HFS, risking hearing loss, the surgeon has to contemplate performing the MVD or make a vigilant preoperative plan. Detailed information is given below in the subsection on intraoperative monitoring.
Management
Medical Treatment
A number of drugs have been found to be helpful for HFS, including anticonvulsants, anticholinergics, muscle relaxants like baclofen, and haloperidol ( ). The drugs commonly have limitations in terms of their tolerance and subsequent side-effects. Botulinum neurotoxin has been indicated since the early 1980s as the treatment of choice for patients who refuse MVD and those with high surgical risks. The therapeutic effects start to show in 3–6 days after injection. A successful outcome has been reported in up to 75%–100% of cases ( ). However, the injections must be repeated every 3–6 months, resulting in a high cost for the therapy ( ). Long-term use of the botulinum neurotoxin also has the risk of muscle weakness leading to facial palsy, diplopia, or ptosis ( ).
Microvascular Decompression
MVD of the facial nerve is one of the standard treatments to alleviate neurovascular contact. It can offer a complete cure and has excellent and prolonged symptom relief, with success rates of 83%–99% ( ). Since there are a variety of operative techniques involving MVD, depending on the surgeon’s preference and the patient’s condition, we describe our own MVD techniques here. The main aspects of our techniques are sharp arachnoid dissection and minimal cerebellar retraction to achieve MVD with optimal safety and effectiveness. Neuroendoscopy can be used to provide a more accurate view of neurovascular conflicts and confirm the completeness of the decompression in cases with limited microscopic view ( ). In rare cases HFS appears with the cisternal portion of the facial nerve being indented by the offending vessels ( ). The outcomes of MVD in HFS cases with distal compression are statistically similar when compared with proximal compression ( ), so it is recommended that neurosurgeons keep in mind the possibility of the compressing vessel existing at a distal part of the facial nerve when a likely candidate is not detected at the conventional root exit zone on preoperative evaluation.
Operative Technique
Patient Positioning
After general anesthesia the patient is placed in either supine or lateral position (the latter is our preference). Two large, soft pillows are inserted beneath the patient’s body and leg, and one pillow is placed between the legs to prevent compression of the fibular peroneal nerve. An axillary roll is placed under the dependent axilla, and the forearm, opposite to the incision site, is wrapped with air-bead wrapping to secure the space between the head and shoulder. The head is fixed using a three-point head clamp (Mayfield). One pin should be on the front side over the hairline and two near the inion and the vertex, so that the clamp holds the head up against gravity. The neck should be bent at the end of positioning to allow for the drilling procedure. The head is rotated slightly away from the affected side, and flexed with lateral bending of about 10–15 degrees so that the digastric notch is positioned at the top. In this head position, to preserve venous return and minimize postoperative neck pain due to extensive bending, about two fingers breadth of distance must be left between the chin and sternum, as well as between the mandibular angle and clavicle. The upper shoulder is taped down and away from the operator’s field.
Scalp Incision to Craniectomy
The mastoid eminence is defined with a marker, and a line is drawn from the inion to the external auditory canal along which the transverse sinus is located. The digastric groove line will show the junction of the transverse and sigmoid sinuses. In MVD for HFS exposure of the transverse sinus is not needed, so the incision line is rather inferior to that made for trigeminal neuralgia. A linear incision line is created medial and parallel to the hairline, measuring about 60–70 mm in length. If the patient has a short, thick neck the incision line must be rather longer and medially (posteriorly) positioned, approximately slightly greater than 10 mm in distance from the hairline, to allow free movement of the operator’s hands and instruments during the microsurgery. An S-shaped incision at the end is more helpful to render a wider exposure for short- and thick-necked patients. After the scalp incision, the subcutaneous tissue is undermined and a small self-retaining retractor is applied. The fascia is incised, and the muscle layers are split using monopolar cauterization. There are two muscle layers: the sternocleidomastoid muscle and the suboccipital muscle. In most cases the anastomosis of the superficial temporal artery and occipital artery passes through the second layer of fascia and must be safely sacrificed with bipolar coagulation and then cut. After the muscles are split, a burr hole is created with a cranial perforator at the flat occipital squama containing the mastoid emissary vein, located at the superior lateral corner. The craniectomy is extended to about 20–25 mm in size using a rongeur until the sigmoid sinus is slightly exposed at its lateral (anterior) margin. During craniectomy all bleeding from bone and the opened mastoid air cell must be fully sealed with bone-wax.
Dural Incision and Exposure
A small dural incision of less than 5 mm in length is started at the caudal lateral corner under a microscope. The CSF is aspirated slowly using a small cottonoid until the cerebellum becomes more relaxed. If relaxation is not sufficient, the small dura incision is extended to expose and tear the cisterna magna or lateral cerebellomedullary cistern to drain more CSF. The dura is incised in a T-shaped fashion. The vertical line of this T-shaped incision (stem) is created curvilinearly along the sigmoid sinus and the horizontal side (arm) of the incision at the caudal medial corner. The resulting flaps are secured with tenting sutures to widen the exposure. After the dural incision, the cerebellum is elevated superiorly (cephaladly) using a tapered retractor to expose the XIth cranial nerve. With gentle retraction, all the arachnoid membranes around the lower cranial nerve are incised sharply with microscissors. A wide dissection is applied toward the IXth and Xth cranial nerves to provide sufficient surgical space and minimal tension to the VIIth and VIIIth nerves. When the choroid plexus of the lateral recess is exposed, the retractor is moved in such a way as to retract the cerebellum slightly from the lateral to the medial side, taking care not to cause mechanical traction injury to the cochlear nerve. Some small bridging veins are occasionally met and, as a policy, we do not coagulate and divide them unless these tiny veins cannot be mobilized during retraction after full dissection of the arachnoid layer.
While exposing the root exit zone of the facial nerve and the VIIth and VIIIth nerve complex, one must avoid placing the retractor deeper than the choroid plexus since the ventral cochlear nucleus and the proximal portion of the cochlear nerve are located just beneath it. Retracting this area could lead to severe hearing loss. Therefore it is necessary to pull the choroid plexus up with a retractor and then retract the flocculus and the choroid plexus.
Decompression of the Offending Vessel
Once the offending vessel is visualized, it must be carefully dissected until it is free enough to be mobilized. However, if the vertebral artery or its branches are involved, transposing them may not be easy because of their large caliber and frequently associated atherosclerotic change. Vascular compression is frequently caused by two or more vessels and completely exposing and dissecting them may be difficult, especially for those offenders with tiny perforators into the brainstem. If an offender is not found, we try to find it utilizing minimal retraction with a suction tip or micromirror behind the VII–VIIIth nerve complex.
Materials used for MVD are various, including muscle–fascial grafts, Gelfoam, Ivalon sponges, Teflon felt, artificial dura, and others. In our institution we use only Teflon, because shredded Teflon is easy to manipulate in the small field of operation and is conveniently shaped into balls of variable sizes or sheet forms. Teflon felt can also be used when performing a sling technique to transpose the offender away from the root exit zone. At the end of the decompression, a few drops of fibrin glue are placed around the Teflon felt for fixation.
There are two methods for MVD: interposition and transposition. The interposition technique is useful for almost all cases, but the transposition technique may be better for a compressing vertebral artery when decompression is not sufficient with interposition because of strong compression by or immobility of the vertebral artery.
Closure
The dura is closed in a watertight fashion via subcutaneous fascial grafting or with a small amount of muscle grafting. Recently artificial materials such as a fibrin sealant patch are frequently used to improve hemostasis and prevent CSF leakage. The craniectomy site is covered with autologous bone chips that were collected during perforating and rongeuring at opening. Muscles and fascia are sutured layer by layer.
Intraoperative Monitoring
Intraoperative facial EMG is highly useful to predict the adequacy of decompression of the affected nerve. The lateral spread response (LSR) is recorded at the other branches of the facial nerve when one branch of the facial nerve is electrically stimulated based on the ephaptic transmission theory. The sensitivity and specificity of this technique were each reported to be 0.97 ( ). Disappearance of the LSR seems to be a good index associated with early spasm relief. However, its consistency with the overall clinical outcome is still a matter of debate ( ).
The use of intraoperative brainstem auditory evoked potential monitoring has substantially reduced the risk of postoperative hearing loss ( ). Among several waves, latency and amplitude changes in waves I and V are important factors to predict postoperative auditory disturbances ( ). In particular, a decrease in amplitude by over half or a latency delay of more than 1 ms of wave V are important points that might correlate with ischemic change or stretching of the VIIIth cranial nerve, resulting in postoperative hearing deficits ( ). During the operation, if such warning signs are detected surgeons should pause the procedure and release the cerebellar retraction to allow time for recovery. The operation can resume in 10 min, in which time the waves usually recover to the original state. Loss of wave V during MVD significantly increases the odds of hearing loss, so careful manipulation is necessary to prevent changes in the waves and subsequent hearing problems ( ).
Hemifacial Spasm
Introduction
Hemifacial spasm (HFS) is a unilateral, involuntary, paroxysmal movement of facial muscles innervated by the ipsilateral facial nerve. Its characteristic features include tonic–clonic contractions and synkinesis of the muscles. These symptoms usually start from the orbicularis oculi muscle and gradually spread inferiorly to the muscles around the cheek, mouth, and neck. Eventually the facial spasm worsens in frequency and severity, resulting in sustained muscle contractions and a disfiguring grimace ( ).
In Schultze first reported the concept of vascular compression in a 56-year-old man with left HFS, revealing at autopsy a giant aneurysm from the left vertebral artery that was compressing the facial nerve root. Gowers described the classical clinical features of HFS in , and the terminology of “Hémispasme facial périphérique” was initially used by Babinski in . Gardner reported his first case of HFS treated by posterior fossa vascular decompression surgery in . In the 1970s Jannetta expanded Gardner’s neurovascular compression theory and established the microvascular decompression (MVD) procedure as a standard treatment for HFS ( ). Since then detailed surgical techniques for MVD have advanced, with the development of neuroimaging and intraoperative monitoring in the pursuit of better outcomes and fewer complications ( ).
Etiology and Pathophysiology
HFS can be divided into two types according to the cause of the disease: primary or secondary. Primary HFS is approximately four times more frequently presented than secondary HFS ( ). Primary HFS is mainly precipitated by aberrant vascular compression at the root exit zone of the seventh cranial nerve ( ). Compression of the nerve by any artery or vein can elicit clinical symptoms in the affected patients. The most common offending vessels are the posterior inferior cerebellar or anterior inferior cerebellar arteries, followed by the vertebral artery or superior cerebellar artery ( ). In contrast, secondary HFS is caused by facial nerve damage from any other cause, such as cerebellopontine angle tumor, vascular malformation, or a demyelinating condition (e.g., multiple sclerosis) ( ) ( Table 78.1 ).
Cerebellopontine angle tumors: acoustic neuroma, meningioma |
Epidermoid, arachnoid cyst, lipoma |
Arteriovenous malformation, cerebral aneurysm |
Brainstem lesions: stroke, trauma, demyelinating disorders |
Infections |
Structural abnormalities of the posterior cranial fossa: chiari malformation |
Two different hypotheses have been accepted regarding the underlying pathophysiology of HFS. The ephaptic transmission hypothesis (the peripheral hypothesis) states that close vascular contact with a portion of the “transitional zone,” 1.9–2.5 mm between the central (oligodendrocytes) and peripheral (Schwann cell) types of myelin, can cause myelin breakdown, thereby allowing abnormal neural firing in injured axons to communicate aberrantly to the adjacent nerve fibers ( ). In particular, the cranial nerves in the transitional zone are only protected by the arachnoid membrane because of a lack of epineurium, and thus are vulnerable to mechanical injury ( ). The second hypothesis, the hyperactivity hypothesis (the central hypothesis), states that abnormal hyperexcitability of the facial motor nucleus results from vascular irritation to the peripheral facial nerve as well as to the nucleus itself ( ). Recently a sympathetic hypothesis came to the fore, suggesting that neurotransmitters released from sympathetic nerve endings in the adventitia of the offending arteries induced ectopic action potentials in nerve fibers and consequent involuntary muscle contractions ( ). One new study suggests that HFS is related to the reorganization of the gray and white matter, based on viewing reduced or increased gray-matter volume in a specific cortex on voxel-based morphometry and diffusion tensor imaging ( ).
Clinical Manifestations
The prevalence of HFS is 7.4–14.5 per 100,000 persons per year ( ). Most patients are middle aged, with a mean age of onset of 44 years. HFS rarely occurs in young patients (1%–6%), although when it does it assumes a similar course and progress as in adults ( ). Females are about twice as susceptible to HFS as males ( ). The majority of HFS appears on one side of the face, and less than 5% of cases occur on both sides ( ). Generally, HFS is triggered or aggravated by emotional stress, fatigue, psychiatric tension, or anxiety ( ). Although it is still controversial, some studies suggest that arterial hypertension is associated with HFS. Hypertension contributes to the intravascular hemodynamic changes resulting in vessel ectasia, and a strong correlation between hypertension and ventrolateral medulla compression has been found ( ).
Typical HFS begins at the lower eyelid, gradually progressing to the entire oculi muscles and those in the lower face, including the orbicularis oris and platysma. Atypical HFS, a less common type, is associated with a facial contraction that spreads in the opposite direction, from the lower portion up to the frontalis muscles. Although these two entities usually show different compression sites of the facial nerve at the brainstem (typical HFS is associated with compression at the antero-caudal aspect), all types of HFS are regarded as a type of neurovascular compression syndrome and managed in the same way ( ). In severe cases paroxysmal and persisting contraction of facial muscles causes functional blindness in the affected eye, insomnia, an annoying clicking sound from involving the stapedius muscle, and eventually cranial nerve deficits such as hearing loss or facial palsy ( ). HFS usually has a progressive course, and spontaneous resolution is generally not expected ( ).
Diagnosis
Clinical Diagnosis
The diagnosis of HFS is made based on the clinical symptoms. Synchronized upper facial muscle contractions are the main feature of HFS diagnosis and are induced by a simple physical maneuver, like lifting the ipsilateral eyebrow with eye closure (the other Babinski sign) ( ). Furthermore, HFS mimics other craniofacial dyskinesias, including blepharospasm, tics, synkinesis, myokymia, craniocervical dystonia, and partial seizures ( ). Psychogenic HFS is rare, occurring in 2.4% of patients, who do not require surgical treatment ( ). It is very important to differentiate HFS from other similar diseases ( ).
- •
Blepharospasm is characterized by bilateral synchronous contractions of the muscles around the eye. It differs from HFS in being bilateral, and involves only the musculature around the eye rather than presenting with steady progression down the face, as in HFS.
- •
Tics are brief, repetitive, stereotyped, and involuntary movements. Tics are similar to the movements of habitual spasms like blinking, and are associated with more tonic components than HFS.
- •
Facial myokymia is characterized by unilateral, undulating, worm-like, continuous muscle contractions associated with intrinsic brainstem pathology, which has a distinct and diagnostic electromyographic pattern. The symptoms disappear spontaneously in most cases within a few weeks.
- •
Postparalytic synkinesis may occur after aberrant regeneration of the facial nerve following Bell’s palsy. A history of antecedent Bell’s palsy, with these movements developing on regeneration of the nerve, is helpful in differentiating this condition.
- •
Tardive dyskinesia is associated with a history of exposure to dopaminergic antagonists or other neuroleptic drugs. It usually presents as stereotypical movements of the face, especially around the lips and neck.
Neuroradiologic Evaluations
Neuroradiologic examinations are usually required to confirm the diagnosis and detect the cause of HFS. In most patients the pathogenesis of HFS is attributed to neurovascular compression of the facial nerve at the root exit zone; however, a minority of patients with HFS may have secondary causes like a space-occupying lesion or demyelination, which must be ruled out ( ). Of the various modalities, high-resolution three-dimensional (3D) T2-weighted sequences of magnetic resonance imaging (MRI), including 3D balanced steady-state free precession (SSFP) imaging such as constructive interference in the steady state (CISS) or fast imaging employing steady-state acquisition, are useful to assess the location of the neurovascular compression ( ). These are imaging methods combined with fast-gradient echo that have various advantages, such as a high signal-to-noise ratio, high spatial resolution, and less cerebrospinal fluid (CSF) flow artifact ( ). Three-dimensional time-of-flight (3D TOF) magnetic resonance angiography (MRA) can assist in evaluation of the anatomical characteristics of the vascular structures at the root exit zone ( ) ( Fig. 78.1 ), and 3D SSFP and TOF MRA have a high accuracy in detecting the offending vessels compressing the facial nerve ( ). Fusion imaging of 3D magnetic resonance cisternograms and angiograms is also useful for the preoperative assessment for MVD in patients with HFS to show the relationship between the vessel and nonvascular soft tissues clearly ( ). Three-dimensional virtual endoscopy not only provides excellent views of the neurovascular structure but also enables preoperative simulations of the surgery ( ). Recently, with the development of imaging techniques and software, 3D tubular models make it possible to understand preoperative 3D neurovascular structures at a level that nearly matches the intraoperative findings ( ).

In addition, 3D TOF MRA can describe the postoperative state of the decompression site between the vessels and the root exit zone. These findings may be helpful to guide further management in patients with persistent or recurrent HFS after MVD ( ). In our institution we routinely use 3D TOF MRA and CISS imaging for the preoperative evaluation of patients with HFS, and if needed these images are acquired to ascertain whether adequate decompression was performed in failure cases ( Fig. 78.2 ).

Additional Methods
Electromyography (EMG) can differentiate HFS from other abnormal movement disorders with a muscle or denervation origin. Electrophysiological findings such as detecting lateral spread are an important hallmark providing additional information to make a diagnosis and determine whether adequate decompression has been achieved during surgery. Preoperative audiologic examinations including pure tone audiometry and tympanometry are informative to make a decision on surgery. If a patient has a hearing impairment on the contralateral side of the HFS, risking hearing loss, the surgeon has to contemplate performing the MVD or make a vigilant preoperative plan. Detailed information is given below in the subsection on intraoperative monitoring.
Management
Medical Treatment
A number of drugs have been found to be helpful for HFS, including anticonvulsants, anticholinergics, muscle relaxants like baclofen, and haloperidol ( ). The drugs commonly have limitations in terms of their tolerance and subsequent side-effects. Botulinum neurotoxin has been indicated since the early 1980s as the treatment of choice for patients who refuse MVD and those with high surgical risks. The therapeutic effects start to show in 3–6 days after injection. A successful outcome has been reported in up to 75%–100% of cases ( ). However, the injections must be repeated every 3–6 months, resulting in a high cost for the therapy ( ). Long-term use of the botulinum neurotoxin also has the risk of muscle weakness leading to facial palsy, diplopia, or ptosis ( ).
Microvascular Decompression
MVD of the facial nerve is one of the standard treatments to alleviate neurovascular contact. It can offer a complete cure and has excellent and prolonged symptom relief, with success rates of 83%–99% ( ). Since there are a variety of operative techniques involving MVD, depending on the surgeon’s preference and the patient’s condition, we describe our own MVD techniques here. The main aspects of our techniques are sharp arachnoid dissection and minimal cerebellar retraction to achieve MVD with optimal safety and effectiveness. Neuroendoscopy can be used to provide a more accurate view of neurovascular conflicts and confirm the completeness of the decompression in cases with limited microscopic view ( ). In rare cases HFS appears with the cisternal portion of the facial nerve being indented by the offending vessels ( ). The outcomes of MVD in HFS cases with distal compression are statistically similar when compared with proximal compression ( ), so it is recommended that neurosurgeons keep in mind the possibility of the compressing vessel existing at a distal part of the facial nerve when a likely candidate is not detected at the conventional root exit zone on preoperative evaluation.
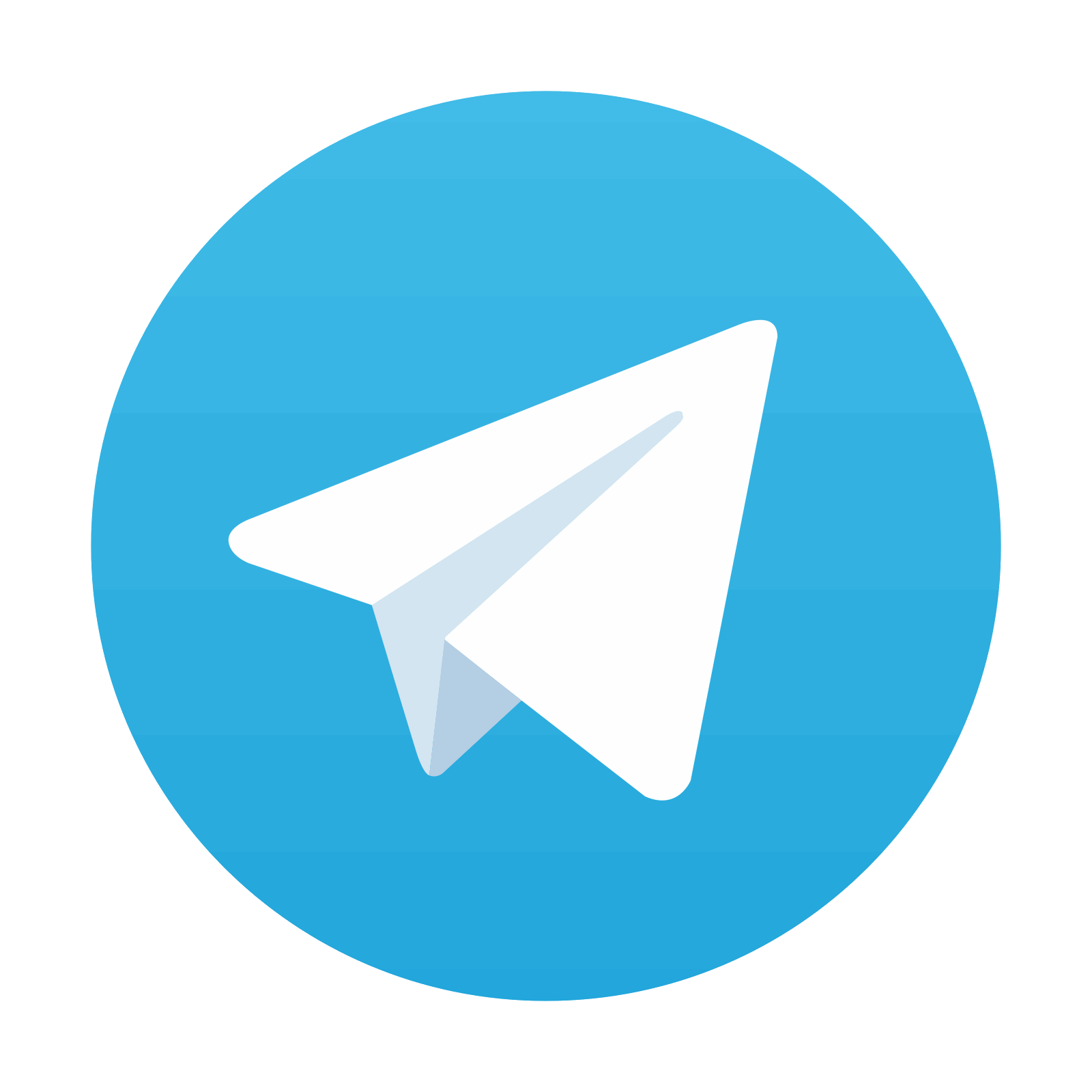
Stay updated, free articles. Join our Telegram channel

Full access? Get Clinical Tree
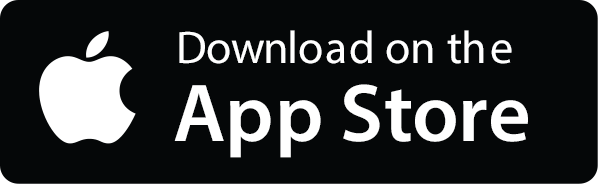
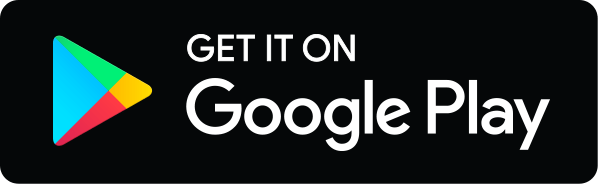